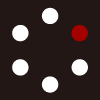
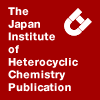
HETEROCYCLES
An International Journal for Reviews and Communications in Heterocyclic ChemistryWeb Edition ISSN: 1881-0942
Published online by The Japan Institute of Heterocyclic Chemistry
e-Journal
Full Text HTML
Received, 9th January, 2012, Accepted, 13th February, 2012, Published online, 21st February, 2012.
DOI: 10.3987/COM-12-12424
■ Halenaquinone Derivatives from Tropical Marine Sponge Xestospongia sp.
Yeon-Ju Lee, Chang-Kwon Kim, Song-Kyu Park, Jong Soon Kang, Jong-Seok Lee, Hee Jae Shin, and Hyi-Seung Lee*
Marine Natural Products Laboratory, Korea Ocean Research & Development Institute, Ansan PO Box 29, Ansan 426-744, Korea
Abstract
Chemical investigations of the tropical marine sponge of the genus Xestospongia have resulted in the isolation of a new halenaquinone derivative named xestosaprol N (8) together with known metabolites. Their structures were elucidated on the basis of mass spectrometry and detailed 2D NMR spectroscopic data.Marine sponges are proving to be productive sources of many interesting bioactive polyketides, including a series of pentacyclic quinone and hydroquonone compounds. Halenaquinone derivatives isolated from sponges of the genera Xestospongia,1-7 Adocia,8 and Petrosia9 have exhibited antimalarial activity,13 and inhibition activity against various enzymes, including protein tyrosine kinase,3,10 topoisomerase II,4 Cdc25B phosphatase,5 phospholipase A2,7 farnesyltransferase,7 Na+,K+-ATPase,11 and phosphatidylinositol 3-kinase.12
During the course of our search for biologically active constituents from tropical marine sponges,14-16 we encountered a sponge of the genus Xestospongia from Federated States of Micronesia, and a crude extract from the sponge exhibited moderate growth inhibition against several human tumor cell lines. Directed by the results of 1H NMR analysis, the crude extracts were separated by employing solvent partitioning followed by various chromatographic methods including reversed-phase vacuum flash chromatography, and C18 HPLC to afford the pure halenaquinone derivatives (1–8) (Figure 1). Herein we describe the structure elucidation and biological evaluation of these secondary metabolites.
Compound 1 was identified as halenaquinol sulfate by combined spectroscopic analyses and comparison of NMR data with those reported previously.1 The 1H and 13C NMR spectra of compounds 1–7 were closely related to each other and all of them possess a polycyclic moiety. By comparing the spectral data of these compounds with previously reported spectral data, their structures were readily identified as being those of xestoquinol sulfate (2),2 xestosaprol B (3),2 halenaquinone (4),17 tetrahydrohalenaquinone A (5),18 and B (6),2 and adociaquinone B (7).8
Compound 8 was isolated as a yellow amorphous solid, whose molecular formula was determined to be C21H19NO7S on the basis of HRESIMS analysis. The IR spectrum showed characteristic hydroxyl (3468 cm-1) and carbonyl (1686 cm-1) absorption bands. The UV spectrum, with absorption maxima at 215 and 278 nm, suggested the presence of a polycyclic polyketide.
The 1H and 13C NMR spectra of compound 8 measured in acetone-d6 exhibited signals characteristic of a halenaquinone derivative (Table 1). There were two aromatic singlets (δH 8.80 and 8.40), one methine (δH 4.27), five methylenes (δH 4.15, 3.38, 3.40/2.49, 2.37/1.98, 2.07/1.87), and one methyl singlet (δH 1.61). The 1H–1H COSY spectrum exhibited correlations between the oxymethine protons at δH 4.27 (H-2) and two pairs of methylene at δH 3.40/2.49 (H-1) and δH 2.37/1.98 (H-3) (Figure 2). A combination of the HSQC and HMBC data allowed for the assignment of the characteristic 13C NMR signals, and the HMBC correlations from δH 8.80 (H-10) to δC 174.8 (C-8) and δC 180.1 (C-12), from δH 8.40 (H-17) to δC 40.6 (C-5) and δC 134.8 (C-9), from δH 1.61 (H-19) to δC 136.7 (C-6) and δC 158.1 (C-18), and from δH 4.13 (H-21) to δC 147.5 (C-13) and δC 49.8 (C-20) confirmed the connectivity of the polycyclic moieties. The important COSY and HMBC interactions of compound 8 are shown in Figure 2. The 1H and 13C NMR data for 8 were compared with the published values for adociaquinone B (7),8 and the major difference between them was found to be the absence of signals for a furan ring in 8.
