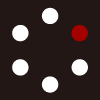
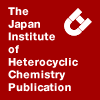
HETEROCYCLES
An International Journal for Reviews and Communications in Heterocyclic ChemistryWeb Edition ISSN: 1881-0942
Published online by The Japan Institute of Heterocyclic Chemistry
e-Journal
Full Text HTML
Received, 28th November, 2011, Accepted, 17th January, 2012, Published online, 19th January, 2012.
DOI: 10.3987/COM-11-12400
■ Cytotoxic Xanthone Glycosides from Aerial Part of Centaurium spicatum
Ahmed E. Allam, Mohamed A. El-Shanawany, Enaam Y. Backheet, Alaa M. Nafady, Fumihide Takano,* and Tomihisa Ohta
Department of Pharmacognosy and Chemistry of Natural Products, Graduate School of Natural Science and Technology, Kanazawa University, Kakuma, Kanazawa, Ishikawa 920-1192, Japan
Abstract
A new xanthone glycoside, 8-hydroxy-3,5-dimethoxy-1-O-[α-L-arabinofuranosyl-(1→6)-β-D-glucopyranosyl]-xanthone (1) in addition to two known xanthones named 8-hydroxy-3,5-dimethoxy-1-O-β-D-glucopyranosyl-xanthone (2) and 1,8-dihydroxy-3,5-dimethoxyxanthone (methylbellidifolin) (3) were isolated from the methanol extract of aerial part of Centaurium spicatum, and tested for their cytotoxic activities against three different types of cell lines HeLa, THP-1and HL-60 cell lines. All compounds showed cytotoxicities to all cell lines, whereas showed a moderate activity against human monocytic cell line HL-60. Among three tested xanthones, the cytotoxic activity of compound 1 was stronger than those of other xanthones and the IC50 values for compound 1 were 3.22 (against THP-1), 8.67 (against HeLa) and 74.6 (against HL-60) µM, respectively.Centaurium spicatum L. Fritsch (Gentianaceae) is an annual herb occurring in Southern Europe and Northern Africa where it is used together with other Centaurium species such as C. pulchellum in traditional medicines treated for abdominal pain, hypertension, gallstones, kidney and ureter stones, renal colic, wounds and diabetes. A survey of the current literatures revealed that the isolation and identification of secoiridoids, sweroside, swertiamarin and gentiopicrin, as well as polyoxygenated xanthones from the plant.1-4 Alkaloids of the pyridine type (e. g. gentianine), spicatine and the series of amides derived from the secoiridoid glucoside swertiamarin and kantaurin were also shown to be present.3 The study of xanthones is noticed not only from the chemosystematic investigation but also from the pharmacological point of view. Xanthone aglycones possessed antidepressant and antitubercular activities, while xanthone glycosides showed a depressant effect.5 A choleretic, diuretic, antimicrobial, antiviral and cardiotonic activities by some xanthones have also been established.5-8 The pharmacological interest in xanthones is based on their inhibitory effect on monoamine oxidase as well as their cytotoxic and antitumour activity.9,10 In our course study, it was found that a classification of xanthones called xanthone alpha mangostin can suppress development of malignant tumors. It was also revealed that one xanthone analogue, garcicone E, can also prevent liver, lung and colon cancers.11 In the present work, we reported the isolation and structural elucidation of a new xanthone glycoside in addition to two known xanthones from C. spicatum and were tested for their cytotoxic activities against three different tumor cell lines in vitro.
