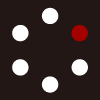
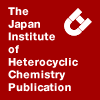
HETEROCYCLES
An International Journal for Reviews and Communications in Heterocyclic ChemistryWeb Edition ISSN: 1881-0942
Published online by The Japan Institute of Heterocyclic Chemistry
e-Journal
Full Text HTML
Received, 22nd January, 2012, Accepted, 8th February, 2012, Published online, 9th February, 2012.
DOI: 10.3987/COM-12-12433
■ Efficient Synthesis of 2,4-Diarylquinolines via Fe(III) Trifluoroacetate Catalyzed Three-Component Reactions under Solvent-Free Conditions
Min Zhang,* Ting Wang, Biao Xiong, Fengxia Yan, Xiaoting Wang, Yuqiang Ding, and Qijun Song
School of Chemistry and Materials Engineering, Jiangnan University, 1800 Lihu Road, Wuxi, Jiangsu Province, 214122, China
Abstract
A convenient and efficient one-pot synthesis of 2,4-diarylquinolines is described by using Fe(CF3CO2)3 as a catalyst for the first time. In this method, three-component coupling of arylaldehydes, arylamines and arylacetylenes furnished the corresponding products in good to excellent yields. The work features the use of cheap and eco-friendly catalyst, excellent functional group tolerance and solvent-free conditions. A variety of new products were afforded effectively.INTRODUCTION
2,4-Diarylquinolines constitute a significant important class of heterocyclic compounds found in numerous bioactive compounds1 and polymer materials.2 Additionally, 2-arylquinolines may serve as versatile building blocks for the synthesis of highly conjugated molecular devices via direct C-H bond functonalization,3 or valuable ligands for the preparation of cyclometalated photosensitisers and liquid crystal materials.4 Generally, 2,4-diarylquinolines can be prepared by well-established name reactions (i.e. Skraup,5 Friedländer,6 Combes,7 etc). And recently developed approaches8 also showed alternative pathways to achieve related goals. However, some of the methods to complete the assembly of desired products involve either the use of volatile organic solvents or the requirement of prefunctionalized reagents which would lead to tedious work-up procedures. Notably, more recently established domino reatctions from arylaldehydes, arylamines and arylacetylenes provided a direct and versatile pathway to synthesize substituted quinolines.9 In spite of these significant contributions, the development of efficient catalysis for such an important transformation with the merit of good functional tolerance and eco-compatibility still remains an interesting goal.
As a continuation of our research interest in the synthesis of functionalized quinolines10 and finding their applications in organometallic chemistry,11 we became interested in developing an efficient and general protocol for the construction of 2,4-diarylquinolines. During our work on the synthesis of beta-amino ketones by employing iron(III) trifluoroacetate catalyzed multicomponent reactions,12 the replacement of ketones with arylacetylenes resulted in 2,4-diarylquinolines exclusively (Scheme 1). Considering iron catalysis appeared as rising star has attracted considerable attention in recent years owing to its significant advantages such as high abundance in nature, easy availability, environmentally benign, economic attractiveness, etc.13 And iron(III) trifluoroacetate is a cost-effective, non-corrosive, crystalline catalyst with outstanding physical stability.14 We are therefore interested in further studying its catalytic application in 2,4-diarylquinoline syntheses. To the best of our knowledge, such a protocol has not been reported yet. Herein, we present a direct and efficient Fe(CF3CO2)3-catalyzed syntheses of 2,4-diarylquinolines.
