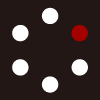
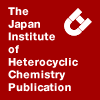
HETEROCYCLES
An International Journal for Reviews and Communications in Heterocyclic ChemistryWeb Edition ISSN: 1881-0942
Published online by The Japan Institute of Heterocyclic Chemistry
e-Journal
Full Text HTML
Received, 26th November, 2011, Accepted, 27th January, 2012, Published online, 7th February, 2012.
DOI: 10.3987/REV-11-724
■ Reaction of Arynes with Carbon-Heteroatom Double Bonds
Kentaro Okuma*
Department of Chemistry, Faculty of Sciences, Fukuoka University, 8-19-1 Nanakuma, Jonan-ku, Fukuoka 814-0180, Japan
Abstract
Recent achievements in the construction of heterocycles by the reaction of arynes with carbon-heteroatom double bonds are reviewed. Use of the Diels-Alder type, ionic, and radical mechanisms resulted in the formation of novel heterocycles containing oxygen, nitrogen, sulfur, and selenium atoms.CONTENTS
1. Introduction
2. Synthesis of aryne precursors
3. Types of reaction toward carbon-heteroatom double bond
4.1. Carbonyl compounds
4.2. Simple aldehydes and ketones
4.3. Dimethylformamide
4.4. Salicylaldehyde
4.5. Acetylacetones
4.6. Acetoacetic esters
4.7. Epoxides
5.1. Carbon-nitrogen double bonds
5.2. Oximes
5.3. Hydrazones
5.4. Azides
5.5. Nitrones
6.1. Thiocarbonyl compounds
6.2. Carbon disulfide
6.3. Dithioesters
6.4. Thiones
6.5. Thioaldehydes
7.1. Selenium compounds
7.2. Selones
7.3. Selenoureas
1. INTRODUCTION
Arynes are reactive intermediates that have enthralled organic chemists due to their vast applications in the synthesis of functional materials. Fundamental research on the reaction of arynes was carried out in the 1950s and the 1960s.1 Arynes are regarded as routine synthetic intermediates, particularly for Diels-Alder and 1,3-dipolar type cycloaddition reactions and reactions with nucleophiles, such as amines.2 Although the formation and properties of arynes were recently reviewed by many authors, their reactions with carbon-heteroatom double bonds were relatively rare.3 As the original methods for the formation of benzynes involved base-induced reactions of halo- or dihalobenzenes, carbonyl compounds cannot tolerate the presence of strong bases.4
Carbonyl compounds are known to play a vital role in organic chemistry. In this regard, a vast array of syntheses and reactions are described in every textbook of organic chemistry. The reaction of arynes with amines or N-containing heterocyclic compounds (pyridines, pyrroles, and acridines) has been extensively studied by many authors.5 However, those studies were severely impeded by several difficulties in the formation of arynes from their precursors under basic or thermal reaction conditions. Recent study of the chemistry of arynes has shown extensive progress because of the availability of benzyne precursors, 2-(trimethylsilyl)phenyl triflate (1) or phenyl[2-(trimethylsilyl)phenyl]iodonium triflate (2), which form benzyne under very mild conditions (Figure 1). In this review, we describe the synthesis of aryne precursors reported recently and the reaction of arynes with carbon-heteroatom double bonds.
2. SYNTHESIS OF ARYNE PRECURSORS
Although a number of examples of the generation of arynes were described in reviews reported until 1983,6 many of them required strong bases, oxidants, or elevated temperatures. There were only a few precursors that generated benzyne nearly quantitatively under very mild conditions (at ambient temperature and under neutral conditions). In 1983, Kobayashi and coworkers reported the synthesis of a new type of benzyne precursor, triflate 1, which requires only fluoride ion for generation. Original triflate 1 was synthesized by a three-step reaction starting from 2-chlorophenol (3) (Scheme 1).7
Recently, the emergence of a vast number of aryne reactions using triflates 1 as aryne precursors has inspired many organic chemists to improve the synthetic methods of triflates 1. As the first attempt, when substituted 2-bromophenols (6) were used as the starting material, substituted triflates 1 were synthesized conveniently (Scheme 2).8
Bronner and Garg reported another type of synthesis of triflate 1 by using phenols (8), which proceeded through carbamate intermediates (10) (Scheme 3).9
Kirkham et al. reported the synthesis of substituted triflates 1 via the cycloaddition of pyrone derivatives (11) to alkynylboronates (12), followed by oxidation and reaction with triflic anhydride (Scheme 4).10
Kirkham’s group also reported the synthesis of 2-(trimethylsilyl)iodobenzene (16), which involved reacting pyranones (14) with trimethylsilyliodoacetylene (15), which also worked as an aryne precursor, to give Diels-Alder adducts (17) and small amounts of iodobenzenes (18) as minor products (Scheme 5).11
Recently, an improved synthesis of triflate 1 was reported by Atkinson et al. by using 2-chlorophenol 3 as the starting material (Scheme 6).12
Interestingly, Ikawa et al. reported a similar type of reagent, 2-(trimethylsilyl)phenyl nonaflate (20), which was obtained by reacting 2-(trimethylsilyl)phenols with nonafluorobutanesulfonyl fluoride in the presence of Cs2CO3. This reagent can be used without isolation (Scheme 7).13
Another benzyne precursor, iodonium triflate 2, was developed by Kitamura et al., which required only two steps from 1,2-dichlorobenzene (22) (Scheme 8).14
An improved synthesis of similar benzyne precursors (27) was developed by the same authors (Scheme 9).15
These aryne precursors play important roles in the recent progress of aryne chemistry. The reactions of arynes with carbon-heteroatom double bonds are shown in the following sections.