A methanol extract of C. spicatum revealed inhibitory activity of cell proliferation in cultured HeLa, THP-1 and HL-60, respectively. The n-butanol fraction from the methanol extract decreased cell viability in cultured HeLa, THP-1 and HL-60 cells with IC50 = 42 ± 8, 55 ± 7 and 180 ± 5 μg/mL respectively. The n-butanol fraction was then separated by chromatography on Diaion HP-20 resin. Fractions were successively eluted with water, 100% methanol, and 100% acetone, and biological activities were estimated. The fraction-eluted with 100% methanol showed the strongest cytotoxic activities. IC50 values for inhibitory activity in these cells were 18 ± 9 (HeLa), 22 ± 4 (THP-1) and 120 ± 6 μg/mL (HL-60), respectively. The inhibitory activities of methanol extract were 2.5- to 1.5-times higher than those of the original n-butanol extract. The 100% methanol-eluted fraction was further separated into five fractions by silica gel column chromatography using chloroform-methanol as eluents. These fractions 2 and 4, which showed a strongest activities, were then separated by HPLC equipped with ODS column, yielding three active xanthones. Two xanthones, 2 and 3, were known compounds, and identified as 8-hydroxy-3,5-dimethoxy-1-O-β-D-glucopyranosyl-xanthone 2 (18 mg) and 1,8-dihydroxy-3,5-dimethoxyxanthone or methylbellidifolin 3 (20 mg) by comparison of their spectral data with those reported in the literature (Figure 1).12,13
A new xanthone 1, 8-hydroxy-3,5-dimethoxy-1-O-[α-L-arabinofuranosyl-(1→6)-β-D-glucopyranosyl]- xanthone, was obtained as yellow needles (20 mg) with a molecular formula of C26H30O15 as determined by HRFABMS m/z 583.1673. The UV spectrum of 1 in methanol suggested a xanthone derivative.14 The 1H and 13C NMR spectrum showed the typical pattern of a xanthone glycoside skeleton where a pair of meta-coupled aromatic protons at (δH 6.74 and 6.77 ppm) assignable to H-2 and H-4, respectively in addition to a pair of ortho-coupled aromatic protons at (δH 7.36 and 6.65 ppm) assignable to H-6 and H-7 respectively were observed. Further, 1H NMR spectrum revealed presence of two methoxy (δH 3.91 and 3.85 ppm) and one hydroxyl group at δH 12.45 ppm (8-OH) (Table 1). The spectrum also showed presence of two sugar moieties where there were two doublet signals at δH 4.17 and 5.07 confirmed the presence of two sugars in the molecule. The terminal sugar moiety was suggested to be arabinose which was confirmed from 1H-NMR spectrum where the presence of a proton at δH 3.78, d, J= 3.7 (i.e. equatorial coupling) assigned for H-4 of arabinose (c.f. xylose where H-4 is of an axial coupling).15 In 13C NMR spectrum, the presence of 11 of both methin (δC 100.8, 73.2, 75.7, 69.5, 76.5, 69.6, 104.2, 73.3 and 76.3) and methylene (δC 68.6 and 65.6) signals confirmed the glycosylation of the molecule. A long-range correlation observed in HMBC experiment, between the downfield shifted C-1 of the xanthone moiety (δC 158.6) and the anomeric proton of glucose (δH 5.07) confirmed that this was the site of glycosylation and that glucose was the first sugar in turn, HMBC long range coupling between the downfield shifted C-6 of glucose (δC 68.6) and the anomeric proton of arabinose (δH 4.17) was indicative of a 1–6 linkage between the two glycosidic moieties.5 Another important long-range correlation observed in HMBC experiment between the downfield shifted C-3 (δC 165.6) of the xanthone moiety and the methoxy singlet protons (δH 3.91) from one side and between C-5 (δC 139.1) of the xanthone moiety and the methoxy singlet protons (δH 3.85) from the other side confirming the site of attachments of the two methoxy groups to C-3 and C-5 of the xanthone moiety (Figure 2). The other carbons were assigned from the combination of 1H–1H correlated spectroscopy (COSY), heteronuclear multiple quantum coherence (HMQC) and heteronuclear multiple bond correlation (HMBC) data and by comparison with the literature.16 The identification of a glucopyranosyl and an arabinofuranosyl moiety was done by means of comparison with literature data.17 In addition, acid hydrolysis of the glycosides was carried out and the sugars were identified as glucose and arabinose. Also optical rotation values of L-arabinofuranosyl tetrabenzoate derivatives of the acid hydrolysis products of 1 were [α]D25 –87.5 (c 0.825, CHCl3) for β-D-glucopyranosyl tetrabenzoate and [α]D25 +112.0 (c 0.825, CHCl3) for α-L-arabinofuranosyl tetrabenzoate which were identical with the synthetic models for both β-D–glucopyranose tetrabenzoate with [α]D31 –88.0 (c 1.0, CHCl3) and α-L-arabinofuranose tetrabenzoate with [α]D29 +112.5 (c 0.848, CHCl3) respectively.13,18-20
Also, the retention time of each of the benzoyl derivatives of the hydrolysis products L-arabinofuranosyl tetrabenzoate and D-xylopyranosyl tetrabenzoate was found to be 8.2 and 9.1 min, respectively which was identical with the retention times of synthetic models of tetrabenzoate derivatives of both L- arabinofuranosyl and D-xylopyranosyl tetrabenzoate where it was found to be 8.5 and 9.1 min, respectively19,20 which confirming both sugar moieties to be β-D-xylopyranose and α-L-arabinofuranose.
