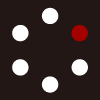
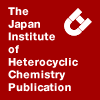
HETEROCYCLES
An International Journal for Reviews and Communications in Heterocyclic ChemistryWeb Edition ISSN: 1881-0942
Published online by The Japan Institute of Heterocyclic Chemistry
e-Journal
Full Text HTML
Received, 22nd December, 2011, Accepted, 10th February, 2012, Published online, 20th February, 2012.
DOI: 10.3987/COM-11-12415
■ 1,3-Dipolar Cycloaddition of Ethyl Diazoacetate with (E)-3-Arylidenechroman-4-ones. A New Access to Spirocyclopropane Derivatives
Nadia Wannassi, Hanene Jelizi, Mohamed El Baker Rammah, Kabula Ciamala,* Michael Knorr, Karin Monnier-Jobé, Yoann Rousselin, Marek M. Kubicki, and Carsten Strohmann
Institute of UTINAM UMR CNRS 6213, Faculty of Science and Technology, University of Franche-Comté, 16 Route de Gray, F-25030 Besançon, France
Abstract
(E)-3-Arylidenechroman-4-ones 1a–e (Ar = Ph, p-MeC6H4, p-MeOC6H4, p-MeSC6H4, p-ClC6H4) react regioselectively (100%) with ethyl diazoacetate 2 in refluxing toluene to afford in good yields spiro-Δ2-pyrazolines ethyl 4’-oxo-4-aryl-spiro[chromanone-3’,5-pyrazole]-3-carboxylate 3a–e. The occurrence of Δ1-pyrazolines as reaction intermediates has been evidenced. The regio- and stereochemistry of spiranic compounds 3a-e has been established on the basis of spectroscopic data and elemental analyses, corroborated by a single crystal X-ray crystallographic analysis of one product 3a. The spiro-adducts 3a–e were brominated by NBS to give spirocyclic-substituted 3-bromo-4,5-dihydro-3H-pyrazoles 4a–e. The latter compounds evolve straightforwardly N2 in refluxing toluene to afford substituted spirocyclic 1-bromocyclopropane-1-carboxylates. The molecular structure of cyclopropane derivative 5e has been determined by means of a single-crystal X-ray diffraction study.INTRODUCTION
Diazo compounds are remarkably versatile intermediates in organic synthesis that participate in a variety of thermal, photochemical, metal-catalyzed rearrangement, cycloaddition, and insertion reactions with concomitant expulsion of N2.1 These compounds are found in nature, examples of which include azaserine,2 members of the kinamycin3 and lomaiviticin4 families of marine natural products. The high synthetic utility and pharmacological importance of ethyl diazoacetate prompted Curtius and Buchner to synthesize some biologically interesting pyrazolines derivatives.1 The main characteristic feature of diazo esters, which is common to all aliphatic diazo compounds, is that their reactions can proceed with either retention or elimination of the diazo fragment. The 1,3-dipolar cycloaddition of diazo esters to compounds containing multiple bonds is the first step of these transformations.5,6 The ensuing reactions with elimination of the nitrogen molecule are primarily characterized by generation of carbenes, their complexes with transition metals, cationoid reagents, and biradicals.1,6-8
In the case of alkenes and their derivatives, these reactions lead to the formation of 1- or 2-pyrazolines,5 which are useful intermediates for the synthesis of various classes of compounds9 including those possessing biological activity.10 However, in the most cases the reactions of diazo carbonyl compounds with alkenes require long reaction times, elevated temperatures, or use of alkenes with electron-withdrawing substituents or a reactive strained double bond.
Furthermore, 4-chromanone derivatives are used as versatile intermediates for the synthesis of many natural products such as brazilin, hematoxylin, ripariochromene, clausenin, calonlide (A) and inophyllum (B).11,12 Chromanone heterocycles have also been paid much attention owing to their important pharmacological proprieties.13
We have examined previously the reactivity of mono- and disubstituted diazoalkanes such as ethyl diazoacetate and diphenyldiazomethane towards α-methylene-γ-butyrolactones and itaconic anhydride.14,15
To the best of our knowledge, there is only one report on the cycloaddition of a diazo compounds to trisubstituted olefins, where the dipolarophiles have the reactive double bond conjugated with an aryl and a carbonyl groups.16 Our group has also examined the reactivity of (E)-3-arylidenechroman-4-ones towards isoquinoline Reissert salts.17 In continuation of our research interest in this field, we present in this contribution the first examples on the regioselective reaction of ethyl diazoacetate 2 with (E)-3-arylidenechroman-4-ones 1a–e. The products 3a–e obtained thus were transformed by action of NBS to ethyl 3-bromo-4’-oxo-4-aryl-spiro[chromanone-3’,5-pyrazole]-3-carboxylate derivatives 4a–e, which, on heating, the extrude straightforwardly N2 with formation of cyclopropylcarboxylates 5a–e. In order to elucidate effect of the p-substituent on the 3(1)-aryl group of 1 on the outcome of the reaction, we have systematically varied the electronic propensities of the substituent.
RESULTS AND DISCUSSION
The (E)-3-arylidenechroman-4-ones 1a–e have been prepared by the acid-catalyzed condensation of 4-chromanones with various benzaldehydes. As confirmed by NMR spectroscopy, these starting materials had an E-configuration in accordance with the literature.18-25 The 1,3-dipolar cycloaddition of 1a–e was carried out in refluxing toluene during 48 h (Scheme 1).