3. TYPES OF REACTION TOWARD CARBON-HETEROATOM DOUBLE BOND
As arynes are electron-deficient,1-3 they are regarded as superelectrophiles that rapidly react with dienes and 1,3-dipoles. Generally, arynes react with dienes or 1,3-dipoles to afford the corresponding [4+2] cycloadducts or 1,3-dipolar adducts. However, the reaction with carbon-heteroatom double bonds behaves in different manners. Benzyne is generally represented by three forms: ionic, triple-bonded, and biradical (Figure 2).1-3
Accordingly, there are three types of reaction patterns. In the first reaction pattern, the addition of nucleophilic heteroatoms to benzyne yields zwitterion intermediates, which further react with electrophiles to give 1,2-disubstituted benzenes (Type 1). Ring expansion products would be obtained if the intramolecular reaction proceeded. In the second reaction pattern, unsaturated carbon-heteroatom double bonds behave as hetero Diels-Alder dienes or 1,3-dipoles that react with benzyne to give the corresponding adducts (Type 2). In the third reaction pattern, carbon-heteroatoms attack benzyne to furnish biradical intermediates that are generally proton-transferred products or four-membered cycloadducts (Type 3). The reactions in the following sections are categorized according to these three types of reaction patterns (Figure 3).
4.1. CARBONYL COMPOUNDS
The reactions of benzyne with carbonyl compounds have not been reported until the 1960s due to the labile nature of carbonyl compounds toward such bases as alkyllithiums and amides. The formation of benzyne by using strong bases has hindered its ability to react with carbonyl compounds, such as benzaldehyde and acetone. Stiles et al. reported a new benzyne precursor, benzenediazonium-2- carboxylate (28), which requires only slightly elevated temperature for the formation of benzyne.16 However, the reaction of carboxylate 28 with acetone afforded 1,3-benzodioxa-4-ones (29), which suggested that the formation of benzyne was difficult under these conditions (Scheme 10).17
Tropone (30) reacted with benzyne that was generated from the thermolysis of carboxylate 28 at 40 °C to give [4 + 2] cycloadduct (31) predominantly in 40% yield. This reaction constitutes the first example of the Diels-Alder reaction and is representative of troponoid reactions (Type 2) (Scheme 11).18 Another type of cycloaddition reaction was reported by Campbell and Rees. 2,3,4,5-Tetraphenylcyclopentadienone (32) reacted with benzyne prepared by the oxidation of 1-aminobenzotriazole (33) to afford initially [4+2] adduct (34), which lost carbon monoxide to afford 1,2,3,4-tetraphenylnaphthalene (35) in 95% yield (Type 2) (Scheme 12).19
4.2. SIMPLE ALDEHYDES AND KETONES
The first true reaction of benzyne with carbonyl compounds was reported by Heaney and McCarty. They reacted benzaldehyde with tetrachlorobenzyne (36) prepared from tetrachlorobenzene- diazonium-2-carboxylate (37) to afford 1,3-dioxane (38), a 1:2 adduct (Type 1) (Scheme 13). A similar type of adduct (39) was obtained by reacting benzaldehyde with 1-(2-carboxyphenyl)-3,3-dimethyltriazine (40) (Scheme 14).20
The reaction of α,β−unsaturated aldehydes with benzyne afforded a mixture of [2+2] (41) and [4+2] (42) adducts (Types 1 and 2) (Scheme 15).21
After these observations, there was no report of the reaction of benzyne with simple aldehydes or ketones. Yoshida et al. reinvestigated this reaction under different conditions by using triflate 1 as the benzyne precursor, which resulted in the formation of xanthenes (43), another type of 1:2 adduct, in 22-70% yields (Types 1 and 2) (Scheme 16).22
They also found that three-component coupling occurred through the reaction of arynes with aminosilanes (44) and carbonyl compound, which resulted in the formation of 2-aminobenzhydrols (45). Aminosilanes 44 initially reacted with arynes to afford zwitterion intermediates (46), which further reacted with carbonyl compounds to afford benzhydrols 45 (Type 1) (Scheme 17).23
Recently, carbon-carbon insertion products (47) were isolated by reacting trifluoroacetyl derivatives (48) with arynes (Type 1) (Scheme 18).24
4.3. DIMETHYLFORMAMIDE
The reaction of benzyne with dimethylformamide (DMF) was reported by Yaroslavsky in 1965. Carboxylate 28 reacted with DMF to afford salicylaldehyde (49) in 32% yield. This is the first true addition reaction of an amide bond (Type 1) (Scheme 19).25 The first practically useful application of C-N insertion of this type of reaction was reported by Yoshida et al. Treatment of triflate 1 and CsF in 1,3-dimethyl-2-imidazolidinone (50) and other urea derivatives resulted in the formation of benzodiazepinones (51) in 53-89% yields (Scheme 20).26
Yoshioka et al. recently found that benzoxete intermediates (52) were initially formed by reacting arynes with DMF, which further reacted with dialkylzinc to afford three-component reaction products (53) (Type 1) (Scheme 21).27
