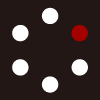
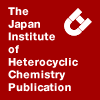
HETEROCYCLES
An International Journal for Reviews and Communications in Heterocyclic ChemistryWeb Edition ISSN: 1881-0942
Published online by The Japan Institute of Heterocyclic Chemistry
e-Journal
Full Text HTML
Received, 25th December, 2011, Accepted, 8th February, 2012, Published online, 14th February, 2012.
DOI: 10.3987/COM-11-12416
■ New Route to Novel Polysubstituted Quinolines Starting with Eugenol, the Main Constituent Ocimum sanctum L. Oil
Nguyen Huu Dinh,* LeVan Co, Nguyen Manh Tuan, Le Thi Hong Hai, and Luc Van Meervelt
Department of Chemistry, Hanoi National University of Education, 136 Xuanthuy Hanoi, Vietnam
Abstract
An efficient and simple method has been reported for the synthesis of (6-hydroxy-3-sulfoquinolin-7-yloxy)acetic acid (Q1) starting with eugenol, the main constituent of Ocimum sanctum L. oil. The reaction pathway from a quinone-aci compound to the quinoline compound Q1 was proposed on the basis of 1H NMR method. Q1 was used as a key compound for further transformation to 9 novel polysubstituted quinolines. The structure of the synthesized compounds was characterized by spectroscopic methods.INTRODUCTION
Quinolines are an important class of heterocyclic compounds. The quinoline skeleton has been used as the basis for the design of many synthetic antimalarial,1,2 antibacterial, antifungal,3,4 anti-tuberculosis,5-7 anticancer8 compounds. Almost all these compound are polysubstituted quinolines, which have been synthesized starting from industrial petrochemical products. Moreover classical methods for quinoline synthesis often do not allow for adequate diversity and substitution on the quinoline ring system. Therefore, having new synthetic methods for preparing quinoline derivatives including a clean, efficient, large-scale and cheap technology is still needed to obtain useful poly-functionalized quinolines.
Some time ago, we had focused our attention to several main components of vegetable essential oils that, owing to their structure, could act as good substrate in order to prepare heterocyclic compounds. For example, some furoxans and metallacyclic complexes were prepared from safrole (in sassafras oil)9,10 and from eugenol (in clove oil),11,12 thiazolidinones and indoles were synthesized from anethole (in star anise oil).13
In this context, now we present a novel route for synthesis of polysubstituted quinolines from eugenol (l-hydroxy-2-methoxy-4-allylbenzene), the main constituent of Ocimum sanctum L. oil (a cheap natural source for commercial extraction of eugenol).14
RESULTS AND DISCUSSION
The route for the preparation of the key compound to polysubstituted quinolines is described in Figure 1. Eugenoxyacetic acid, 2-methoxy-4-(2-propenyl)phenoxyacetic acid, is known to be a beneficial food additive. It is easily prepared by reaction of eugenol with monochloroacetic acid.15 Treating with excess nitric acid in acetic acid eugenoxyacetic acid underwent an unexpected ether cleavage, a normal nitration, and then an unexpected electrophilic addition to the double bond of the side chain that led to the formation of a reactive quinone-aci compound A.16 Reduction of the quinone-aci compound afforded (6-hydroxy-3-sulfoquinolin-7-yloxy)acetic acid (Q1).
There are many methods of synthesis of quinoline ring. The classical syntheses such as Skraup, Doebner-von Miller, Friedländer, Pfitzinger, Conrad-Limpach, Combes are well-known. The new approaches diverse range of efficient quinoline synthesis were reviewed by V. V. Kouznetsov et al.,17 however there is no similar to the method described in Figure 1. Therefore the accurately structural determination of the quinoline compound Q1 is necessary.