RESULTS AND DISCUSSION
In order to formulate an efficient iron catalytic system, the synthesis of 6-methyl-2-(3-nitrophenyl)-4-phenylquinoline (4a) from 3-nitrobenzaldehyde 1a, p-toluidine 2a, and phenylacetylene 3a was chosen as a model reaction to evaluate the influence of different iron catalysts and solvents. Initially, the reactions were conducted at 100 oC for 5 hours by using 10 mol% of catalyst and THF as the reaction solvent. Among the catalysts tested (Table 1, entries 1-5), Fe(CF3CO2)3 showed the best reactivity and resulted in desired product in 56% yield (Table 1, entry 5). Gratifyingly, the product yield was increased to 67% under solvent free conditions (Table 1, entry 6). Further investigations showed that 10 mol% of Fe(CF3CO2)3 catalyst and 7 hours of reaction time are sufficient to give the best result (Table 1, entry 7). Employing the optimized reaction conditions also other polar or less polar solvents can be used, but the prodcut yields were somewhat lower (Table 1, entries 8-10).
With the best reaction conditions in hand, we then tested the substrate scope of this iron-catalyzed protocol. As shown in Scheme 2, all the reactions proceeded smoothly and gave the 2,4-diarylquinoline derivatives in good to excellent isolated yields. Electro-withdrawing and -donating groups on the aryl ring of different substrates are well tolerated. Specifically, substituents on arylaldehydes and anilines have significant influence on the product yields, while the ones on phenylacetylenes have only little influence on the reaction efficiency. Generally, the reactions using electron-deficient aldehydes (Scheme 2, see 4a-4t) or electron-rich anilines (Scheme 2, entries 4c and 4h) could result in higher product yields, which are associated with the easier formation of imines and subsequent generation of more reactive propargyglic amine intermediates in the presence of phenylacetylenes and iron catalyst. And the results are well consistent with the proposed mechanism in reported literatures.9 Interestingly, nitrobenzaldehyde could result in high product yields under our catalytic system (Scheme 2, see 4a, 4r and 4s), it is in marked contrast to early reported system.9b Reactions from o-chlorobenzadehydes gave relatively lower yields, and which are attributed to the effect of steric hindrance (Scheme 2, see 4b, 4f, 4i-4j, 4l, 4o and 4p). Notably, the product bearing a halogenated group has the potential for further manipulation of products (Table 2, see 4b, 4e, 4f, 4i-4j and 4l-4t).
In addition to investigate the regioselectivity of this synthetic protocol by using unsymmetrical m-anisidine 2e, which could lead to two possible regioisomers 4u and 4u’ (Scheme 3). However, the results indicated that product 4u was obtained exclusively, and the cyclization process occurs at less steric hindered position of the aniline. It clearly demonstrates that the steric factor is a major contributor to site the regioselectivity in the reaction. And 2,4,7-trisubstituted quinoline 4u was obtained in 81% isolated yield.
In conclusion, a convenient and efficient one-pot synthesis of 2,4-diarylquinolines is demonstrated by using Fe(CF3CO2)3 as a catalyst for the first time. A wide range of arylaldehydes, arylamines and arylacetylenes could be transformed into expected products in good to excellent yields in an efficient manner. Compared to the reported procedures, iron(III) trifluoroacetate is comparably inexpensive, eco-compatible and easily accessible,14 and the assembly of quinoline products occurs at lower temperature or in shorter reaction time with good functional tolerance and solvent-free conditions. Considering the significant advantages of this work, we are convinced it is and will be of practical value for the synthesis of 2,4-diarylquinolines.
EXPERIMENTAL
All the obtained compounds (except 4c, 4d and 4e, all other products are new ones) were characterized by melting points (mp), 1H-NMR, 13C-NMR, infrared spectra (IR), Low mass spectra (MS) and high-resolution mass spectra (HRMS). Melting points were measured on an Electrothemal SGW-X4 microscopy digital melting point apparatus and are uncorrected. IR spectra were recorded on a FTLA2000 spectrometer; 1H-NMR and 13C-NMR spectra were obtained on Bruker Avance-300. Chemical shifts were reported in parts per million (ppm, δ) downfield from tetramethylsilane. Proton coupling patterns of NMR are described as singlet (s), doublet (d), triplet (t), quartet (q), multiplet (m), and broad (br). LRMS were measured on an Agilent Technologies 6210 TOF LC/MS using H2O + 0.1% formic acid (10%) and methanol (90%) as eluent.