Interestingly, Yoshida et al. reported the reaction of triflate 1 with β-keto esters (54) in DMF. DMF initially reacted with aryne to give benzoxete intermediate 52, which further reacted with esters 54 to afford coumarine derivatives (55). Thus, another type of three-component reaction proceeded (Type 1) (Scheme 22).28
We have also attempted the reaction of DMF with benzyne prepared from triflate 1 in the presence of a small amount of water, which resulted in the formation of xanthols (56). On the other hand, when acetylimidazole (57) was used as the substrate, 9-N,N-diphenylaminoanthracene (58) was obtained in 85% yield (Types 1 and 2) (Scheme 23).29
The insertion of arynes into amide bonds was observed when trifluoroacetanilides (59) were treated with triflates 1 in the presence of CsF (Type 1) (Scheme 24).30
When acetanilides (61) were used instead of 59, only phenylated products (62) were obtained (Type 1) (Scheme 25).31
However, when a similar reaction was carried out by using benzanilides (63) or pivaloanilides (64) as the starting anilides, insertion products (65) were obtained (Type 1) (Scheme 26).32
4.4. SALICYLALDEHYDE
As shown in the previous section, the reaction of benzyne with DMF gave salicylaldehyde 49 in 32% yield.25 Recent reports have suggested that benzaldehydes react with benzyne to afford initially benzoxete derivatives, which further react with other electrophiles to give three-component reaction products.27,28 Thus, we speculated that initial salicylaldehyde product 49 would further react with benzyne to afford xanthols 56. Actually, when salicylaldehyde 49 was treated with triflate 1 and CsF in acetonitrile at rt, xanthenes 66 and xanthones 67 were obtained in moderate yields. When 2-hydroxyacetophenone (68) was used as the ketone, 9-methylenexanthene (69) was isolated in 86% yield (Type 1) (Scheme 27).33
4.5. ACETYLACETONES
One of the most improved reactions of benzyne with ketones is the reaction with β-diketones (70) and β-keto esters (71). Yoshida et al. found that the reaction of acetylacetone with benzyne derived from triflate 1 and KF afforded carbon-carbon insertion products (72). Malonic esters also reacted with benzyne to afford carbon-carbon insertion products (Type 1) (Scheme 28).34
Interestingly, Yang et al. reported the same reaction by using carboxylate 28 as the benzyne precursor in the presence of CuBr, which resulted in the formation of phenylation products (73) (Type 1) (Scheme 29).35
4.6. ACETOACETIC ESTERS
Tamber and Stoltz reported the reaction of arynes with acetoacetic esters (71), which resulted in the formation of carbon-carbon insertion products (74). They applied this method to the ring expansion reaction (Type 1) (Scheme 30).36
They applied this method also to the synthesis of 3-hydroxyisoquinolines (75). The utility of this approach is demonstrated in the synthesis of the atropisomeric P,N-ligand, QUINAP (76) (Scheme 31).37
As already shown, Yoshida et al. demonstrated that the reaction of arynes with DMF gave ortho-quinone methide intermediates, which further reacted with malonic esters to afford coumarines 55 in good yields (Types 1 and 2) (Scheme 22).28
4.7. EPOXIDES
In a review published in 1967, Hoffmann described that the reaction of styrene oxide (77) with benzyne gave initially a zwitterion intermediate that collapsed either by ring closure to form 3-phenyldihydrobenzofuran (78) or by intramolecular proton transfer to form ether (79) as a private communication (Type 1) (Scheme 32).38
After 40 years, Guitan et al. reported the same reaction by using triflate 1 as the benzyne precursor, which resulted in the formation of benzofuran 78 along with ethers 79 (Type 1) (Scheme 33).39
We have also reported a three-component reaction of benzyne with epoxides 77 and chloroform, which resulted in the formation of ω-trichloroalkyl phenyl ethers (80 and 81) (Type 1) (Scheme 34).40 The reaction of oxetane (82), THF, and tetrahydropyran with benzyne and chloroform afforded corresponding ω-trichloroalkyl phenyl ethers (83).41
5.1. CARBON-NITROGEN DOUBLE BONDS
Although the reactions of benzyne with pyridine or acridine were reported in the past, there were few reports on the reaction of imines with benzyne until 1975. Nakayama et al. reported the reaction of benzyne with benzylideneanilines (84), which provided 2+2 and 4+2 cycloaddition derived products (85 and 86) in 35 and 11% yields, respectively (Type 1) (Scheme 35).42
Fishwick et al. confirmed the feasibility of 2+2 addition reaction by isolating dihydroacridine (87) along with the above adducts (Type 1) (Scheme 36).43
Interestingly, Singal and Kaur reported the isolation of 1,2-diarylbenzazetines (88) by reacting carboxylate 28 with benzylideneanilines 84 (Scheme 37).44
Meanwhile, Shou et al. reported the one-pot synthesis of phenanthridines (89) by reacting carboxylate 28 with benzaldehydes and anilines (Scheme 38).45 Thus, the result for isolation of benzazetines 88 requires further investigation (Type 2).