The relative configurations of the 2-hydroxy and 5-methyl groups in 8 were assigned as 2S* and 5S* by NOESY experiments and analyses of the magnitudes of the proton-proton couplings observed.19 NOESY cross-peaks at H-1 (axial, δH 2.49)/H-19 and H-3 (axial, δH 2.37)/H-19 and small coupling constant (J = 3.0 Hz) were evidence that H-2 was established in an equatorial position of the chair conformer (Figure 3). These assignments are consistent with those the previously reported for xestosaprol B (3), and tetrahydrohalenaquinone A (5) and B (6). Therefore, compound 8 was deduced to be a new metabolite, named xestosaprol N.
Compounds 1-8 were evaluated for their growth inhibition activities against six human tumor cell lines including ACHN (renal), HCT-15 (colon), MDA-MB-231 (breast), NCI-H23 (lung), NUGC-3 (stomach) and PC-3 (prostate). The absorbance was measured in quadruplicate and the percentage of cell viability was calculated.21 In vitro growth inhibition activity was examined after 48 h treatment of cells by SRB (sulforhodamine B) assay. Adriamycin was used as positive control and the results are summarized in Table 2. As can be seen from the table, adociaquinone B (7) showed significant growth inhibition against ACHN, NCI-H23, NUGC-3, and PC-3 with GI50 values of 0.96, 0.99, 0.96, and 0.87 µg/mL, respectively. Halenaquinone (4) also displayed moderate activities against all of the tumor cell lines tested in this study with GI50 values of 3.68-8.05 µg/mL, while GI50 values of compounds 1-3, 5 and 6 were not be able to obtain because these compounds did not show any inhibitory activity against tumor cell growth at 30 µg/mL, the maximal concentration used.
The structural peculiarity in achieving high growth inhibitory values for tumor cell lines is not clear, with the adociaquinone B (7) and halenaquinone (4) being more active than other halenaquinone derivatives 1-3, 5, 6, 8. Although compound 8 shows weak activity against NCI-H23, and PC-3, 4, 7, 8, which have a naphthoquinone moiety, are active in this test. When comparing the relative activities of 4, 7, and 8, compound 4 and 7 are more potent than 8, which is absent furan ring in polycyclic polyketides. Thus, naphthoquinone and furan rings in compound appear to be an important for these activities.
In conclusion, biological and chemical investigations of the crude extract of the Micronesian marine sponge of the genus Xestospongia led to the isolation of a new halenaquinone derivative 8, together with seven known related polyketides. Their structures were elucidated on the basis of mass spectrometry and detailed 2D NMR spectroscopic data. Among the isolated halenaquinone derivative, adociaquinone B (7) and halenaquinone (4) displayed significant to moderate growth inhibitory activity against six human tumor cell lines, ACHN, HCT-15, MDA-MB-231, NCI-H23, NUGC-3 and PC-3.
EXPERIMENTAL
General Experimental Procedures
NMR spectra were recorded on a Varian Unity 500 instrument at 500 MHz for 1H and 125 MHz for 13C. All chemical shifts were recorded with respect to the residual solvent signals as an internal standard (CDCl3 δH 7.26 ppm, δC 77.0 ppm). IR spectra were recorded on a JASCO FT/IR-4100 spectrophotometer as thin films. UV spectra were obtained in MeOH using a Shimadzu UV-1650PC spectrophotometer. Mass spectral data were obtained at the Korean Basic Science Institute, Seoul, Korea. All solvents used were of spectroscopic grade or were freshly distilled from glass prior to use.