General procedure for synthesis of the 2,4-diarylquinolines
A schlenk tube equipped with a magnetic stirrer bar and also with a provision of circulating cool water was used in the experimentation, catalyst Fe(CF3CO2)3 (0.1 mmol), arylamine (1.05 mmol), arylaldehyde (1.0 mmol), alkyne (1.5 mmol) were introduced successively, and the resulting mixture was stirred in an open system at 100 °C for 7 h. TLC was used to detect the completion of the reaction. After cooling down to room temperature, the product was purified by flash column chromatography on silica, eluting with petroleum ether, ethyl acetate in the ratio of 20: 1, to provide desired quinoline products.
Analytical Data of all obtained new compounds
6-Methyl-2-(3-nitrophenyl)-4-phenylquinoline (4a)
Yield: 90%; Light yellow solid; mp 133-134 °C; 1H NMR (300 MHz, CDCl3) δ 8.99 (t, J = 1.8 Hz, 1H), 8.50 (m, 1H), 8.23 (m, 1H), 8.08 (d, J = 8.4 Hz, 1H), 7.75 (s, 1H), 7.67-7.43 (m, 8H), 2.43 (s, 3H); 13C NMR (75 MHz, CDCl3): δ 148.0, 144.4, 141.4, 133.7, 132.4, 129.6, 128.8, 124.3, 123.5, 121.8, 117.7, 113.6, 112.5, 21.5; IR (KBr, cm-1) νmax = 3005, 2974, 1619, 1556, 1529, 1486, 1341, 755, 701; MS (EI, m/z): 340 [M]+; HRMS (EI): Calcd. for C22H16N2O2 [M]+: 340.12; Found: 340.12116.
2-(2-Chlorophenyl)-6-nitro-4-phenylquinoine (4b)
Yield: 62%; Light yellow solid; mp 127-132 °C; 1H NMR (300 MHz, CDCl3) δ 8.85 (d, J = 2.7 Hz, 1H), 8.43 (dd, J = 9.3 Hz, 1H), 8.27 (d, J = 9.0 Hz, 1H), 7.81 (s, 1H), 7.73-7.65 (m, 1H), 7.56-7.44 (m, 6H), 7.40-7.33 (m, 2H); 13C NMR (75 MHz, CDCl3): δ 160.4, 150.8, 150.2, 145.9, 138.7, 136.6, 132.4, 131.9, 130.7, 129.6, 129.4, 127.4, 124.8, 123.1, 122.9; IR (KBr, cm-1) νmax = 3037, 2912, 1594, 1555, 1485, 1340, 845, 755, 743; MS (EI, m/z): 360 [M]+; HRMS (EI): Calcd. for C21H13ClN2O2 [M]+: 360.07; Found: 360.06638.
6-Methyl-2,4-diphenylquinoline (4c)
Yield: 91%; White solid; mp 123-125 °C; 1H NMR (300 MHz, CDCl3): δ 8.04-8.10 (m,3H), 7.56 (s, 1H), 7.35-7.49 (m, 10H), 2.38 (s, 3H).
2-(4-Methoxyphenyl)-6-methyl-4-phenylquinoline (4d)
Yield: 82%; White solid; mp 143-147 °C; 1H NMR (300 MHz, CDCl3): δ 8.02- 8.09 (m, 3H), 7.65 (s, 1H), 7.18-7.54 (m, 7H), 6.94-6.98 (d, J = 8.7 Hz, 2H), 3.81 (s,3H), 2.39 (s, 3H).
6-Chloro-2-(4-methoxyphenyl)-4-phenylquinoline (4e)
Yield: 76%; White solid; mp 138-141 °C; 1H NMR (300 MHz, CDCl3): δ 8.02-8.08 (m, 3H), 7.70-7.74 (d, J = 8.7 Hz, 2H), 7.43-7.56 (m, 6H), 6.94-6.96 (d, 2H), 3.79 (s, 3H).