NOE correlation was also noted between H-3'' (δH 3.08) and H-5'' (δH 3.63, 3.95) protons of the L-arabinose confirmed the presence of α-L-arabinofuranosyl moiety. The combination of these data led to the identification of 1 as 8-hydroxy-3,5-dimethoxy-1-O-[α-L-arabinofuranosyl-(1→6)-β-D-gluco- pyranosyl]-xanthone and considered as a new compound. In addition, two known xanthones named 8-hydroxy-3, 5-dimethoxy-1-O-β-D-glucopyranosyl-xanthone (2) and 1,8-dihydroxy-3,5-dimethoxy- xanthone (methylbellidifolin) (3) were isolated from C. spicatum aerial part and the data were compared by those of the previously published.17,18 (Figure 1).
After establishing their structures, we investigated their cytotoxicities against cultured HeLa, THP-1 and HL-60 cells lines. Cells were treated with compounds 1-3 at various concentrations (1-100 µM) for 48 h, and determined cell viability by 3-(4,5-dimethylthiazol-2-yl)-2,5-diphenyl tetrazolium bromide (MTT) method. The cell viabilities of HeLa or THP-1 cells at 48 h were significantly decreased by treatment of these compounds in concentration-dependent manners. The IC50 values for 1, 2 or 3 against HeLa cell were 3.22 ± 0.84, 6.42 ± 0.47 and 5.28 ± 0.69 µM respectively. Cell viability in THP-1 cell treated with compounds 1, 2 or 3 also decreased, and their IC50 values were 8.67 ± 0.25 (for 1), 17.3 ± 0.14 (for 2) and 5.02 ± 0.18 (for 3) µM. However, these compounds showed moderate cytotoxicity in cultured human leukemic HL-60 in contrast to the results obtained in HeLa and THP-1 cells (Table 2). A positive control agent, mitomycin C, was also showed a cytotoxic activity against three cell lines, and its average of IC50 values in three different cell lines was approximately 10 µM (Table 2). Although an aglycone of xanthone glycoside (compound 3) still has a selective cytotoxicity against three different tumor cell lines, whose activity in the case of xanthone glycoside (i.e. compound 1) is higher than that of 3. It could therefore be discussed that the presence of sugar moieties of the tetraoxygenated xanthones would be important to show the enhancement of cytotoxicity by aglycone xanthone. In this study, we isolated a new xanthone glycoside 1 accompanying two known compounds 2 and 3, which showed a selective cytotoxicity, from the areal part of C. spicatum L.
EXPERIMENTAL
General Optical rotations were determined with a Horiba SEPA-3000 high-sensitivity polarimeter (Horiba). We determined UV spectra on a Shimadzu UV-1600 UV-visible spectrometer, IR spectra on a Shimadzu FTIR-8400 IR spectrophotometer and NMR spectra on a JEOL GSX-600 spectrometer in DMSO and CDCl3. Chemical shifts were referenced to the residual solvent peaks DMSO (δH 2.49 and δC 39.7) and CDCl3 (δH 7.2 and δC 77). Fast atom bombardment (FABMS) and high-resolution fast atom bombardment (HRFABMS) were carried out on a JEOL JMS SX-102 mass spectrometer. Reversed-phase high-performance chromatography (HPLC) was undertaken on an ODS column (particle size: 5 μm, TOSO, 18 × 250 mm) RP-23 (5 μm; Waters). Diaion HP-20 (Mitsubishi) (Tokyo, Japan), silica gel (63– 210 μm; Kanto Kagaku) and ODS (63–212 μm; Wako Pure Chemical) (Tokyo, Japan) were used for open column chromatography. Thin-layer chromatography (TLC) was carried out on silica gel (SiO2, 60-100 mesh; Wako Pure Chemical) 60 F254 and RP-18 F254S (Merck).