In the 1H NMR of Q1, two doublets at 8.80 and 8.24 ppm (J = 2 Hz) are assigned to H2 and H4 respectively, two singlets at 7.28 and 7.25 ppm are assigned to H5 and H8, a singlet at 4.81 ppm (2H) is associated with methylene group (H11). In the 13C NMR of Q1 there are 9 signals associated with 9 aromatic carbon atoms (C2 – C10 in Figure 2), a signal of CH2 group (C11, at 66.30 ppm), and a signal of C=O group (C12 at 171.42 ppm). HSQC spectrum of Q1 allows to assign the signals of C2, C4 and C11. In HMBC spectrum of Q1 two cross peaks of H11 showed signal of C7 at 152.16 ppm, signal of C12 at 171.41 ppm.
In Figure 2 the cross peak a of C7 indicates that singlet at 7.28 ppm belongs to H5, as a result singlet at 7.25 ppm belongs to H8. Two cross peaks b and c of H5 show signals of C9 and C4, two cross peaks d and e of H8 show signals of C6 and C10, respectively. The cross peaks h, i and k show signals of C2, C9 and C5 respectively. The cross peaks l, m and n show signals of C9, C3 and C4 respectively.
These NMR data are in good agreement with the quinoline structure of Q1 but do not show the presence of SO3-, an unexpected group at 3-position of Q1. The mass spectra in the positive mode of Q1 showed pseudomolecular ions (M+H)+ as following, (au/%): 300/100, 301/12 (13C), 302/5 (34S), and negative mode mass spectra of Q1 showed pseudomolecular ions (M-H)- as following, (au/%): 298/100, 299/12 (13C), 300/5 (34S). This is corresponding to molecular mass of Q1 (299 au) which containing one S atom.
In order to finally determine the structure of Q1, the single-crystal X-ray diffraction for it was analyzed. The results (Figure 3) show the presence of sulfonate at 3-position and Q1 exists in a zwitterionic form (NH+ and SO3-).
This brings up the question: how was the quinone-aci compound A converted into the quinoline compound Q1? In order to answer this question, reaction process of the conversion from A to Q1 in sample tube was monitored with 1H NMR. The representative spectra and the important remarks are presented in Figure 4.
On the basis of data in Figure 4, we suggest a reaction pathway for the conversion from A to Q1 as in Figure 5.
When treating with Na2S2O4/OH- during 6 hours the phenol-nitro compoud A1 (a tautomer of the quinone-aci compound A) was reduced into amino compound A2 (Figure 5). After the addition of trifloroacetic acid into the reaction mixture the –CH=NOO- group of A2 was transformed into –CH=O group to give A3 (Nef reaction18). The condensation of –CH=O and H2N- groups caused the cyclization to form A4, which was oxidized by air oxygen to A5. The addition of NaHSO3 into carbonyl group of A5 followed by the elimination of H2O afforded the target compound Q1. In fact the stage from A to A2 (alkaline stage) took place for 6 hours while the stage from A2 to Q1 (from the addition of trifloroacetic acid to the appearance of a significant amount of the precipitate, acidic stage) took place for more than 72 hours. The acidic stage can be accelerated by addition sulfuric acid and heating at 90 oC.
The other polysubstituted quinolines were prepared starting from Q1 as shown below:
i) Esterification of Q1 with acetic anhydride afforded (6-acetoxy-3-sulfoquinolin-7-yloxy)acetic acid (Q2). In the NMR spectra of Q2, for CH3C=O group: proton signal appeared at 2.38 ppm, carbon signals appeared at 168.41 and 20.33 ppm. In the HMBC of Q2 signal of proton H2 (9.32 d; J 1.5) has not cross peak with signal of the carbonyl carbon (168.41) indicating that the CH3C=O group does not attached to N of quinoline ring.
ii) Esterification of Q1 with methanol gave methyl ester Q3, which was transformed into acylhydrazine Q4 (Experimental and Table 1).
iii) Nitration of Q1 leaded to (5-nitro-6-hydroxy-3-sulfoquinolin-7-yloxy)acetic acid (Q5), which was reduced into amino compound Q6 (Experimental and Table 1). The HSQC and HMBC spectra of Q5 and Q6 show the nitro group and the amino group are attached to C5 of the quinoline ring.
iv) Chlorination of Q1 afforded (5-chloro-6-hydroxy-3-sulfoquinolin-7-yloxy)acetic acid (Q7, Experimental and Table 1). The HMBC spectrum of Q7 shows Cl is attached to C5 of the quinoline ring.
v) Bromination of Q1 afforded (5-bromo-6-hydroxy-3-sulfoquinolin-7-yloxy)acetic acid (Q8), which was transformed into methyl ester Q9 and then into acylhydrazine Q10 (Experimental and Table 1). The HMBC spectra of Q8 and Q9 show Br is attached to C5 of the quinoline ring.