5.2. OXIMES
The synthesis of phenanthridines (90) by the Pd-catalyzed annulation of acyloximes (91) with arynes was achieved by Gerfaud et al. (Type 2) (Scheme 39).46
Yoshida et al. reported the three-component reaction of benzyne with imines and CO2, which resulted in the formation of benzoxazinones (92) in good yields (Type 1) (Scheme 40).47
5.3. HYDRAZONES
Other reactions with carbon-nitrogen double bonds have been investigated for their potential use in the synthesis of nitrogen-containing heterocycles. For example, Larock and coworkers reported the synthesis of pyrazoloindazolones by the 1,3-dipolar cycloaddition reaction of arynes with azomethine imines.48 They also reported the synthesis of 2-(dimethylamino)aryl ketones (93), which are biologically important ketones, by reacting arynes with 1,1-dimethylhydrazones of aldehydes (94) (Type 1) (Scheme 41).49
When N-tosylhydrazones (95) were used, a completely different pathway proceeded to afford N-tosylindoles (96) in good yields (Type 1) (Scheme 42).50
N-Tosylhydrazones 95 also reacted with arynes prepared from triflate 1 and CsF in the presence of triethylbenzylammonium chloride in THF at 70 oC to afford 3-substituted indazoles (97) in 33-99% yields (Scheme 43).51
5.4. AZIDES
Although the reaction of azides (98) with benzyne was already reported by Wittig and Hoffman as well as Reynolds in the past, Larock and Zhang reinvestigated this reaction by using triflate 1 as the benzyne precursor. In addition, without having to isolate aromatic azides, the reaction proceeded smoothly to give benzotriazoles (99) in good yields (Type 2).52 The microwave-assisted reaction of azides with aryne was also reported (Scheme 44).53
Recently, many applications using this methodology were reported. The regioselective 1,3-dipolar addition reaction of disubstituted triflate 1 with various alkyl azides 100 afforded benzotriazole analogues 101 of several anti-Helicobacter pylori antibiotics (Scheme 45) (Type 2).54
Oxadisilole-fused benzotriazole derivatives (102) were synthesized by the 1,3-dipolar cycloaddition reaction of various azides 98 with arynes generated in situ from benzobisoxadisilole (103) in 56-92% yields (Scheme 46) (Type 2).55
Glycosyl azides 100 also reacted with benzyne generated in situ from triflate 1 and CsF to give 1,2,3-benzotriazole-linked glycoconjugates (104) in good yields with high stereoselectivity (Scheme 47).56
5.5. NITRONES
Nitrones (105) reacted with arynes to afford corresponding 1,3-dipolar cycloadducts, benzoisoxazolines (106) (Type 2) (Scheme 48).57
Wu et al. reported that when benzyne precursor (27) was prepared in situ, a similar type of 1,3-dipolar reaction proceeded in the presence of fluoride anion and nitrones to afford benzoisoxazolines 106 (Scheme 49).58
A new type of reaction was developed, which involved the silver triflate catalyzed reaction of 2-alkylbenzaldoxime (107) with aryne derived from triflate 1, resulting in the formation of 2-oxa-6-azabicyclo[3.2.2]nona-6,8-dienes (108). The intermediates of this reaction were isoquinoline N-oxides (109), one of the nitrone derivatives (Type 2) (Scheme 50).59
6.1. THIOCARBONYL COMPOUNDS
Due to the weakness of the C=S bond, thiocarbonyl compounds are more reactive than their C=O counterparts. Thioketones are considered to be super dipolarophiles and dienophiles that rapidly combine with 1,3-dienes in Diels-Alder cycloaddition reactions. Thus, thiones are more likely to react with benzyne to give the corresponding Diels-Alder adducts. However, only the intramolecular reaction of arynes with thioamides was reported in 1960. Treatment of thiobenz-(o-bromo)-anilide (110) with potassium amide in liquid ammonia afforded 2-phenylbenzothiazole (111) in 90% yield (Type 1) (Scheme 51).60
Dittmer and Whitman reported the reaction of carboxylate 28 with thiobenzophenone (112) in the hope of obtaining a benzyne-thione adduct. However, the obtained product was 2,2-diphenyl-4H- benzo[1,3]oxathiin-4-one (113), not the benzyne adduct (Scheme 52).61
This result clearly showed that carboxylate 28 is not adequate for the formation of benzyne.