The dipolarophiles 1a–e reacted with the commercially available ethyl diazoacetate 2 with the exocyclic double bond of the (E)-3-arylidenechroman-4-ones. In each case, only a single spiro-compound ethyl 4’-oxo-4-aryl-spiro[chromanone-3’,5-pyrazole]-3-carboxylate 3a–e was isolated as yellow or colorless solid according to Scheme 1. The electron-rich carbon atom of the diazo compound adds to the unsaturated carbon atom β to carbonyl group. It is thus reasonable to assume that preferential approach of the dipole 2 takes place from the less-hindered diastereotopic side of the dipolarophiles 1a–e. This reaction involves initial formation of five-membered spiranic ∆1-pyrazoline that undergo a prototropic rearrangement to form the thermodynamically more stable Δ2-pyrazolines, ethyl 4’-oxo-4-aryl-spiro[chromanone-3’,5-pyrazole]-3-carboxylates 3a–e. Indeed, the formation of the intermediate five-membered spiranic ∆1-pyrazoline was proven by a 1H NMR study. When dipolarophiles 1a–e and 2 were allowed to react for only 6 h, a mixture of ∆1-pyrazoline and ∆2-pyrazoline was obtained in a 40:60 ratio. After 48 h, the isomerisation of ∆1-pyrazoline into ∆2-pyrazoline was complete. The 1H NMR spectrum of intermediate ∆1-pyrazoline 3a (Ar = Ph) clearly indicates the presence of two doublets around δ = 4.45 and 4.90 ppm, which correspond to protons 3-H and 4-H, respectively (Scheme 1). Furthermore, the magnitude of the vicinal coupling constant (J = 9.0 Hz) between the two protons suggests that they are in cis-configuration. This finding is in line with our previous observations and those reported in the literature.14,15,26
The structure of product 3a–e and the regiochemistry of the cycloaddition, i.e. the nitrogen atom being bonded to the more substituted carbon atom of the unsymmetrical double bond, have been established on the basis of spectroscopic data and X-ray structural analysis of one cycloadduct 3a. The IR spectra of these compounds 3a–e contain absorption bands at 3370 cm-1, 1710 cm-1, 1685 cm-1 and 1575 cm-1 due to NH, ester C=O, ring C=O and C=N stretching vibrations, respectively. The 1H NMR spectra display the expected triplet and quartet pattern due to the OEt group at around δ = 1.22 ppm and δ = 4.17 – 4.21 ppm, with J = 7.2 Hz. The presence of a singlet between 4.70 and 4.81 ppm, assigned to the benzylic proton 4-H, is indicative for the regiochemistry of the [3+2] cycloadition, leading exclusively to 5-spiro-fused 2-pyrazoline derivatives.19,27-30 Two mutually coupled doublets (J = 12.3 Hz), which correspond to the methylene protons 2’-H of the chromanone ring, are observed between δ = 4.03 and 4.18 ppm. Another characteristic in products 3a–e is the presence of a N-H proton signal between 6.57 and 6.68 ppm, exchangeable by D2O. The 13C NMR chemical shifts of the spiro-carbon atom C-5(3’), which is deshielded due to proximity of the nitrogen atom, are observed at the field between 71.56 and 71.86 ppm.17,31 This excludes the presence of the inverse regioisomers 3’a–e. In 3’a–e, one should expected the resonances of theses carbons at a more upfield region than the observed experimental values.16,32 All these data led us to conclude that the structure of adducts 3a–e is as depicted in Scheme 1.
In the cycloadducts 3a–e appear two new chiral centers, i.e. the quaternary spiro-atom C-5(3’) and the C-4 atom of pyrazoline ring (Scheme 1). The relative stereochemistry of these carbon [rel-(4S*,5(3’)R*)] results from preservation of the (E) configuration of the initial olefin. This stereochemistry was encountered in all cycloadducts 3a–e and confirmed to the favored approach of the two reagents. The suggested stereochemistry of the cycloadducts was corroborated by X-ray crystal analysis of product 3a (Figure 1). It should be noted that the phenyl group in compound 3a is trans-arranged with respect to the ring carbonyl group. All metric parameters are normal and deserve no special comment. The observed regio- and stereochemistry are in accordance with previous observations by us and other investigators.14,15,26
In a next step, the ∆2-pyrazolines 3a–e were allowed to react at room temperature (24 h) with NBS in CHCl3 solution in the presence of acetic acid. The formation of the single brominated product suggests that the reaction first involves the protonation of a carbonyl group of NBS, followed by bromination of compounds 3a–e at the 3-position via an ionic process implying lone-pair participation of N-H at the 1-position to afford the corresponding ∆1-pyrazolines ethyl-4-aryl-3-bromo-4’-oxo-spiro- [chromanone-3’,5-pyrazole]-3-carboxylate 4a–e with up to 85% yield (Scheme 2).
In the 1H NMR spectra of these brominated products, the signal of the 4-H proton appear as a singlet in the region between 4.04-4.15 ppm. In the 13C NMR spectra of esters 4a–e, the following signals are observed, δc, ppm: 34.7-36.2 (4-C), 62.7-63.8 (3’,5-C), 64.5-68.7 (3-C), 160.1-165.1 (C=O ester), in addition signals arising from aromatic carbon atoms are present in the spectra (see Experimental Section).
It is known that thermolysis of pyrazolines may lead to formation of spiro-cyclopropane carboxylates derivatives.33-35 Depending on the experimental conditions and the substituents at the bridgehead position, both isomers with endo or exo ester groups can be formed.33 With this objective in mind, we have thermolyzed compounds 4a–e in refluxing toluene for 24 h. Both spectroscopic data as well as elemental analyses were in agreement with formation of the targeted ethyl 1-bromo-3-aryl-4’-oxo-spiro [chromanone-3’,2-cyclopropane]-1-carboxylates 5a–e. The compounds 4a–e released N2 generating biradicals intermediates, which then cyclize leading to the cyclopropanecarboxylates in 65-85% yield (Scheme 3). 1H NMR spectra of 5a–e confirmed the formation of single diastereoisomers during the thermal decomposition of pyrazolines 4a–e.