Animal Material
Specimens of Xestospongia sp. were collected by hand with scuba equipment at a depth of 15–20 m off the shore of Weno Island, Chuuk State, Federated States of Micronesia, in July, 2007. The sample was identified by Prof. C. J. Sim, Natural History Museum, Hannam University, and a voucher specimen has been deposited at the Marine Biotechnology Research Center, Korea Ocean Research & Development Institute, Korea, under the curatorship of Mr. S. Park.
Extraction and Isolation
The specimens were lyophilized (wet wt. 652 g) and repeatedly extracted with MeOH (1 L x 2) and CH2Cl2 (1 L x 1). The extracts were filtered and concentrated under reduced pressure to afford 76.4 g of crude extract. The residue was partitioned between H2O and n-BuOH to yield 28.5 g of organic-soluble material. The n-BuOH layer was re-partitioned between 15% aqueous MeOH (14.0 g) and n-hexane (14.4 g). The residue from the aqueous MeOH layer was subjected to C18 reversed-phase flash chromatography using gradient mixtures of MeOH and H2O (elution order: 50%, 40%, 30%, 20%, 10% aq. MeOH, and 100% MeOH). The fraction eluted with 50% aqueous MeOH (3.46 g) was dried and separated by reversed phase HPLC (YMC-ODS-AQ C18 column, 250 x 10 mm; 65% aqueous MeOH) to afford in the order of elution, 1, 3, 4, 5, 2, 8 and 6 as yellow solids. Proton NMR analysis revealed that compounds 1 and 6 were pure whereas the others contained impurities. Purifications of the remaining metabolites were then accomplished by reversed phase HPLC (YMC-ODS-A C18 column, 250 x 4.6 mm; 85% aqueous MeCN for 2, 70% aqueous MeOH for 3-5, 8). The fraction eluted with 30% aqueous MeOH (780 mg) was separated by reversed phase HPLC (YMC-ODS-A C18 column, 250 x 10 mm; 45% aqueous MeOH) to yield compounds 7. The purified metabolites were isolated in the following amounts: 61.0, 2.0, 4.5, 4.6, 7.7, 10.6, 8.0 and 3.0 mg for 1–8, respectively.
Xestosaprol N (8): amorphous solid, [α]D -18.0 (c 0.10, MeOH); UV (MeOH) λmax (logε) 278 (4.52), 215 (4.30); IR (neat) νmax 3468, 2964, 1686, 1344, 1040 cm-1; 1H and 13C NMR see Table 1; HRESI-MS m/z 452.0775 [M+Na]+, (calcd for C21H19NO7SNa, m/z 452.0780).
Cell Growth Inhibition Activity
Human cancer cell lines (ACHN, NCI-H23, MDA-MB-231, HCT-15, NUGC-3 and PC-3) were used for cell growth inhibition assays. The cells were cultured in RPMI 1640 medium supplemented with 5% fetal bovine serum at 37 oC in humidified air containing 5% CO2. Inhibition of cell growth for an individual compound was determined with the total protein SRB assay described previously.15,20 The cells were plated at a proper density in 96-well plates and incubated for 24 h. After further incubation for 48 h in the presence or absence of the compounds (at concentration of 0.3, 3, and 30 µg/mL) cells were fixed with 50% trichloroacetic acid and stained with sulforhodamine B. The absorbance was measured at 540 nm using a microplate reader.
ACKNOWLEDGEMENTS
We are grateful to the Department of Marine Resources, State of Chuuk, Federated States of Micronesia, for allowing our marine organism research. This work was partially supported by the Ministry of Land, Transport and Maritime Affairs (PM56641), and a grant (No. A101836) of the Korean Health Technology R&D Project, Ministry for Health, Welfare & Family Affairs, Republic of Korea.