6.2. CARBON DISULFIDES
Some carbon-sulfur double bonds have reacted with benzyne to afford the corresponding adducts. Nakayama reported the reaction of benzyne with carbon disulfide. Benzyne that was generated by the oxidation of 1-aminobenzotriazole or the decomposition of carboxylate 28 or 2-carboxybenzenediazonium chloride reacted with carbon disulfide to give the resonance-stabilized and nucleophilic carbene, which could be dimerized to give bi-(1,3-benzodithiol-2-ylidene) (114) (Type 2) (Scheme 53).62
6.3. DITHIOESTERS
The reaction of dithioesters (115) with benzyne was reported by Paquer et al. Initially formed sulfur ylide (116) reacted with water to give thioacetal, which finally yielded aldehydes and (2-methylthio)phenyl disulfide (117).63 A similar type of reaction that involved benzo-1,3-dithiole-2-thione (118) and benzyne was reported by Nakayama et al. In this case, sulfonium ylide intermediate further reacted with HCl to give sulfonium chloride (119) (Type 1) (Scheme 54).64
We have reported the reaction of 3,3,5,5-tetramethyl-4-thioxothiolane-2-thione (120) with benzyne, which resulted in the formation of four-membered spirocyclic 1,3-benzodithioles (121) (Type 1) (Scheme 55).65
Interestingly, the reaction of 1,2-dithiolane-3-thione (122) with triflate 1 in the presence of tetrabutylammonium fluoride (TBAF) at rt gave the same benzo-1,3-dithiole 121 in 85% yield (Type 1) (Scheme 56).66
The reaction of 3-hydroxy-2,2,4-trimethylpent-3-enoic acid dithiolactone (123) with triflate 1, followed by the addition of TBAF, gave colorless crystals of benzo-1,3-dithiol (124). When benzothiophene-1-thione (125) was allowed to react with carboxylate 28, another type of benzo-1,3-dithiol 124 was obtained (Scheme 57).67
Three-membered cyclic thioesters, α-dithiolactone (125), were synthesized by reacting thioketene S-oxide (126) with Lawesson reagent, the intermediate of which was thioketene S-sulfide. The reaction of α-dithiolactone 125 with dimethyl acetylenedicarboxylate (127) gave 1,3-dithiole (128) (Scheme 58).68
The reaction of dithiolactone 125 with triflate 1 in the presence of TBAF at rt gave 1,3-benzodithiole (129). (Scheme 59).69
6.4. THIONES
The first true example of the reaction of simple thiones with benzyne was reported in 1996. The reaction of thiobenzophenone 112 with benzyne prepared from 2-trimethylsilylphenyliodonium triflate 2 in the presence of TBAF resulted in the formation of [4+2] cycloadducts (130) (Type 2) (Scheme 60).70
Two years later, the reaction of thiopivalophenone (131) with benzyne gave corresponding benzothietes (132) in 60% yield. Thus, the true reaction of thiones with benzyne was finally accomplished (Scheme 61).71
Tropothione (133) reacted with benzyne generated from carboxylate 28 in dichloromethane at 40 oC to give an inseparable mixture of benzo-2H-cyclohepta[b]thiophenes (134) in 87% yield. The reaction mechanism was discussed on the basis of DFT calculations. Benzyne was added to the thione via the one-center C-S formation. The α-hydrogen shift of the formed singlet biradical intermediate resulted in the formation of a cumulene intermediate, which finally produced a mixture of benzothiophene derivatives 134 (Type 3) (Scheme 62).72
Recently, Biswas and Greaney reported the reaction of arynes with thioureas (135), which resulted in the formation of amidines (136) as formal C=S insertion products (Type 1) (Scheme 63).73 The reaction of benzyne with dimethylthioformamide (137) gave diphenyl sulfide, xanthene, xanthone, and dimethyldiphenylammonium triflate (138) in 60%, 10%, 12%, and 60% yields, respectively (Type 1) (Scheme 64).29
6.5. THIOALDEHYDES
There is only one example of the reaction of thioaldehyde with benzyne, which appeared in 1999. The reaction of 2,4,6-tri-tert-butylthiobenzaldehyde (139) with benzyne derived from triflate 1 in the presence of TBAF afforded 5,7-di-tert-butyl-3,3-dimethylindan-1-yl phenyl sulfide (140) in 92% yield. The reaction proceeded through a radical intermediate (Type 3) (Scheme 65).74
7.1. SELENIUM COMPOUNDS
The reaction of benzyne with selenium compounds was relatively unknown due to the general instability of selenium compounds. Campbell and Rees carried out the reaction of benzyne generated from the oxidation of 1-aminobenzotriazole (141) with selenium dioxide, which resulted in the formation of selenanthrene (142) in 6% yield (Type 1) (Scheme 66).75
The selenium-selenium insertion reaction of benzyne was reported by Toledo et al. Treatment of diaryl diselenide (143) with 2 equiv of triflate 1 and 4 equiv of CsF in acetonitrile at rt gave 1,2-bis(arylseleno)benzenes (144) in moderate yields (Scheme 67).76
7.2. SELENOKETONES
The reaction of di-tert-butyl selenoketone (145) with benzyne derived from triflate 1 gave mainly a 2+2 cycloadduct (146). However, in the reaction with selenofenchone (147), 2 equiv of benzyne reacted with 147 to afford corresponding selenoxane (148) (Scheme 68).77
7.3. SELENOUREAS
The reaction of triflates 1 with selenourea derivatives (149) gave diaryl diselenides 143 in 64-83% yields (Type 1), whereas the reaction with selenoazadienes (150), followed by the addition of NaBH4, gave 4H-1,3-benzoselenazines (151) (Type 2) (Scheme 69).78
CONCLUSION
The renaissance that occurred in the study of benzyne chemistry in the last decade has been mainly due to the development of methods for the formation of benzyne precursors. A variety of synthetic transformations for constructing multisubstituted aromatic compounds have been developed by the use of newly designed aryne precursors. Notably, triflate 1 reacted with fluoride anion gradually under such conditions as CsF in acetonitrile, KF/crown ether in THF, or TBAT in THF to give further addition to the initially formed benzyne adducts. Carbon-heteroatom double bonds reacted with benzyne to afford zwitterion intermediates, which, upon further reaction with electrophiles, gave ortho substitution or ring expansion products. Thus, the development of more convenient and economical procedures for the synthesis of benzyne precursors remains a challenging task in organic chemistry. The new procedures will allow us to obtain a variety of heterocycles containing N, O, S, and Se.