The structure of Q1 - Q10 was established by IR, NMR, and ESI MS methods. The assignment of 1H NMR and 13C NMR signals (Tables 1, 2) of the reported compounds was based on their chemical shift, spin-spin splitting patterns, and 2D NMR spectra.
The data in tables 1 and 2 are good agreement with the structure of the reported compounds. The molecular weights of Q1 - Q10 determined from ESI MS (see Experimental) correspond with their molecular formula.
In conclusion, a new route for synthesis of polysubstituted quinolines from eugenol, a natural arylolefin, was firstly reported. The reaction pathway from the quinone-aci compound to the quinoline compound was proposed on the basis of 1H NMR method.
EXPERIMENTAL
General
IR spectra were recorded on an IMPACK-410 NICOLET spectrometer in KBr discs at 400–4000 cm-1. ESI mass spectra were recorded using Agilent LC-MSD-Trap-SL series 1100 spectrometer. NMR spectra were recorded on a Bruker AVANCE 500 MHz spectrometer, in d6-DMSO with TMS as the internal standard, at 298–300 K. C, H, and N were analyzed in Analytical Laboratory – Institute of Chemistry of Natural Compounds (in Hanoi). Single-crystal X-ray diffraction is recorded on a Bruker SMART6000 diffractometer (fine-focus sealed tube, CuKα radiation, crossed Göbel mirrors) at 100K. The intensity data were corrected for Lorentz and polarization effects, and for absorption (SADABS).19 The structure was solved by direct methods (SHELXS-97)20 and refined by full-matrix least-squares based on F2 using SHELXL-97.21 Hydrogen atoms were located in the calculated positions.
Preparation
(6-Hydroxy-3-sulfoquinolin-7-yloxy)acetic acid (Q1). 10.92 g (0.04 mol) of the quinon-aci compound A (previously prepared in reference16) was added to a solution of 41.76 g (0.24 mol) Na2S2O4, 40 mL concentrated ammonia and 80 mL H2O during 30 min. The resulting solution was stirred at room temperature for 6 h. and then acetic acid was added (until pH 2). The reaction mixture was kept openly at air at room temperature for 72 h. The crystalline compound obtained was isolated by filtration and washed with water. The crude product was recrystallized from EtOH/water 2:1 by volume to afford the product as light yellow crystals, decomposed at above 250 oC. The yield was 8.13 g (68%). IR (cm-1): 3470, 3234 (OH); 3075, 2930 (C-H); 1680, 1632 (C=O); 1610, 1503 (ring). 1H NMR and 13C NMR see Tables 1 and 2. ESI MS, m/z (au)/relative intensity (%): +MS, 300/100 (M+H+), -MS: 298/100 (M-H+). Anal. Calcd. for C11H9NO7S (M 299.26): C, 44.15; H, 3.03; N, 4.68. Found: C, 44.46; H, 3.26; N, 4.42. For single-crystal X-ray diffraction Q1 was recrystallized from a solution of 1M HCl.
(6-Acetoxy-3-sulfoquinolin-7-yloxy)acetic acid (Q2). 299 mg (1 mmol) Q1 was added to a solution of 0.4 mL H2SO4 in 6 mL Ac2O. The reaction mixture was stirred at 40-50 oC for 2 h. The solid obtained was isolated by filtration and washed with water, EtOH and recrystallized from water. The yield was 188 mg (55%), light yellow crystals, decomposed above 260 oC. IR (cm-1): 3512, 3480 (OH); 3082, 3046, 2931, 2852 (C-H); 1758, 1645 (C=O); 1601, 1503 (ring). 1H NMR and 13C NMR see Tables 1 and 2. Anal. Calcd. for C13H11NO8S (M 341.29): C, 45.75; H, 3.25; N, 4.10. Found: C, 45.48; H, 3.16; N, 4.32.