References
1. M. Kobayashi, N. Shimizu, Y. Kyogoku, and I. Kitagawa, Chem. Pharm. Bull., 1985, 33, 1305. CrossRef
2. J. Kobayashi, T. Hirase, H. Shigemori, M. Ishibashi, M.-A. Bae, T. Tsuji, and T. Sasaki, J. Nat. Prod., 1992, 55, 994. CrossRef
3. K. A. Alvi, M. C. Diaz, P. Crews, D. L. Slate, R. H. Lee, and R. Moretti, J. Org. Chem., 1992, 57, 6604. CrossRef
4. G. P. Concepcion, T. A. Foderaro, G. S. Eldredge, E. Lobkovsky, J. Clardy, L. R. Barrows, and C. M. Ireland, J. Med. Chem., 1995, 38, 4503. CrossRef
5. S. Cao, C. Foster, M. Brisson, J. S. Lazob, and D. G. I. Kingston, Bioorg. Med. Chem., 2005, 13, 999. CrossRef
6. N. Millan-Aguinaga, I. E. Soria-Mercado, and P. Williams, Tetrahedron Lett., 2010, 51, 751. CrossRef
7. A. Longeon, B. R. Copp, M. Roue, J. Dubois, A. Valentin, S. Petek, C. Debitus, and M.-L. Bourguet-Kondracki, Bioorg. Med. Chem., 2010, 18, 6006. CrossRef
8. F. J. Schmitz and S. J. Bloor, J. Org. Chem., 1988, 53, 3922. CrossRef
9. Y. C. Shen, C. V. S. Prakasha, and J.-H. Guh, Tetrahedron Lett., 2004, 45, 2463. CrossRef
10. D. S. Lawrence and J. Niu, Pharmacol. Ther., 1998, 77, 81. CrossRef
11. I. A. Gorshkova, B. A. Gorshkov, S. A. Fedoreev, and V. A. Stonik, Comp. Biochem. Physiol., 2001, 128C, 531.
12. H. Fujiwara, K. Matsunaga, M. Saito, S. Hagiya, K. Furukawa, H. Nakamura, and Y. Ohizumi, Eur. J. Pharmacol., 2001, 413, 37. CrossRef
13. D. Laurent, V. Jullian, A. Parenty, M. Knibiehler, D. Dorin, S. Schmitt, O. Lozach, N. Lebouvier, M. Frostin, F. Alby, S. Maurel, C. Doerig, L. Meijerf, and M. Sauvain, Bioorg. Med. Chem., 2006, 14, 4477. CrossRef
14. H.-S. Lee, K.-M. Yoon, Y.-R. Han, K. J. Lee, S.-C. Chung, T.-I. Kim, S.-H. Lee, J. Shin, and K.-B. Oh, Bioorg. Med. Chem. Lett., 2009, 19, 1051. CrossRef
15. H.-S. Lee, J. H. Lee, H. Won, S.-K. Park, H. M. Kim, H. J. Shin, H. S. Park, C. J. Sim, and H.-K. Kim, Lipids, 2009, 44, 71. CrossRef
16. H.-S. Lee, T.-H. Lee, S. H. Yang, H. J. Shin, J. Shin, and K.-B. Oh, Bioorg. Med. Chem. Lett., 2007, 17, 2483. CrossRef
17. D. M. Roll, P. J. Scheuer, G. K. Matsumoto, and J. Clardy, J. Am. Chem. Soc., 1983, 105, 6177. CrossRef
18. K. A. Alvi, J. Rodriguez, M. C. Diaz, R. Moretti, R. S. Wilhelm, R. H. Lee, D. L. Slate, and P. Crews, J. Org. Chem., 1993, 58, 4871. CrossRef
19. J. Dai, A. Sorribas, W. Y. Yoshida, M. Kelly, and P. G. Williams, J. Nat. Prod., 2010, 73, 1188. CrossRef
20. S. C. Kuo, H. Z. Lee, J. P. Juang, Y. T. Lin, T. S. Wu, J. J. Chang, D. Lednicer, K. D. Paull, C. M. Lin, E. Hamel, and K. H. Lee, J. Med. Chem., 1993, 36, 1146. CrossRef