References
1. For a recent review, see C. Wentrup, Aust. J. Chem., 2010, 63, 979. CrossRef
2. R. W. Hoffmann, ‘Dehydrobenzene and cycloalkynes’ Academic Press, New York, 1967.
3. T. Kitamura, Aust. J. Chem., 2010, 63, 987; CrossRef H. Pellissirr and M. Santelli, Tetrahedron, 2003, 59, 701; CrossRef H. H. Wenk, M. Winkler, and W. Sander, Angew. Chem. Int. Ed., 2003, 42, 502; CrossRef D. Peňa, D. Pérez, and E. Guitián, Angew. Chem. Int. Ed., 2006, 45, 3579; CrossRef H. Yoshida, J. Ohshita, and A. Kunai, Bull. Chem. Soc. Jpn., 2010, 83, 199. CrossRef
4. G. Wittig, Naturwissenschaften, 1942, 30, 696; CrossRef J. D. Roberts, H. E. Simmons Jr., L. A. Carlsmith, and C. W. Vaughan, J. Am. Chem. Soc., 1953, 75, 3290. CrossRef
5. G. Wittig and W. Behnisch, Chem. Ber., 1958, 91, 2358; CrossRef G. Wittig and K. Niethammer, Chem. Ber., 1960, 93, 944; CrossRef E. K. Fields and S. Meyerson, J. Org. Chem., 1966, 31, 3307. CrossRef
6. T. L. Gilchrist, ‘The chemistry of triple-bonded functional groups’ Part 1. ed. by S. Patai and Z. Rappoport, John Wiley & Sons, Chichester, 1983, Chapter 11; E. K. Fields, ‘Organic reactive intermediates’, ed. by S. M. McManus, Academic Press, New York, 1973, Chapter 7; R. H. Levin, ‘Reactive intermediates’, ed. by M. Jones and R. A. Moss, John Wiley & Sons, New York, 1978, Chapter 1.
7. Y. Himeshima, T. Sonoda, and H. Kobayashi, Chem. Lett., 1983, 1211. CrossRef
8. D. Peňa, A. Cobas, D. Pérez, and E. Guitián, Synthesis, 2002, 1454. CrossRef
9. S. M. Bronner and N. K. Garg, J. Org. Chem., 2009, 74, 8842. CrossRef
10. J. D. Kirkham, P. M. Delaney, G. J. Ellames, E. C. Row, and J. P. A. Harrity, Chem. Commun., 2010, 46, 5154. CrossRef
11. J. A. Crossley, J. D. Kirkham, D. J. Browne, and J. P. A. Harrity, Tetrahedron Lett., 2010, 51, 6608. CrossRef
12. D. J. Atkinson, J. Sperry, and M. A. Brimble, Synthesis, 2010, 911. CrossRef
13. T. Ikawa, T. Nishiyama, T. Nosaki, A. Takagi, and S. Akai, Org. Lett., 2011, 13, 1730. CrossRef
14. T. Kitamura and M. Yamane, J. Chem. Soc., Chem. Commun., 1995, 983; CrossRef T. Kitamura, M. Yamane, K. Inoue, M. Todaka, N. Fukatsu, Z. Meng, and Y. Fujiwara, J. Am. Chem. Soc., 1999, 121, 11674. CrossRef
15. T. Kitamura, Z. Meng, and Y. Fujiwara, Tetrahedron Lett., 2000, 41, 6611; CrossRef Y. L. Chen, J. Q. Sun, X. Wei, W. Y. Wong, and A.W. M. Lee, Tetrahedron Lett., 2002, 43, 2259. CrossRef
16. M. Stiles, R. G. Miller, and U. Burckhardt, J. Am. Chem. Soc., 1963, 85, 1792; CrossRef F. M. Logullo, A. H. Seiz, and L. Friedman, Org. Synth. Collect. Vol. 5, 54 (1973).