6-Chloro-2-(2-chlorophenyl)-4-phenylquinoline (4f)
Yield: 73%; White solid; mp 138-141 °C; 1H NMR (300 MHz, CDCl3) δ 8.10 (d, J = 9.0 Hz, 1H), 7.87 (d, J = 2.4 Hz, 1H), 7.68-7.59 (m, 3H), 7.50-7.31 (m, 8H); 13C NMR (50 MHz, CDCl3): δ 157.6, 147.4, 139.9, 137.4, 132.9, 131.8, 131.7, 130.2, 129.6, 128.9, 128.8, 127.3, 124.6, 123.8; IR (KBr, cm-1) νmax = 3037, 3005, 1588, 1542, 1480, 1435, 1397, 1154, 1042, 890, 830, 785, 753, 700; MS (EI, m/z): 349 [M]+; HRMS (EI): Calcd. for C21H13Cl2N [M]+: 349.04; Found: 349.04247.
4-(4-tert-Butylphenyl)-6-methyl-2-phenylquinoline (4g)
Yield: 85%; White solid; mp 153-157 °C; 1H NMR (300 MHz, CDCl3): δ 8.11-8.05 (m, 3H), 7.70 (s, 1H), 7.65 (s, 1H) 7.52-7.34 (m, 8H), 2.41 (s, 3H), 1.36 (s, 9H); 13C NMR (75 MHz, CDCl3): δ 156.1, 151.4, 149.0, 139.5, 135.8, 131.8, 129.9, 128.7, 124.6, 126.0, 119.5, 34.6, 31.4, 32.8, 21.9; IR (KBr, cm-1) νmax = 3025, 2975, 1590, 1533, 1405, 1381, 972, 899, 745, 705; MS (EI, m/z): 351 [M]+; HRMS (EI): Calcd. for C26H25N [M]+: 351.20; Found: 351.19858.
4-(4-tert-Butylphenyl)-2-(4-methoxyphenyl)-6-methylquinoline (4h)
Yield: 90%; White solid; mp 137-143 °C; 1H NMR (300 MHz, CDCl3): δ 8.08-8.00 (m, 3H), 7.65 (s, 1H), 7.62 (s, 1H), 7.51-7.40 (m, 5H), 6.97-6.94 (m, 2H), 3.80 (s, 3H), 2.40 (s, 3H), 1.36 (s, 9H); 13C NMR (75 MHz, CDCl3): δ 160.8, 155.8, 151.1, 148.2, 146.9, 135.7, 131.6, 129.6, 129.3, 128.8, 125.6, 128.8, 125.6, 124.6, 119.1, 114.3, 55.4, 34.8, 31.4, 21.8; IR (KBr, cm-1) νmax = 3050, 2961, 2904, 2865, 2835, 1606, 1588, 1514, 1359, 1251, 1173, 1034, 832, 737; MS (EI, m/z): 381 [M]+; HRMS (EI): Calcd. for C27H27NO [M]+: 381.21; Found: 381.20925.
2-(2-Chlorophenyl)-6-methyl-4-phenylquinoline (4i)
Yield: 78%; White solid, mp 123-130 °C; 1H NMR (300 MHz, CDCl3): δ 8.06 (d, J = 8.7 Hz, 1H), 7.68-7.64 (m, 2H), 7.58 (s, 1H), 7.52-7.23 (m, 9H), 2.41 (s, 3H); 13C NMR (75 MHz, CDCl3): δ 156.1, 147.4, 147.3, 139.8, 136.9, 132.5, 131.8, 130.1, 129.9, 129.8, 129.7, 128.6, 128.4, 128.3, 127.2, 125.6, 124.5,123.1, 21.9; IR (KBr, cm-1) νmax = 3055, 3007, 2987, 1587, 1548, 1489, 1437, 1357, 1262, 1085, 1042, 826, 755, 701; MS (EI, m/z): 329 [M]+; HRMS (EI): Calcd. for C22H16ClN [M]+: 329.10; Found: 329.09701.