Methyl (6-hydroxy-3-sulfoquinolin-7-yloxy)acetate (Q3). 150 mg (0.5 mmol) Q1 and 0.01 mL H2SO4 were added to a solution of 2 mL DMSO in 5 mL MeOH. The reaction mixture was refluxed for 12 h. The crystalline compound obtained was isolated by filtration and washed with EtOH, and then EtOAc. The yield was 102 mg (65%), light yellow crystals, decomposed above 265 oC. IR (cm-1): 3449, 3234 (OH); 3060, 2969 (C-H); 1784 (C=O); 1608, 1521 (ring). 1H NMR and 13C NMR see Tables 1 and 2. ESI MS, m/z (au)/relative intensity (%): +MS, 314/4 (M+H+), -MS: 312/100 (M-H+). Anal. Calcd. for C12H11NO7S (M 313.28): C, 46.01; H, 3.54; N, 4.47. Found: C, 46.37; H, 3.28; N, 4.19.
[(6-Hydroxy-3-sulfoquinolin-7-yloxy)acetyl]hydrazine (Q4). 154 mg (0.5 mmol) Q3 and 0.5 mL solution of N2H4.H2O 80% were added to 15 mL EtOH. The reaction mixture was refluxed for 10 h. and then was evaporated to volume 5 mL. The resulting solution was cooled to 5 oC. The crystalline compound obtained was isolated by filtration and recrystallized from EtOH/water 3:1 by volume. The yield was 97 mg (62%), light yellow crystals, decomposed above 270 oC. IR (cm-1): 3506, 3334, 3227 (OH, NH); 3048, 2933 (C-H); 1690 (C=O); 1508, 1490 (ring). 1H NMR and 13C NMR see Tables 1 and 2. ESI MS, m/z (au)/relative intensity (%): +MS, 314/100 (M+H+), -MS: 312/100 (M-H+). Anal. Calcd. for C12H11NO7S (M 313.28): C, 46.01; H, 3.54; N, 4.47. Found: C, 46.38; H, 3.44; N, 4.21.
(6-Hydroxy-5-nitro-3-sulfoquinolin-7-yloxy)acetic acid (Q5). To a stirring solution of 299 mg (1 mmol) Q1 and 1 mL H2SO4 in 10 mL AcOH was slowly added 1 mL HNO3 and stirred at room temperature for 2 h. The reaction mixture was kept at room temperature for 12 h. The crystalline compound obtained was isolated by filtration and washed with water and EtOH. The crude product was recrystallized from EtOH/water 3:1 by volume to afford the product as yellow crystals, decomposed above 250 oC. The yield was 190 mg (55%). IR (cm-1): 3592, 3513 (OH); 3094, 2950 (C-H); 1730 (C=O); 1629, 1503, 1434 (ring). 1H NMR and 13C NMR see Tables 1 and 2. ESI MS, m/z (au)/relative intensity (%): +MS, 345/2 (M+H+), -MS: 343/100 (M-H+). Anal. Calcd. for C11H8N2O9S (M 344.25): C, 38.38; H, 2.34; N, 8.14. Found: C, 38.61; H, 2.12; N, 7.89.
(5-Amino-6-hydroxy-3-sulfoquinolin-7-yloxy)acetic acid (Q6). To 6 mL of solution 1M NaOH were added 1.1 g (6 mmol) Na2S2O4 and then 344 mg (1 mmol) Q5. The reaction mixture was heated at 90 oC for 5 h. The solid obtained was isolated by filtration, washed with water and recrystallized from MeCN/water 2:1 by volume to afford the product as yellow crystals, decomposed above 250 oC. The yield was 160 mg (51%). IR (cm-1): 3430, 3388, 3288 (OH, NH); 3089, 2960, 2932 (C-H); 1666 (C=O); 1628, 1510, 1480 (ring). 1H NMR and 13C NMR see Tables 1 and 2. ESI MS, m/z (au)/relative intensity (%): -MS, 313/38 (M-H+). Anal. Calcd. for C11H10N2O7S (M 314.27): C, 42.04; H, 3.21; N, 8.91. Found: C, 39.86; H, 3.43; N, 9.18.