17. C. P. Chiusoli and C. Venturello, Chem. Commun., 1969, 771. CrossRef
18. J. Ciabattoni, J. E. Crowley, and A. S. Kende, J. Am. Chem. Soc., 1967, 89, 2778. CrossRef
19. C. D. Campbell and C. W. Rees, J. Chem. Soc. C, 1969, 748. CrossRef
20. H. Heaney and C. T. McCarty, Chem. Commun., 1970, 123; J. Nakayama, M. Yoshida, and O. Simamura, Chem. Lett., 1973, 451. CrossRef
21. A. T. Bowne and B. H. Levin, Tetrahedron Lett., 1974, 15, 2043; CrossRef H. Heaney and J. M. Jablonski, Chem. Commun., 1968, 1139; CrossRef H. Heaney, J. M. Jacoblonski, and C. T. McCarty, J. Chem. Soc., Perkin Trans. 1, 1972, 2903. CrossRef
22. H. Yoshida, M. Watanabe, H. Fukushima, J. Ohshita, and A. Kunai, Org. Lett., 2004, 6, 4049. CrossRef
23. H. Yoshida, T. Morishita, H. Fukushima, J. Ohshita, and A. Kunai, Org. Lett., 2007, 9, 3367; CrossRef T. Morishima, H. Fukushima, H. Yoshida, J. Ohshita, and A. Kunai, J. Org. Chem., 2008, 73, 5452. CrossRef
24. H. Yoshida, Y. Ito, Y. Yoshioka, J. Ohshita, and K. Takaki, Chem. Commun., 2011, 47, 8664. CrossRef
25. S. Yaroslavsky, Tetrahedron Lett., 1965, 1503. CrossRef
26. H. Yoshida, E. Shirakawa, Y. Honda, and T. Hiyama, Angew. Chem. Int. Ed., 2002, 41, 3247. CrossRef
27. E. Yoshioka, S. Kohtani, and H. Miyabe, Org. Lett., 2010, 12, 1956; CrossRef E. Yoshioka, S. Kohtani, and H. Miyabe, Angew. Chem. Int. Ed., 2011, 50, 6638. CrossRef
28. H. Yoshida, Y. Ito, and J. Ohshita, Chem. Commun., 2011, 47, 8512. CrossRef
29. K. Okuma, A. Nojima, Y. Nakamura, N. Matsunaga, N. Nagahora, and K. Shioji, Bull. Chem. Soc. Jpn., 2011, 84, 328; CrossRef For the synthesis of 58; see C. Xie and Y. Zhang, Org. Lett., 2007, 9, 781. CrossRef
30. Z. Liu and R. C. Larock, J. Am. Chem. Soc., 2005, 127, 13112. CrossRef
31. J. C. Haber, M. A. Lynch, S. L. Spring, A. D. Pechulis, J. Raker, and Y. Wang, Tetrahedron Lett., 2011, 52, 5847. CrossRef
32. D. G. Pintori and M. F. Greaney, Org. Lett., 2010, 12, 168. CrossRef
33. K. Okuma, A. Nojima, N. Matsunaga, N. Nagahora, and K. Shioji, Org. Lett., 2009, 11, 169. CrossRef
34. H. Yoshida, M. Watanabe, J. Ohshita, and A. Kunai, Chem. Commun., 2005, 3292. CrossRef
35. Y. Yang, W. Shou, and Y. Wang, Tetrahedron Lett., 2007, 48, 8163. CrossRef
36. U. K. Tamber and B. M. Stoltz, J. Am. Chem. Soc., 2005, 127, 5340; CrossRef D. C. Ebner, U. K. Tamber, and B. M. Stoltz, Org. Synth., 2009, 86, 161.
37. K. V. Allan, B. D. Hong, and B. M. Stoltz, Org. Biomol. Chem., 2009, 7, 4960. CrossRef
38. M. Stiles and A. Haag, personal communication in reference 2. p. 178.
39. S. B. Rodil, D. Peňa, and E. Guitan, Synlett, 2007, 1308. CrossRef
40. K. Okuma, H. Hino, A. Sou, N. Nagahora, and K. Shioji, Chem. Lett., 2009, 38, 1030. CrossRef
41. K. Okuma, Y. Fukuzaki, A. Nojima, A. Sou, H. Hino, N. Matsunaga, N. Nagahora, K. Shioji, and Y. Yokomori, Bull. Chem. Soc. Jpn., 2010, 83, 1238. CrossRef
42. J. Nakayama, H. Midorikawa, and M. Yoshida, Bull. Chem. Soc. Jpn., 1975, 48, 1063. CrossRef
43. C. W. G. Fishwick, R. C. Gupta, and R. C. Storr, J. Chem. Soc., Perkin Trans. 1, 1984, 2827; CrossRef A. A. Ali, N. K. Mohamed, A. A. Hassan, and A.-F. E. Mourad, Tetrahedron, 1999, 55, 1111. CrossRef
44. K. K. Singal and J. Kaur, Synth. Commun., 2001, 31, 2809. CrossRef
45. W.-G. Shou, Y.-Y. Yang, and Y.-G. Wang, J. Org. Chem., 2006, 71, 9241. CrossRef
46. T. Gerfaud, L. Neuville, and J. Zhu, Angew. Chem. Int. Ed., 2009, 48, 572. CrossRef
47. H. Yoshida, H. Fukushima, and A. Kunai, J. Am. Chem. Soc., 2006, 128, 11040. CrossRef