4-(4-tert-Butylphenyl)-2-(2-chlorophenyl)-6-methyl quinoline (4j)
Yield: 75%; White solid, mp 127-130 °C; 1H NMR (300 MHz, CDCl3): δ 8.04 (d, J = 8.4 Hz, 1H), 7.70 (s, 1H), 7.64 (dd, J = 7.2 Hz, 1H), 7.57 (s, 1H), 7.54-7.38 (m, 6H), 7.33-7.23(m, 2H), 2.41 (s, 3H), 1.32 (s, 9H); 13C NMR (75 MHz, CDCl3): δ 156.1, 147.4, 147.3, 139.8, 136.9, 132.5, 131.8, 130.1, 129.9, 129.8, 129.7, 128.6, 128.4, 128.3, 127.2, 125.6, 124.5,123.1, 21.9; IR (KBr, cm-1) νmax = 3055, 2962, 2865, 1586, 1546, 1493, 1439, 1042, 839, 826, 751, 599; MS (EI, m/z): 385 [M]+; HRMS (EI): Calcd. for C26H24ClN [M]+: 385.16; Found: 385.15972.
4-(4-tert-Butylphenyl)-6-methyl-2-phenylquinoline (4k)
Yield: 85%; Faint yellow solid; mp 147-150 °C; 1H NMR (300 MHz, CDCl3): δ 8.26-8.12 (m, 3H), 7.85 (s, 1H), 7.80 (s, 1H), 7.66-7.47 (m, 8H), 2.55 (s, 3H), 1.50 (s, 9H); 13C NMR (75 MHz, CDCl3): δ 156.1, 151.4, 148.5, 147.5, 139.9, 136.2, 135.7, 131.8, 129.9, 129.3, 129.1, 128.8, 127.5, 125.8, 125.6, 124.6, 119.5, 34.8, 31.5, 21.9; IR (KBr, cm-1) νmax = 3105, 2987, 2935, 2876, 1574, 1535, 1510, 1042, 879, 785, 814, 771; MS (EI, m/z): 351 [M]+; HRMS (EI): Calcd. for C26H25N [M]+: 351.20; Found: 351.19866.
4-(4-tert-Butylphenyl)-6-chloro-2-(2-chlorophenyl) quinoline (4l)
Yield: 80%; White solid; mp 157-159 °C; 1H NMR (300 MHz, CDCl3): δ 8.09 (d, J = 8.7 Hz, 1H), 7.93 (s, 1H), 7.90-6.88 (m, 10H), 1.33 (s, 9H); 13C NMR (75 MHz, CDCl3): δ 157.2, 151.9, 147.1, 134.5, 132.7, 132.4, 131.7, 130.4, 130.2, 35.1, 31.2; IR (KBr, cm-1) νmax = 3110, 3007, 2935, 2806, 1674, 1603, 1575, 1487, 823, 767, 703, 691; MS (EI, m/z): 405 [M]+; HRMS (EI): Calcd. for C25H21Cl2N [M]+: 405.11; Found: 405.10509.
4-(4-tert-Butylphenyl)-6-fiuoro-2-phenylquinoline (4m)
Yield: 90%; Light yellow solid; mp 157-159 °C; 1H NMR (300 MHz, CDCl3): δ 8.18 -8.07 (m, 3H), 7.77 (s, 1H), 7.53-7.34 (m, 9H), 1.35 (s, 9H); 13C NMR (75 MHz, CDCl3): δ 151.9, 148.5, 145.9, 129.4, 128.9, 127.5, 125.8, 119.9, 34.8, 31.4; IR (KBr, cm-1) νmax = 3007, 2984, 2875, 1593, 1473, 1435, 880, 845, 798, 655; MS (EI, m/z): 355 [M]+; HRMS (EI): Calcd. for C25H22FN [M]+: 355.17; Found: 355.17362.