Plant Material Aerial part of C. spicatum L. (Gentianaceae) was collected in May 2009 from New Valley, 200 km southwest of Assiut City, Egypt. The plant was identified and authenticated by Prof. Dr. A. Fayed, Professor of Plant Taxonomy, Faculty of Science, Assiut University. A voucher specimen (T-318) was deposited at the Department of Pharmacognosy, Faculty of Pharmacy Assiut University, Assiut, Egypt and at the Department of Pharmacognosy and Chemistry of Natural Products, School of Pharmaceutical Sciences, Kanazawa University, Kanazawa, Japan.
Extraction and isolation Air-dried C. spicatum aerial parts (2 kg) were extracted thrice with MeOH (5 L) at room temperature. The solvents were combined and filtered through filter paper (Advantec MFS Incorporated). The solvent was removed under reduced pressure at 40 °C to yield the MeOH extract (550 g), which was partitioned between distilled water and EtOAc (1 L of each) to give the aqueous fraction (300 g) and the EtOAc fraction (80 g). The aqueous fraction was further partitioned by n-BuOH to give the n-BuOH fraction (100 g) and the rest aqueous fraction (140 g). The EtOAc fraction was in turn partitioned between 90% MeOH and n-hexane to give 90% MeOH fraction (50 g) and n-hexane fraction (20 g). The n-BuOH fraction (100 g) was separated on a Diaion HP-20 column using water (2 L), MeOH (25%, 50%, 75%, and 100%) (2 L of each) and acetone (2 L) to give three crude fractions. Individual fractions water fraction (40 g), MeOH fraction (15.4 g) and the acetone fraction (5 g) were obtained after removal and evaporation of the respective solvents. The MeOH-eluted fraction (25 g) was further separated by chromatography on an ODS column (80 × 200 mm) using five concentrations of MeOH -H2O (10, 30, 50, 60, and 90% v/v; elution volume: 1.5 L each) to give six corresponding fractions. The biologically active fraction eluted with 50% MeOH (15.4 g) was further separated by chromatography on an silica gel column (80 × 200 mm) with a step-wise gradient of CHCl3 and MeOH (9:1, 8:2, 7:3, 6:4, 5:5, 4:6, 0:1) to give seven corresponding fractions. The fraction eluted with 9:1 gives compound 3 (20 mg). The fraction eluted with 4:6 (280 mg) was further separated by chromatography on an ODS column (80 × 200 mm) (Cosmosil 140 C18 PREP, Nacalai Tesque, Tokyo, Japan) using seven concentrations of MeOH-H2O (10, 25, 40, 50, 60, 70, and 90% v/v; elution volume: 200 mL of each) to give seven corresponding fractions. A 150-mg portion of the fraction eluted with 25% v/v MeOH-H2O was further separated by preparative HPLC, ODS column: C30 UG-5, 20 mm× 250 mm. particle size: 5 μm, flow rate: 6 mL/min. (Develosil, Nacalai Tesque, Tokyo, Japan) equipped with a UV detector (210 nm). The mobile phase was 25% v/v MeOH-H2O which resulted in elution of compound 2 (18 mg). These preparative HPLC conditions were also used after gradually increasing the mobile phase to 50% v/v MeOH-H2O giving compound 1 (20 mg). Two of the three isolated compounds were identified as 8-hydroxy-3,5-dimethoxy-1-O-β-D-glucopyranosyl-xanthone (2) and 1,8-dihydroxy-3,5-dimethoxy-xanthone or methylbellidifolin (3) by comparison of their spectral data with those reported in the literature.17,18 The elution profile of the fraction eluted with 4:6 CHCl3-MeOH of C. spicatum aerial part was obtained by HPLC using the mobile phase of 25%, 35%, 50% MeOH. The two main peaks originating from compound 2 (tR, 35.5 min, mobile phase: 25% MeOH) and compound 1 (tR, 46.4 min, mobile phase: 50% MeOH) were found under the analytical condition. Each peak at the individual retention time was compared with purified samples.