(5-Chloro-6-hydroxy-3-sulfoquinolin-7-yloxy)acetic acid (Q7). 299 mg (1 mmol) Q1 was dissolved in 10 mL solution of concentrated HCl/water 1:1. To the resulting solution 60 mg KClO3 was added at 40 oC for 30 min. The solid obtained was isolated by filtration, washed with water and recrystallized from water to afford the product as light yellow crystals, decomposed above 280 oC. The yield was 227 mg (62%). IR (cm-1): 3490 (OH); 3089, 2925 (C-H); 1740, 1643 (C=O); 1620, 1592, 1503 (ring). 1H NMR and 13C NMR see Tables 1 and 2. ESI MS, m/z (au)/relative intensity (%): +MS, 334/100 (35Cl), 335/12 (13C), 336/29 (37Cl + 34S), 337/6 (13C), 338/2 (34S), (M+H+); -MS: 332/100 (35Cl), 333/16 (13C), 334/32 (37Cl + 34S), 335/5 (13C), 336/2 (34S), (M-H+). Anal. Calcd. for C11H8ClNO7S (M 333.70): C, 39.59; H, 2.42; N, 4.20. Found: C, 39.30; H, 2.55; N, 4.47.
(5-Bromo-6-hydroxy-3-sulfoquinolin-7-yloxy)acetic acid (Q8). To a solution of 299 mg (1 mmol) Q1 in 3 mL DMSO was added 0.2 mL Br2 and stirred for 5 h. The reaction mixture poured into 5 mL water. After 5 h. the solid obtained was isolated by filtration, washed with water and recrystallized from water to afford the product as light yellow crystals, decomposed above 240 oC. The yield was 265 mg (70%). IR (cm-1): 3363 (OH); 3048, 2933, 2855 (C-H); 1726, 1654 (C=O); 1592, 1503 (ring). 1H NMR and 13C NMR see Tables 1 and 2. ESI MS, m/z (au)/relative intensity (%): +MS, 378/78 (79Br), 379/11 (13C), 380/73 (81Br ), 381/12 (13C), 382/5 (34S), (M+H+); -MS, 376/100 (79Br), 377/10 (13C), 378/95 (81Br ), 379/13 (13C), 380/4 (34S), (M-H+). Anal. Calcd. for C11H8BrNO7S (M 378.15): C, 34.94; H, 2.13; N, 3.70. Found: C, 35.21; H, 2.28; N, 3.38.
Methyl (5-bromo-6-hydroxy-3-sulfoquinolin-7-yloxy)acetate (Q9). A solution of 378 mg (1 mmol) Q8, 2 mL DMSO and 0.1 mL H2SO4 in 5 mL MeOH was refluxed for 6 h. The reaction mixture was cooled to room temperature. The solid obtained was isolated by filtration, washed with EtOH and recrystallized from EtOH/water 1:1 by volume to afford the product as needle yellow crystals, decomposed above 234 oC. The yield was 255 mg (65%). IR (cm-1): 3420 (OH); 3081, 3024, 2940 (C-H); 1769 (C=O); 1620, 1550, 1493 (ring). 1H- and 13C NMR see Tables 1 and 2. ESI MS, m/z (au)/relative intensity (%): -MS, 390/100 (79Br), 391/16 (13C), 392/94 (81Br + 34S), 393/14 (13C), 394/6 (34S). Anal. Calcd. for C12H10BrNO7S (M 392.18): C, 36.75; H, 2.57; N, 3.57. Found: C, 36.47; H, 2.78; N, 3.52.