48. F. Shi, R. Mancuso, and R. C. Larock, Tetrahedron Lett., 2009, 50, 4067. CrossRef
49. A. V. Dubrovsky and R. C. Larock, Org. Lett., 2011, 13, 4136. CrossRef
50. D. McAusland, S. Seo, D. G. Pinori, J. Finlayson, and M. Greaney, Org. Lett., 2011, 13, 3667. CrossRef
51. P. Li, J. Zhao, C. Wu, R. C. Larock, and F. Shi, Org. Lett., 2011, 13, 3340. CrossRef
52. G. Wittig and R. H. Hoffmann, Chem. Ber., 1962, 95, 2718; CrossRef G. A. Reynolds, J. Org. Chem., 1964, 29, 3733; CrossRef F. Shi, J. P. Waldo, Y. Chen, and R. C. Larock, Org. Lett., 2008, 10, 2409; CrossRef F. Zhang and J. E. Moses, Org. Lett., 2009, 11, 1587. CrossRef
53. H. Ankati and E. Biehl, Tetrahedron Lett., 2009, 50, 4677. CrossRef
54. D. J. Atkinson, J. Sperry, and M. A. Brimble, Synlett, 2011, 99. CrossRef
55. Y. Lin, Y. Chen, X. Ma, W. Cao, and J. Chen, Tetrahedron, 2011, 67, 856. CrossRef
56. B. V. S. Reddy, K. Praneeth, and J. S. Yadav, Carbohyd. Res., 2011, 346, 995. CrossRef
57. R. Huisgen and R. Knorr, Naturwissenshaften, 1961, 48, 716; CrossRef T. Matsumoto, T. Sohma, S. Hatazaki, and K. Suzuki, Synlett, 1993, 843; CrossRef T. Hamura, T. Arisawa, T. Matsumoto, and K. Suzuki, Angew. Chem. Int. Ed., 2006, 45, 6842; CrossRef A. A. Ali, H. Hopf, L. Ernst, I. Dix, and P. G. Jones, Eur. J. Org. Chem., 2006, 3001. CrossRef
58. K. Wu, Y. Chen, Y. Lin, W. Cao, M. Zhang, J. Chen, and A. W. M. Lee, Tetrahedron, 2010, 66, 578. CrossRef
59. H. Ren, Y. Luo, S. Ye, and J. Wu, Org. Lett., 2011, 13, 2552. CrossRef
60. J. F. Bunnet and B. F. Hrutfiod, J. Am. Chem. Soc., 1961, 83, 1691. CrossRef
61. D. C. Dittmer and E. C. Whitman, J. Org. Chem., 1969, 34, 2004. CrossRef
62. J. Nakayama, J. Chem. Soc., Perkin Trans. 1, 1975, 525; CrossRef J. Nakayama, J. Chem. Soc., Chem. Commun., 1974, 166. CrossRef
63. D. Paquer, D. Reffet, and C. G. Andrieu, Recl. Trav. Chim. Pays-Bas, 1978, 97, 88. CrossRef
64. J. Nakayama, A. Kimata, H. Taniguchi, and F. Takahashi, Bull. Chem. Soc. Jpn., 1996, 69, 2349. CrossRef
65. K. Okuma, T. Tsubone, T. Shigetomi, K. Shioji, and Y. Yokomori, Heterocycles, 2005, 65, 1553. CrossRef
66. T. Shigetomi, A. Nojima, K. Shioji, K. Okuma, and Y. Yokomori, Heterocycles, 2006, 68, 2243. CrossRef
67. K. Okuma, A. Nojima, and Y. Yokomori, Heterocycles, 2008, 75, 1417. CrossRef
68. K. Okuma, T. Shigetomi, Y. Nibu, K. Shioji, M. Yoshida, and Y. Yokomori, J. Am. Chem. Soc., 2004, 126, 9508. CrossRef
69. T. Shigetomi, H. Soejima, Y. Nibu, K. Shioji, K. Okuma, and Y. Yokomori, Chem. Eur. J., 2006, 12, 7742. CrossRef
70. K. Okuma, T. Yamamoto, T. Shirokawa, T. Kitamura, and Y. Fujiwara, Tetrahedron Lett., 1996, 37, 8883. CrossRef
71. K. Okuma, K. Shiki, and K. Shioji, Chem. Lett., 1998, 79. CrossRef
72. S. Yamabe, T. Minato, A. Ishikawa O. Irinamihira, and T. Machiguchi, J. Org. Chem., 2007, 72, 2832. CrossRef
73. K. Biswas and M. F. Greaney, Org. Lett., 2011, 13, 4946. CrossRef
74. K. Okuma, S. Sonoda, Y. Koga, and K. Shioji, J. Chem. Soc., Perkin Trans. 1, 1999, 2997. CrossRef
75. C. D. Campbell and C. W. Rees, J. Chem. Soc. C, 1969, 752; CrossRef J. Nakayama, M. Kashiwagi, R. Yomoda, and M. Hoshino, Nippon Kagaku Kaishi, 1987, 1424. CrossRef
76. F. T. Toledo, H. Marques, J. V. Comasseto, and C. Raminelli, Tetrahedron Lett., 2007, 48, 8125. CrossRef
77. K. Okuma, A. Okada, Y. Koga, and Y. Yokomori, J. Am. Chem. Soc., 2001, 123, 7166. CrossRef
78. U. N. Rao, R. Sathunuru, and E. R. Biehl, Heterocycles, 2004, 63, 1615. CrossRef