In the IR spectra an absorption band at around 1710 cm-1 corresponding to the ester group is observed. The 1H NMR spectra of 5a–e contain a singlet in the region between 2.42-2.67 ppm arising from the cyclopropane ring proton (3-H), as well as the expected resonances of aromatic protons and those of the OEt groups. The two diastereotopic methylene protons of the six-membered cycle resonate in the range between 4.20-4.87 ppm and are mutually coupled through a 2J coupling of 12.3 Hz. Compounds 5a–e display the following signals in the 13C NMR spectra, δc, ppm: 32.4-35.9 (3-C), 62.6-63.7 (3’,2-C), 62.3-65.4 (1-C). Signals of the carbon atoms within the cyclopropane ring appeared upfield-shifted relative to those belonging to the pyrazoline ring carbon atoms of the precursor compounds 4.
Furthermore, formation of a spirocyclic cyclopropane derivative has been confirmed by an X-ray crystal structure determination of 5e (Figure 2). Each carbon atom of the cyclopropane ring of 5e is chiral, formally giving rise to several diastereoisomers. However, there is only one molecule of 5e in the asymmetric unit of P-1 triclinic centrosymmetric space group, indicating that only one pair of enantiomers is present in the crystal. These are the 8R, 10R, 11S and 8S, 10S, 11R on C8, C10 and C11 atoms. Such a natural choice of an enantiomeric pair may be attributed to the relatively low molecular energy when the O1, Br and the H atom bound to C10 (see Figure 2) are placed over the same face of the cyclopropane ring, thus reducing the steric hindrances. All metric parameters are normal and deserve no further comment.
CONCLUSION
We have shown that the reaction of ethyl diazoacetate 2 with (E)-3-arylidenechroman-4-ones 1a–e is regio- and stereoselective. Independently of the electronic nature of the p-substituents on the 3(1)-aryl group of dipolarophiles 1a–e, the electron-rich carbon atom of the diazo compound 2 added to the unsaturated carbon atom β to carbonyl group. The observed regioselectivity is thus in accordance with other previous studies of our research groups. Spiro esters 3a–e react under mild conditions with NBS in CHCl3 solution in the presence of acetic acid to afford ethyl 3-bromo-4’-oxo-4-aryl-spiro[chromanone- 3’,5-pyrazole]-3-carboxylates 4a–e. Subsequent thermolysis of 4a–e in refluxing toluene leads under N2-extrusion in a stereoselective manner to the cyclopropanecarboxylate derivatives 5a–e which have an exo-configuration of the ester group. Currently, complementary studies on the cycloaddition of ethyl diazoacetate with (Z)-3-arylidene-2(3H)benzofuranones and 2-arylideneindan-1,3- diones are underway.
EXPERIMENTAL
General Remarks: Reactions were carried out under an atmosphere of dry N2. Solvents were purified by standard methods and freshly distilled under nitrogen and dried before use. Melting points were determined on a Kofler bank. IR spectra were recorded in KBr pellets on a Perkin-Elmer 197 spectrometer; only structurally significant bands are reported. NMR spectra were recorded with a Bruker-Spectrospin AC 300 spectrometer operating at 300 MHz for 1H and 75.5 MHz for 13C using TMS as internal standard (0.00 ppm) in CDCl3 as solvent. Chemical shifts were reported in ppm (δ) downfield from TMS. (E)-3-arylidenechroman-4-ones 1a–e were obtained by aldol condensation of arylaldehydes ArCHO with the respective chromanones according to reported methods.18-25 Ethyl diazoacetate 2 was purchased from Aldrich.
General procedure for the preparation of the cycloadducts (3a–e)
A solution of dipolarophiles 1a–e (1 mmol) and ethyl diazoacetate (1 mmol) 2 in dry toluene (10 mL) was stirred under reflux for 48 h. Then the solvent was evaporated under reduced pressure and the residue was recrystallized from EtOH to afford compounds 3a–e.
Ethyl 4’-oxo-4-phenyl-spiro[chromanone-3’,5-pyrazole]-3-carboxylate 3a
Yield 0.26 g (75%); colorless solid; mp 176 °C; IR (KBr, cm-1): 3290 (N-H), 1710 (C=O ester), 1685 (C=O), 1570 (C=N); 1H NMR (CDCl3): δ 1.20 (t, 3H, J = 7.2 Hz, CH3); 4.03 (d, 1H, J = 12.3 Hz, 2’-H); 4.14 (d, 1H, J = 12.3 Hz, 2’-H); 4.21 (q, 2H, J = 7.2 Hz, CH2-O); 4.81 (s, 1H, 4-H); 6.64 (s, 1H, N-H); 6.91-7.97 (m, aromatic H); 13C NMR (CDCl3): δ 14.0 (CH3); 54.2 (4-C); 61.4 (2’-C); 69.7 (CH2-O); 71.5 (3’,5-C); 117.9-146.6 (aromatic C); 160.8 (C=N); 161.2 (C=O ester); 189.5 (4’-C=O). Anal. Calcd for C20H18N2O4: C, 68.56; H, 5.18; N, 8.00. Found: C, 68.49; H, 5.09; N, 7.94.