Compound 1 8-Hydroxy-3,5-dimethoxy-1-O-[α-L-arabinofuranosyl-(1→6)-β-D-glucopyranosyl]- xanthone, yellow needles (20 mg); [α]D25 -109.9° (c 0.333, MeOH). UV (CH3OH) λmax (log ε) 234, 264, 318, and 375 nm; IR νmax (KBr) 3445, 1783, 2987, 1645, 1533 cm-1; for 1H NMR spectroscopic data (600 MHz, CD3OD) and 13C NMR spectroscopic data (125 MHz, CD3OD). HRFABMS m/z 583.1673 [M+H]+ (calcd for C26H30O15). FAB+ MS m/z 289 [aglycone] +, 583 [M+H] + and 605 [M+Na]+.
Acid hydrolysis Acid hydrolysis of the glycosides was carried out by refluxing 5 mg of compound in 5 mL of 6% HCl in MeOH for 3 h. The reaction mixture was partitioned against EtOAc (3 × 10 mL). The aglycone was obtained from the EtOAc layer and the aqueous layer was evaporated and developed crystal needles with EtOAc–H2O–MeOH–HOAc (13:3:3:4). Identification of glucose, arabinose and xylose present in the sugar fraction was carried out by comparison with authentic samples of glucose (Rf 0.48), arabinose (Rf 0.50) and xylose (Rf 0.55) (Sigma) (Tokyo, Japan) in TLC over silica gel (CHCl3- MeOH-H2O 8:5:1) using 5% H2SO4 in MeOH as spraying reagent followed by heating the plates at 120 °C for 15-20 min.
Secondary sugar units Benzoyl chloride (0.5 mL) was added to each ice-cooled solution of either D-glucopyranose or L-arabinofuranose in dry pyridine (1.0 mL) and each mixture was stirred at room temperature for 15 h. MeOH (1.0 mL) was added dropwise to the reaction mixture, stirred for 30 min and then diluted with EtOAc and aqueous Na2CO3 and the layers were separated. Each organic layer was washed with brine and the combined aqueous layers for each were extracted with EtOAc. Each combined organic extracts were dried over MgSO4 and concentrated. Each residual dark brown oil was purified by silica gel cc (eluting with hexane/EtOAc 5:1) to give both corresponding tetrabenzoyl derivatives. The tetrabenzoyl derivatives of each hydrolysis products of 1 were subjected to chiral HPLC analyses using CHIRALPAK IB (Daisel Chemical Industries, 4.6 × 250 mm; MeOH, 1.0 mL/min; UV detection at 254 nm).
Cytotoxic assay To determine the cytotoxic activities of the tested samples by HeLa, THP-1 or HL-60 cell lines, individual cells (Dainippon Pharmaceutical Company, Tokyo, Japan) were cultured and maintained in appropriate medium such as D-MEM (Sigma, St. Louis, MO, USA) (for HeLa) or RPMI-1640 (Sigma, St. Louis, MO, USA) (for THP-1 and HL-60) media. Cell suspended in medium were seeded at 1.0 × 105 cells/mL into 96-well tissue culture plates and then incubated at 37 °C in a humidified CO2 incubator.21 The tested samples (purity > 93%) (20 μL in DMSO/PBS) at various concentrations were added in each well. After 48-h cultivation, supernatants were removed and adherent cells (HeLa) incubated with 3-(4,5-dimethylthiazol-2-yl)-2,5-diphenyltetrazolium bromide (MTT, 20 μL, 5 mg/mL in PBS) for 4 h, and then solubilized with 10% (w/v) sodium dodecyl sulfate (SDS; in 60% [v/v] dimethylformamide) solution (50 μL) for 18 h. The plate was then centrifuged at 1,200 rpm for 5 min to precipitate wells and formazan. The absorbance was measured at 570 nm using a microplate reader and the cytotoxicity calculated by comparing absorbance with that of the non treated control culture. Cell growth curve was graphed using statistical analysis software (Kaleida Graph version 4.00; Synergy Software) and IC50 values calculated using simple linear regression. IC50 values were calculated from dose response curves for a 48 h drug exposure. Data were obtained through independent measurements of cell density and cell viability by the MTT assays.22 A dose– response curve was plotted for each compound and the IC50 value was calculated as the concentration of the test compound resulting in 50% reduction of optical density compared with the control. The IC50 values of three experiments and correlation coefficient values for the tested samples in each experiment were calculated using the same software. Differences were considered significant at p < 0.005.