6-Fluoro-2-(4-methoxyphenyl)-4-phenylquinoline (4n)
Yield: 87%; Faint yellow solid; mp 133-135 °C; 1H NMR (300 MHz, CDCl3): δ 8.12-8.03 (m, 2H), 7.77-7.69 (m, 2H), 7.50-7.35 (m, 4H), 7.12-6.76 (m, 5H), 3.79 (s, 3H); 13C NMR (75 MHz, CDCl3): δ 190.8, 160.9, 159.5, 132.3, 132.0, 131.9, 129.4, 128.8, 122.3, 119.7, 119.4, 115.9, 115.7, 114.4, 114.3, 109.5, 108.9, 55.6, 55.4; IR (KBr, cm-1) νmax = 2977, 2856, 2814, 1603, 1383, 1290, 910, 878, 754, 725, 580; MS (EI, m/z): 329 [M]+; HRMS (EI): Calcd. for C22H16FNO [M]+: 329.12; Found: 329.12159.
4-(4-tert-Butylphenyl)-2-(2,4-dichlorophenyl)-6-methylquinoline (4o)
Yield: 77%; Gray solid; mp 149-154 °C; 1H NMR (300 MHz, CDCl3): δ 8.12 (s, 1H), 7.87-7.91 (m, 2H), 7.75 (s, 1H), 7.24-7.47 (m, 7H), 2.35 (s, 3H), 1.34 (s, 9H); 13C NMR (75 MHz, CDCl3): δ 154.8, 151.5, 151.0, 148.9, 129.9, 129.7, 129.4, 127.7, 125.6, 121.1, 31.4, 21.4, 21.1; IR (KBr, cm-1) νmax = 3087, 3006, 2890, 2860, 990, 956, 874, 838, 771, 666; MS (EI, m/z): 419 [M]+; HRMS (EI): Calcd. for C26H23Cl2N [M]+: 419.12; Found: 419.12071.
2-(2,4-Dichlorophenyl)-6-fluoro-4-phenylquinoline (4p)
Yield: 73%; Light yellow solid; mp 129-133 °C; 1H NMR (300 MHz, CDCl3): δ 8.18-8.09 (m, 1H), 7.75-6.98 (m, 11H); 13C NMR (75 MHz, CDCl3): δ 153.0, 149.2, 135.0, 132.7, 130.4, 129.4, 128.4, 127.3, 125.5, 124.7, 123.5; IR (KBr, cm-1) νmax = 2977, 1804, 1573, 1498, 1005, 878, 820, 835, 779, 629, 575; MS (EI, m/z): 367 [M]+; HRMS (EI): Calcd. for C21H12Cl2FN [M]+: 367.03; Found: 367.03308.
4-(4-tert-Butylphenyl)-6-fluoro-2-(4-methoxy phenyl) quinoline (4q)
Yield: 86%; White solid; mp 149-151 °C; 1H NMR (300 MHz, CDCl3): δ 8.13-8.03 (m, 3H), 7.71 (s, 1H), 7.52-7.35 (m, 6H), 6.98-6.93 (m, 2H), 3.80 (s, 3H), 1.34 (s, 9H); 13C NMR (75 MHz, CDCl3): δ 160.8, 158.9, 156.4, 151.8, 148.6, 146.1, 145.6, 135.5, 135.0, 132.0, 129.1, 128.8, 125.8, 119.5, 114.2, 109.3, 108.6, 55.4, 34.8, 31.4; IR (KBr, cm-1) νmax = 3110, 3008, 2988, 2876, 1600, 1577, 1386, 1275, 1075, 898, 774, 698; MS (EI, m/z): 385 [M]+; HRMS (EI): Calcd. for C26H24FNO [M]+: 385.18; Found: 385.18418.