Ethyl 4’-oxo-4-(p-tolyl)-spiro[chromanone-3’,5-pyrazole]-3-carboxylate 3b
Yield 0.22 g (60%); yellow solid; mp 178 °C; IR (KBr, cm-1): 3280 (N-H), 1712 (C=O ester), 1686 (C=O), 1550 (C=N); 1H NMR (CDCl3): δ 1.22 (t, 3H, J = 7.2 Hz, CH3); 1.56 (s, 3H, CH3) ; 4.05 (d, 1H, J = 12.3 Hz, 2’-H); 4.15 (d, 1H, J = 12.3 Hz, 2’-H); 4.18 (q, 2H, J = 7.2 Hz, CH2-O); 4.76 (s, 1H, 4-H); 6.57 (s, 1H, N-H) ; 6.92-7.97 (m, aromatic H); 13C NMR (CDCl3): δ 14.4 (CH3); 21.6 (CH3); 54.3 (4-C); 61.7 (2’-C); 70.0 (CH2-O); 71.8 (3’,5-C); 118.2-147.2 (aromatic C); 161.2 (C=N); 161.5 (C=O ester); 190.1 (4’-C=O). Anal. Calcd for C21H20N2O4: C, 69.22; H, 5.53; N, 7.69. Found: C, 69.15; H, 5.43; N, 7.61.
Ethyl 4’-oxo-4-(p-anisyl)-spiro[chromanone-3’,5-pyrazole]-3-carboxylate 3c
Yield 0.31 g (82%); yellow solid; mp 142 °C; IR (KBr, cm-1): 3289 (N-H), 1710 (C=O ester), 1684 (C=O), 1570 (C=N); 1H NMR (CDCl3): δ 1.22 (t, 3H, J = 7.2 Hz, CH3); 4.06 (d, 1H, J = 12.0 Hz, 2’-H); 4.16 (d, 1H, J = 12.0 Hz, 2’-H); 4.17 (q, 2H, J = 7.2 Hz, CH2-O); 3.86 (s, 3H, OCH3) ; 4.70 (s, 1H, 4-H); 6.50 (s, 1H, N-H) ; 6.95-8.03 (m, aromatic H); 13C NMR (CDCl3): δ 14.0 (CH3); 54.2 (4-C); 55.2 (OCH3); 61.4 (2’-C); 69.7 (CH2-O); 71.6 (3’,5-C); 117.9-146.4 (aromatic C); 160.8 (C=N); 161.2 (C=O ester); 189.5 (4’-C=O). Anal. Calcd for C21H20N2O5: C, 66.31; H, 5.30; N, 7.36. Found: C, 66.19; H, 5.35; N, 7.29.
Ethyl 4’-oxo-4-(p-methylthiophenyl)-spiro[chromanone-3’,5-pyrazole]-3-carboxylate 3d
Yield 0.33 g (85%); colorless solid; mp 168 °C; IR (KBr, cm-1): 3270 (N-H), 1711 (C=O ester), 1684 (C=O), 1560 (C=N); 1H NMR (CDCl3): δ 1.22 (t, 3H, J = 7.2 Hz, CH3); 2.48 (s, 3H, SCH3); 4.06 (d, 1H, J = 12.0 Hz, 2’-H); 4.18 (d, 1H, J = 12.0 Hz, 2’-H); 4.21 (q, 2H, J = 7.2 Hz, CH2-O); 4.73 (s, 1H, 4-H); 6.64 (s, 1H, N-H) ; 6.91-7.93 (m, aromatic H); 13C NMR (CDCl3): δ 14.0 (CH3); 15.4 (SCH3); 53.8 (4-C); 61.4 (2’-C); 69.5 (CH2-O); 71.5 (3’,5-C); 117.7-146.4 (aromatic C); 160.7 (C=N); 161.1 (C=O ester); 189.6 (4’-C=O). Anal. Calcd for C21H20N2O4S: C, 63.62; H, 5.08; N, 7.07. Found: C, 63.51; H, 5.02; N, 7.01.
Ethyl 4’-oxo-4-(p-chlorophenyl)-spiro[chromanone-3’,5-pyrazole]-3-carboxylate 3e
Yield 0.33 g (87%); colorless solid; mp 166 °C; IR (KBr, cm-1): 3280 (N-H), 1710 (C=O ester), 1683 (C=O), 1570 (C=N); 1H NMR (CDCl3): δ 1.24 (t, 3H, J = 7.2 Hz, CH3); 4.03 (d, 1H, J = 12.3 Hz, 2’-H); 4.16 (d, 1H, J = 12.3 Hz, 2’-H); 4.19 (q, 2H, J = 7.2 Hz, CH2-O); 4.74 (s, 1H, 4-H); 6.68 (s, 1H, N-H); 6.92-7.96 (m, aromatic H); 13C NMR (CDCl3): δ 14.1 (CH3); 53.7 (4-C); 61.5 (2’-C); 69.2 (CH2-O); 71.6 (3’,5-C); 117.8-146.2 (aromatic C); 160.2 (C=N); 161.0 (C=O ester); 189.3 (4’-C=O). Anal. Calcd for C20H17ClN2O4: C, 62.42; H, 4.45; N, 7.28. Found: C, 62.30; H, 4.38; N, 7.23.
General procedure for the preparation of compounds (4a–e)
N-Bromosuccinimide, (1.1 mmol), was added at room temperature to a solution of 1 mmol of 3a–e in a mixture of 20 mL of anhydrous CHCl3 and 10 mL of acetic acid. When the reaction was complete (TLC), the mixture was stirred for an additional 40 min and washed with water, a 5% aqueous solution of sodium hydrogen carbonate, and water again. The organic phase was dried over MgSO4, evaporated to a volume of ca. 5 mL, diluted with 10 mL of EtOH, evaporated again, and the precipitate was filtered.