[(5-Bromo-6-hydroxy-3-sulfoquinolin-7-yloxy)acetyl]hydrazine (Q10). A solution of 196 mg (0.5 mmol) Q9, 2 mL DMSO and 1 mL solution of N2H4.H2O 80% in 5 mL EtOH was refluxed for 8 h. The reaction mixture was cooled to room temperature. The solid obtained was isolated by filtration, washed with EtOH and recrystallized from EtOH/water 1:1 by volume to afford the product as light yellow crystals, decomposed above 235 oC. The yield was 135 mg (72%). IR (cm-1): 3322, 3221 (OH, NH); 3080, 2922, 2851 (C-H); 1659 (C=O); 1628, 1543, 1457 (ring). 1H- and 13C NMR see Tables 1 and 2. ESI MS, m/z (au)/relative intensity (%): +MS, 392/100 (79Br), 393/16 (13C), 394/95 (81Br + 34S), 395/8 (13C), 396/3 (34S); -MS, 390/95 (79Br), 391/15 (13C), 392/100 (81Br + 34S), 393/13 (13C), 394/5 (34S). Anal. Calcd. for C11H10BrN3O6S (M 392.18): C, 33.69; H, 2.57; N, 10.71. Found: C, 33.96; H, 2.36; N, 10.45.
ACKNOWLEDGEMENTS
This work was supported by Vietnam National Foundation for Science and Technology Development (NAFOSTED).
References
1. M. Foley and L. Tilley, Pharmacology & Therapeutics, 1998, 79, 55. CrossRef
2. S. Meshnick and M. Dobson, ‘Antimalarial Chemotherapy. Mechanisms of Action, Resistance, and New Directions in Drug Discovery’, Humana Press, 2001.
3. A. Mohammed, N. Abdel-Hamid, F. Maher, and A. Farghaly, Coll. Czech. Chem. Commun., 1992, 57, 1547. CrossRef
4. N. Koseva, O. Stoilova, N. Manolova, I. Rashkov, and P.-J. Madec, J. Bioact. and Compat. Polym., 2001, 16, 3. CrossRef
5. L. Savini , L Chiasserini, A. Gaeta, and C. Pellerano, Bioorg. Med. Chem., 2002, 10, 2193. CrossRef
6. A. Nayyar, A. Malde, E. Coutinho, and R. Jain, Bioorg. Med. Chem., 2006, 14, 7302. CrossRef
7. S. Gemma, L. Savini, M. Altarelli, P. Tripaldi, L. Chiasserini, S. Coccone, V. Kumar, C. Camodeca, G. Campiani, E. Novellino, S. Clarizio, G. Delogu, and S. Butini, Bioorg. Med. Chem., 2009, 17, 6063. CrossRef
8. A. Dlugosz and D. Dus, Farmaco., 1996, 51, 367.
9. N. H. Dinh, N. T. Ly, and L. T. T. Van, J. Heterocycl. Chem., 2004, 41, 1015. CrossRef
10. T. T. Da, Y.-M. Kim, N. T. T. Chi, L. X. Chien, and N. H. Dinh, Organometallics, 2008, 27, 3611. CrossRef
11. N. H. Dinh, Ng. T. Ly, and P. V. Hoan, J. Heterocycl. Chem., 2006, 43, 1657. CrossRef
12. T. T. Da, Y. Kim, T. T. C. Mai, N. C. Cuong, and N. H. Dinh, J. Coord. Chem., 2010, 63, 473. CrossRef
13. N. H. Dinh, N. Q. Trung, N. D. Dat, and N. Hien, J. Heterocycl. Chem., in press.
14. P. Prakash and N. Gupta, Indian J. Physiol. Pharmacol., 2005, 49, 125.
15. R. Clauser, Monatsh. 1901, 22, 123. CrossRef
16. N. H. Dinh, T. T. Huan, D. N. Toan, P. M. Kimpende, and L. V. Meervelt, J. Mol. Struct., 2010, 980, 137. CrossRef
17. V. V. Kouznetsov, L. Y. V. Méndez, and C. M. M. Gómez, Curr. Org. Chem., 2005, 9, 141. CrossRef
18. W. E. Noland, Chem. Rev., 1955, 55, 137. CrossRef
19. G. M. Sheldrick, SADABS v2.03: Area-Detector Absorption Correction, University of Göttingen, Germany, 1999.
20. G. M. Sheldrick, SHELXL-97, University of Göttingen, Germany, 1997.