References
1. M. El-Shanawany, M. Makboul, and A. Abdel Baky, Bull. Pharm. Sci. Assiut Univ., 1989, 12, 416.
2. M. El-Shanawany, A. Samir, A. Gamal, M. Alaa, and R. Sabrin, Phytochem. Lett., 2011, 2, 126. CrossRef
3. D. Bishay, S. Ross, and P. Hylands, Planta Med., 1979, 37, 253. CrossRef
4. W. Van der Sluis and R. Labadie, Planta Med., 1981, 3, 221. CrossRef
5. K. Hostettmann and H. Wagner, Phytochemistry, 1977, 16, 821. CrossRef
6. G. Kitanov and K. Blinova, Chem. Nat. Compds., 1987, 2, 151. CrossRef
7. O. Suzuki, Y. Katsumata, M. Oya, V. Chari, R. Klapfenberger, H. Wagner, and K. Hostettmann, Planta Med., 1980, 39, 19. CrossRef
8. O. Suzuki, Y. Katsumata, M. Oya, V. Chari, B. Vermes, H. Wagner, and K. Hostettmam, Planta Med., 1981, 42, 17. CrossRef
9. F. Imperato, Phytochemistry, 1991, 30, 3839. CrossRef
10. Y. Hano, T. Okamoto, T. Nomura, and Y. Momose, Heterocycles, 1990, 31, 1345. CrossRef
11. M. Iinuma, H. Tosa, T. Tanaka, and S. Yonemori, Phytochemistry, 1994, 35, 527. CrossRef
12. A. Prakash, C. Basumatary, S. Ghosal, and S. Handa, Planta Med., 1982, 45, 61. CrossRef
13. M. Komatsu, T. Tomimori, and N. Mikuriya, Chem. Pharm. Bull., 1969, 17, 155. CrossRef
14. K. R. Markham, Techniques of Flavonoid Identification, Academic Press, London, 1982, 8, 39.
15. P. K. Agrawal, Carbon-13 NMR of flavonoids. Elsevier Science Publishers, Amsterdam, Oxford, New York, Tokyo, 1989.
16. C. Terreaux, M. Maillard, M. Gupta, and K. Hostettmann, Phytochemistry, 1995, 40, 1791. CrossRef
17. Y. Jiang, W. Zhang, P. Tu, and X. Xu, J. Nat. Prod., 2005, 68, 875. CrossRef
18. G. Hewitt, C. Fletcher Jr, and S. Hudson. J. Am. Chem. Soc., 1947, 69, 1145. CrossRef
19. Y. Kasai, K. Komatsu, H. Shigemori, M. Tsuda, Y. Mikami, J. Kobayashi, and A. Cladinol, J. Nat. Prod., 2005, 68, 777. CrossRef
20. T. Hasegawa, A. Tanaka A, A. Hosoda, F. Takano, and T. Ohat, Phytochemistry, 2008, 69, 1419. CrossRef
21. T. Hasegawa, F. Takano, T. Takata, M. Niiyama, and T. Ohta, Phytochemistry, 2008, 69, 747. CrossRef
22. M. Marques, T. Girão da Cruz, M. Pedroso de Lima, A. Gameiro, E. Pereira, and P. Garcia, Biochim. Biophys. Acta, 2002, 1589, 63. CrossRef