Ethyl 3-bromo-4’-oxo-4-phenyl-spiro[chromanone-3’,5-pyrazole]-3-carboxylate 4a
Yield 0.30 g (70%); colorless solid; mp 154 °C; IR (KBr, cm-1): 800 (C-Br), 1300 (C-N), 1710 (C=O ester); 1684 (C=O); 1H NMR (CDCl3): δ 0.91 (t, 3H, J = 7.3 Hz, CH3); 4.15 (s, 1H, 4-H); 4.23 (q, 2H, J = 7.3 Hz, CH2-O); 4.53 (d, 1H, J = 12.3 Hz, 2’-H); 4.58 (d, 1H, J = 12.3 Hz, 2’-H); 6.92-8.04 (m, aromatic H); 13C NMR (CDCl3): δ 12.9 (CH3); 35.6 (4-C); 36.9 (2’-C); 50.1 (CH2-O); 63.8 (3’,5-C); 68.7 (3-C); 126.0-155.3 (aromatic C); 160.1 (C=O ester); 188.4 (4’-C=O). Anal. Calcd for C20H17BrN2O4: C, 55.96; H, 3.99; N, 6.53. Found: C, 55.89; H, 3.90; N, 6.46.
Ethyl 3-bromo-4’-oxo-4-(p-tolyl)-spiro[chromanone-3’,5-pyrazole]-3-carboxylate 4b
Yield 0.24 g (54%); yellow solid; mp 160 °C; IR (KBr, cm-1): 815 (C-Br), 1310 (C-N), 1711 (C=O ester); 1685 (C=O); 1H NMR (CDCl3): δ 1.20 (t, 3H, J = 7.3 Hz, CH3); 1.54 (s, 3H, CH3) ; 4.04 (s, 1H, 4-H); 4.20 (q, 2H, J = 7.3 Hz, CH2-O); 4.72 (d, 1H, J = 12.3 Hz, 2’-H); 4.83 (d, 1H, J = 12.3 Hz, 2’-H); 6.98-8.06 (m, aromatic H); 13C NMR (CDCl3): δ 13.9 (CH3); 20.5 (CH3); 36.2 (4-C); 37.4 (2’-C); 51.2 (CH2-O); 62.7 (3’,5-C); 64.5 (3-C); 117.3-145.2 (aromatic C); 162.4 (C=O ester); 189.4 (4’-C=O). Anal. Calcd for C21H19BrN2O4: C, 56.90; H, 4.32; N, 6.32. Found: C, 56.81; H, 4.22; N, 6.24.
Ethyl 3-bromo-4’-oxo-4-(p-anisyl)-spiro[chromanone-3’,5-pyrazole]-3-carboxylate 4c
Yield 0.39 g (80%); yellow solid; mp 135 °C; IR (KBr, cm-1): 820 (C-Br), 1315 (C-N), 1712 (C=O ester); 1684 (C=O); 1H NMR (CDCl3): δ 1.18 (t, 3H, J = 7.3 Hz, CH3); 3.86 (s, 3H, OCH3); 4.11 (s, 1H, 4-H); 4.21 (q, 2H, J = 7.3 Hz, CH2-O); 4.65 (d, 1H, J = 12.3 Hz, 2’-H); 4.80 (d, 1H, J = 12.3 Hz, 2’-H); 7.03-8.05 (m, aromatic H); 13C NMR (CDCl3): δ 13.6 (CH3); 34.7 (4-C); 38.3 (2’-C); 44.7 (CH2-O); 55.1 (CH3-O); 65.5 (3’,5-C); 66.4 (3-C); 118.2-148.3 (aromatic C); 163.1 (C=O ester); 187.5 (4’-C=O). Anal. Calcd for C21H19BrN2O5: C, 54.92; H, 4.17; N, 6.10. Found: C, 54.88; H, 4.21; N, 6.17.
Ethyl 3-bromo-4’-oxo-4-(p-methylthiophenyl)-spiro[chromanone-3’,5-pyrazole]-3-carboxylate 4d
Yield 0.35 g (75%); colorless solid; mp 150 °C; IR (KBr, cm-1): 810 (C-Br), 1300 (C-N), 1710 (C=O ester); 1683 (C=O); 1H NMR (CDCl3): δ 1.16 (t, 3H, J = 7.3 Hz, CH3); 2.41 (s, 3H, SCH3); 4.09 (s, 1H, 4-H); 4.22 (q, 2H, J = 7.3 Hz, CH2-O); 4.67 (d, 1H, J = 12.0 Hz, 2’-H); 4.82 (d, 1H, J = 12.0 Hz, 2’-H); 6.98-8.02 (m, aromatic H); 13C NMR (CDCl3): δ 12.7 (CH3); 14.2 (SCH3); 35.3 (4-C); 37.3 (2’-C); 50.2 (CH2-O); 64.6 (3’,5-C); 65.8 (3-C) ; 125.3-145.5 (aromatic C); 165.0 (C=O ester); 187.5 (4’-C=O). Anal. Calcd for C21H19BrN2O4S: C, 53.06; H, 4.03; N, 5.89. Found: C, 53.00; H, 3.98; N, 5.81.
Ethyl 3-bromo-4’-oxo-4-(p-chlorophenyl)-spiro[chromanone-3’,5-pyrazole]-3-carboxylate 4e
Yield 0.39 g (85%); colorless solid; mp 140 °C; IR (KBr, cm-1): 813 (C-Br), 1311 (C-N), 1709 (C=O ester); 1685 (C=O); 1H NMR (CDCl3): δ 1.23 (t, 3H, J = 7.3 Hz, CH3); 4.08 (s, 1H, 4-H); 4.20 (q, 2H, J = 7.3 Hz, CH2-O); 4.78 (d, 1H, J = 12.3 Hz, 2’-H); 4.87 (d, 1H, J = 12.3 Hz, 2’-H); 7.03-8.04 (m, aromatic H); 13C NMR (CDCl3): δ 13.8 (CH3); 35.9 (4-C); 38.5 (2’-C); 41.2 (CH2-O); 63.1 (3’,5-C); 66.8 (3-C); 118.2-136.6 (aromatic C); 165.1 (C=O ester); 186.1 (4’-C=O). Anal. Calcd for C20H16BrClN2O4: C, 51.80; H, 3.48; N, 6.04. Found: C, 51.71; H, 3.42; N, 5.90.
General procedure for the preparation of the ethyl cyclopropanecarboxylates (5a–e)
A solution of 0.3 mmol of 4a–e in 4 mL of toluene was refluxed for 24 h (TLC), until evolution of nitrogen stopped. The solvent was then evaporated, 2 mL of EtOH were added to the residue, and the formed precipitates were filtered and recrystallized from EtOH.
Ethyl 1-bromo-3-phenyl-4’-oxo-spiro[chromanone-3’,2-cyclopropane]-1-carboxylate 5a
Yield 0.10 g (80%); colorless solid; mp 110 °C; IR (KBr, cm-1): 805 (C-Br), 1710 (C=O ester); 1686 (C=O), 1H NMR (CDCl3): δ 0.64 (t, 3H, J = 7.3 Hz, CH3); 2.67 (s, 1H, 3-H); 3.78 (q, 2H, J = 7.3 Hz, CH2-O); 4.20 (d, 1H, J = 12.3 Hz, 2’-H); 4.57 (d, 1H, J = 12.3 Hz, 2’-H); 6.79-8.02 (m, aromatic H); 13C NMR (CDCl3): δ 12.7 (CH3); 34.5 (3-C); 36.7 (2’-C); 49.8 (CH2-O); 63.7 (3’,2-C); 65.4 (1-C); 117.6-146.4 (aromatic C); 160.0 (C=O ester); 187.3 (4’-C=O). Anal. Calcd for C20H17BrO4: C, 59.87; H, 4.27. Found: C, 59.80; H, 4.20.
Ethyl 1-bromo-3-(p-tolyl)-4’-oxo-spiro[chromanone-3’,2-cyclopropane]-1-carboxylate 5b
Yield 0.09 g (77%); yellow solid; mp 120 °C; IR (KBr, cm-1): 810 (C-Br), 1712 (C=O ester); 1684 (C=O); 1H NMR (CDCl3): δ 0.73 (t, 3H, J = 7.3 Hz, CH3); 1.56 (s, 3H, CH3); 2.56 (s, 1H, 3-H); 3.73 (q, 2H, J = 7.3 Hz, CH2-O); 4.41 (d, 1H, J = 12.3 Hz, 2’-H); 4.80 (d, 1H, J = 12.3 Hz, 2’-H); 6.83-7.93 (m, aromatic H); 13C NMR (CDCl3): δ 13.6 (CH3); 20.4 (CH3); 35.9 (3-C); 37.2 (2’-C); 50.9 (CH2-O); 62.3 (1-C); 62.6 (3’,2-C); 118.1-146.9 (aromatic C); 161.2 (C=O ester); 189.4 (4’-C=O). Anal. Calcd for C21H19BrO4: C, 60.74; H, 4.61. Found: C, 60.61; H, 4.75.
Ethyl 1-bromo-3-(p-anisyl)-4’-oxo-spiro[chromanone-3’,2-cyclopropane]-1-carboxylate 5c
Yield 0.08 g (65%); yellow solid; mp 95 °C; IR (KBr, cm-1): 815 (C-Br), 1711 (C=O ester); 1684 (C=O); 1H NMR (CDCl3): δ 0.78 (t, 3H, J = 7.3 Hz, CH3); 2.60 (s, 1H, 3-H); 3.75 (q, 2H, J = 7.3 Hz, CH2-O); 3.83 (s, 3H, OCH3); 4.62 (d, 1H, J = 12.3 Hz, 2’-H); 4.78 (d, 1H, J = 12.3 Hz, 2’-H); 6.91-7.78 (m, aromatic H); 13C NMR (CDCl3): δ 13.4 (CH3); 32.7 (3-C); 37.8 (2’-C); 43.6 (CH2-O); 55.1 (CH3-O); 64.5 (3’,2-C); 64.7 (1-C); 117.4-146.3 (aromatic C); 160.9 (C=O ester); 189.3 (4’-C=O). Anal. Calcd for C21H19BrO5: C, 58.48; H, 4.44. Found: C, 58.40; H, 4.53.
Ethyl 1-bromo-3-(p-methylthiophenyl)-4’-oxo-spiro[chromanone-3’,2-cyclopropane]-1-carboxylate 5d
Yield 0.11 g (85%); colorless solid; mp 114 °C; IR (KBr, cm-1): 800 (C-Br), 1711 (C=O ester); 1684 (C=O); 1H NMR (CDCl3): δ 0.67 (t, 3H, J = 7.3 Hz, CH3); 2.35 (s, 3H, SCH3); 2.64 (s, 1H, 3-H); 3.77 (q, 2H, J = 7.3 Hz, CH2-O); 4.46 (d, 1H, J = 12.3 Hz, 2’-H); 4.80 (d, 1H, J = 12.3 Hz, 2’-H); 6.95-7.75 (m, aromatic H); 13C NMR (CDCl3): δ 12.4 (CH3); 14.1 (SCH3); 34.9 (3-C); 37.1 (2’-C); 49.7 (CH2-O); 63.5 (3’,2-C); 64.6 (1-C); 117.5-144.2 (aromatic C); 164.8 (C=O ester); 185.6 (4’-C=O). Anal. Calcd for C21H19BrO4S: C, 56.38; H, 4.28. Found: C, 56.30; H, 4.18.
Ethyl 1-bromo-3-(p-chlorophenyl)-4’-oxo-spiro[chromanone-3’,2-cyclopropane]-1-carboxylate 5e
Yield 0.10 g (80%); colorless solid; mp 112 °C; IR (KBr, cm-1): 812 (C-Br), 1710 (C=O ester); 1H NMR (CDCl3): δ 0.81 (t, 3H, J = 7.3 Hz, CH3); 2.42 (s, 1H, 3-H); 3.83 (q, 2H, J = 7.3 Hz, CH2-O); 4.48 (d, 1H, J = 12.3 Hz, 2’-H); 4.87 (d, 1H, J = 12.3 Hz, 2’-H); 6.81-7.85 (m, aromatic H); 13C NMR (CDCl3): δ 13.5 (CH3); 35.4 (3-C); 37.9 (2’-C); 40.8 (CH2-O); 62.8 (3’,2-C); 65.4 (1-C); 117.8-135.4 (aromatic C); 164.7 (C=O ester); 185.42 (4’-C=O). Anal. Calcd for C20H16BrClO4: C, 55.13; H, 3.70. Found: C, 55.08; H, 3.65.
X-Ray structure analyses of 3a and 5e.
Intensity data for 3a were collected on a Brucker AXS diffractometer with MoKα radiation. The crystal structure was solved by direct methods (SHELXS)36 and refined (SHELXL)36 with full-matrix least squares on F2. X-Ray intensity data for 5e were collected at 115 K with MoKα radiation on a Nonius Kappa Apex II diffractometer. The crystal structure was solved by direct methods37 and refined with full-matrix least squares on F2.37,38 Anisotropic thermal parameters were applied for non-hydrogen atoms. All H atoms were placed in calculated positions and included in final refinements with isotropic temperature factors in a riding model on the carbon atoms bearing them.
Crystal data for 3a: C20H18N2O4, M = 350.36, monoclinic, space group P21/c, a = 7.1947(3) Å, b = 17.1869(7) Å, c = 13.6470(7) Å, β = 90.803(4)°, V = 1687.34(13) Å3, Z = 4, Dc = 1.379 Mg/m3, λ(Mo-Kα) = 0.71073 Å, F(000) = 736, µ(Mo-Kα) = 0.097 mm-1, T = 173(2) K. 11957 reflections collected, 3312 unique (Rint = 0.0331) and 2253 with I>2σ(I). Final residuals ρmax = 0.211, ρmin = -0.231 e-/Å3. Final agreement factors: R(F) = 0.0343 and 0.0572, wR(F2) = 0.0620 and 0.0638 for I>2σ(I) and all data, respectively, GOF = 1.067.
Crystal data for 5e: C20H16BrClO4 : M = 435.69, triclinic, space group P-1, a = 6.5526(2) Å, b = 10.1940(4) Å, c = 13.3432(6) Å, α = 86.801(2), β = 85.906(2), γ = 86.323(2)°, V = 886.01(6) Å3, Z = 2, λ(Mo-Kα) = 0.71073 Å, F(000) = 440, µ(Mo-Kα) = 2.494 mm-1, Dcalc = 1.633 g/cm3, T = 115(2) K. 7650 reflections collected, 4074 unique (Rint = 0.0252) and 3708 with I>2σ(I). Final agreement factors: R(F) = 0.0330 and 0.0395 and wR(F2) = 0.0729 and 0.777 for I>2σ (I) and all data, respectively, GOF = 1.120. Final residuals ρmax = 0.416, ρmin = -0.366 e-/Å3.
Crystallographic data for the structures of 3a and 5e have been deposited with the Cambridge Crystallographic Data Centre as supplementary publication number 854948 and 854949. Copy of these data can be obtained, free of charge, on application to CCDC, 12 Union Road, Cambridge CB2 IEZ, UK, fax: 144-(0)1223-336033 or e-mail: deposit@ccdc.cam.ac.uk.
References
1. M. Regitz and G. Maas, Diazo Compounds: Properties and Synthesis, Academic Press, Orlando, Florida, 1986, 65; A. Padwa and M. D. Weingarten, Chem. Rev., 1996, 96, 223; CrossRef M. P. Doyle, M. A. McKervey, and T. Ye, Modern Catalytic Methods for Organic Synthesis with Diazo Compounds, John Wiley and Sons: New York, 1998; H. M. L. Davies and R. E. J. Beckwith, Chem. Rev., 2003, 103, 2861; CrossRef Z. Zhang and J. Wang, Tetrahedron, 2008, 64, 6577. CrossRef
2. Q. R. Bartz, C. C. Elder, R. P. Frohardt, S. A. Fusari, T. H. Haskell, D. W. Johannessen, and A. Ryder, Nature, 1954, 173, 72. CrossRef
3. S. Ito, T. Matsuya, S. Omura, M. Otani, A. Nakagawa, H. Takeshima, Y. Iwai, M. Ohtani, and T. Hata, J. Antibiot., 1970, 23, 315; S. J. Gould, N. Tamayo, C. R. Melville, and M. C. Cone, J. Am. Chem. Soc., 1994, 116, 2207; CrossRef S. Mithani, G. Weeratunga, N. J. Taylor, and G. I. Dmitrienko, J. Am. Chem. Soc., 1994, 116, 2209. CrossRef
4. H. He, W. D. Ding, V. S. Bernan, A. D. Richardson, C. M. Ireland, M. Greenstein, G. A. Ellestad, and G. T. Carter, J. Am. Chem. Soc., 2001, 123, 5362. CrossRef
5. M. Regitz and H. Heydt, 1,3-Cycloaddition Chemistry, ed. by A. Padwa, John Wiley and Sons, New York, 1984, 1, 394.
6. E. A. Shapiro, A. B. Dyatkin, and O. M. Nefedov, Diazoesters, Nauka, Moscow, 1992, 148.
7. G. Maas, Topics in Current Chemistry, Springer-Verlag, Berlin, 1987, 137, 75.
8. M. P. Doyle, Chem. Rev., 1986, 86, 919. CrossRef
9. G. Maas, in The Chemistry of Heterocyclic Compounds ; Synthetic Applications of 1,3-Dipolar Cycloaddition Chemistry toward Heterocycles and Natural Products, ed. by A. Padwa and W. H. Pearson, John Wiley and Sons, New York, 2002, 59, 539.
10. T. Kano, T. Hashimoto, and K. Maruoka, J. Am. Chem. Soc., 2006, 128, 2174. CrossRef
11. E. E. Schweizer and D. Meeder-Nycz, in Chromenes, Chromanones, and Chromones, ed. by G. P. Ellis, John Wiley and Sons, New York, 1977, 70; I. M. Lockhart, in Chromenes, Chromanones, and Chromones, ed. by G. P. Ellis, John Wiley and Sons, New York, 1977, 301.
12. B. Chenera, M. L. West, J. A. Finkelstein, and G. B. J. Dreyer, J. Org. Chem., 1993, 58, 5605. CrossRef
13. A. F. Crowther, R. Howe, B. J. McLoughlin, K. B. Mallion, B. S. Rao, L. H. Smith, and R. W. Turner, J. Med. Chem., 1972, 15, 260. CrossRef
14. C. Roussel, K. Ciamala, J. Vebrel, J. M. Melot, J. Vebrel, and C. Riche, J. Chem. Res. (S), 2002, 449.
15. C. Roussel, K. Ciamala, J. Vebrel, M. Knorr, and M. M. Kubicki, Heterocycles, 2007, 71, 1517. CrossRef
16. A. P. Molchanov, V. S. Korotkov, and R. R. Kostikov, Russ. J. Org. Chem., 2004, 40, 470. CrossRef
17. M. Askri, M. Rammah, K. Monnier-Jobé, K. Ciamala, M. Knorr, and C. Strohmann, Lett. Org. Chem., 2007, 4, 146.
18. B. Bennet, J. A. Donnelly, D. C. Meaney, and P. O. Boyle, J. Chem. Soc., Perkin Trans 1, 1972, 1554. CrossRef
19. R. Raghunathan, M. Shanmugasundaram, S. Bhanumathi, and E. J. Padma Malar, Heteroatom Chem., 1998, 9, 327. CrossRef
20. N. K. Sangwan and S. N. Rastogi, Indian J. Chem., 1981, 20B, 135.
21. G. Wagner, C. Garbe, H. Vieweg, M. Brunn, and A. Dittrich, Pharmazie, 1979, 34, 55.
22. G. C. Wright, J. Heterocycl. Chem., 1976, 13, 1177. CrossRef
23. J. N. Chatterjea, S. C. L. Shaw, P. K. Lal, and R. P. Singh, J. Indian Chem. Soc., 1979, 56, 1006.
24. D. Basavaiah, M. Bakthadoss, and S. Pandiaraju, J. Chem. Soc., Chem. Commun., 1998, 16, 1639.
25. S. Manikandan, M. Shanmugasundaram, R. Raghunathan, and E. J. Padmar Malar, Heterocycles, 2000, 53, 579. CrossRef
26. V. M. Berestovitskaya, N. A. Anisimova, A. T. Gubaidullin, I. A. Litvinov, G. A. Berkova, and N. G. Makarova, Russ. J. General Chem., 2009, 79, 1446. CrossRef
27. R. Huisgen, M. Seidel, G. Wallbillich, and H. Knupfer, Tetrahedron, 1962, 17, 3. CrossRef
28. R. Huisgen, Bull. Soc. Chim. Fr., 1965, 3431.
29. G. Bianchi, R. Gandolfi, and C. De Micheli, J. Chem. Res. (S), 6 and (M), 1981, 135.
30. K. N. Houk and Y. Yamaguchi, Theory of 1,3-Dipolar Cycloadditions in 1,3-Dipolar Cycloaddition Chemistry, ed. by A. Padwa, John Wiley and Sons, 1984, 2, 407.
31. R. Fihi, K. Ciamala, J. Vebrel, and N. Rodier, Bull. Soc. Chim. Belg., 1995, 104, 55. CrossRef
32. M. M. Rammah, M. Othman, K. Ciamala, C. Strohmann, and M. B. Rammah, Tetrahedron, 2008, 64, 3505. CrossRef
33. S. A. Molchanov, A. V. Stepakov, and R. R. Kostikov, Russ. J. Org. Chem., 2000, 36, 899.
34. G. Mass, in Methods of Organic Chemistry, ed. by A. de Meijere, Georg Thieme, 1997, 17a, 405.
35. R. J. Buschby, Methoden der Organischen Chemie (Houben-Weyl), ed. by A. de Meijere, Stuttgart: Georg Thieme 4th Ed. 1997, E17b, 1059.
36. SIR92 program, A. Altomare, G. Cascarano, C. Giacovazzo, and A. Guagliardi, J. Appl. Crystallogr., 1993, 32, 115. CrossRef
37. G. M. Sheldrick, Acta Cryst., 2008, A64, 112.
38. SUPERFLIP program, L. Palatinus and G. Chapuis, J. Appl. Crystallogr., 2007, 40, 786. CrossRef