4-(4-tert-Butylphenyl)-6-fluoro-2-(3-nitrophenyl)quinoline (4r)
Yield: 70%; Gray solid; mp 151-154 °C; 1H NMR (300 MHz, CDCl3): δ 8.96 (s, 1H), 8.47 (d, J = 7.5 Hz, 1H), 8.23-8.13 (m, 2H), 7.79 (s, 1H), 7.63-7.42 (m, 7H), 1.35 (s, 9H); 13C NMR (75 MHz, CDCl3): δ 162.7, 160.6, 159.0, 152.2, 148.6, 145.9, 140.9, 134.6, 133.2, 129.9, 129.1, 125.9, 123.9, 122.3, 119.2, 110.8, 109.0, 34.9, 31.4; IR (KBr, cm-1) νmax = 2985, 2964, 2877, 1640, 1574, 897, 793, 753, 711, 632; MS (EI, m/z): 400 [M]+; HRMS (EI): Calcd. for C25H21FN2O2 [M]+: 400.16; Found: 400.15889.
4-(4-tert-Butylphenyl)-6-chloro-2-(3-nitrophenyl)quinoline (4s)
Yield: 93%; Gray solid; mp 157-161 °C; 1H NMR (300 MHz, CDCl3): δ 8.98 (s, 1H), 8.49 (d, J = 7.8 Hz, 1H), 8.24 (d, J = 7.2 Hz, 1H), 8.11 (d, J = 8.7 Hz, 1H), 7.88 (d, J = 1.9 Hz, 1H), 7.80 (s, 1H), 7.64-7.40 (m, 6H), 1.44 (s, 9H); 13C NMR (75 MHz, CDCl3): δ 154.3, 152.2, 149.3, 148.8, 147.2, 140.9, 133.3, 130.9, 129.9, 129.2, 126.0, 124.8, 122.4, 122.3, 119.4, 34.8, 31.4; IR (KBr, cm-1) νmax = 2968, 2933, 2813, 1747, 1656, 863, 743, 733, 701, 621. MS (EI, m/z): 416 [M]+; HRMS (EI): Calcd. for C25H21ClN2O2 [M]+: 416.13; Found: 416.12902.
ACKNOWLEDGEMENT
The authors are grateful to the funds of the National Natural Science Foundation of China (21101080), Fundamental Research Funds for the Central Universities of China (JUSRP10905, JUSRP211A07 and 1045210372090460), Natural Science Foundation of Jiangsu Province (BK2011144), and “333 Talent Project of Jiangsu Province”.
References
1. C. Praveen, P. Dheenkumar, D. Muralidharan, and P. T. Perumal, Bioorg. Med. Chem. Lett., 2010, 20, 7292. CrossRef
2. S. Ou, Z. H. Lin, C. Y. Duan, H. T. Zhang, and Z. P. Bai, Chem. Commun., 2006, 42, 4392. CrossRef
3. (a) E. F. Flegeau, C. Bruneau, P. H. Dixneuf, and A. Justand, J. Am. Chem. Soc., 2011, 133, 10161; CrossRef P. B. Arockiam, C. Fischmeister, C. Bruneau, and P. H. Dixneuf, Angew. Chem. Int. Ed., 2010, 49, 6629; CrossRef (c) I. Ozdemir, S. Demir, B. Cetinkaya, C. Gourlaouen, F. Maseras, C. Bruneau, and P. H. Dixneuf, J. Am. Chem. Soc., 2008, 130, 1156. CrossRef
4. (a) F. Gartner, A. Boddien, E. Barsch, K. Fumino, S. Losse, H. Junge, D. Hollmann, A. Bruckner, R. Ludwig, and M. Beller, Chem. Eur. J., 2011, 17, 6425; CrossRef (b) S. C. Lee and Y. S. Kim, Mol. Cryst. Liq. Cryst., 2009, 509, 1034. CrossRef
5. Z. H. Skraup, Ber. Dtsch. Chem. Ges., 1880, 13, 2086.
6. (a) H. M. Wang, R. S. Hou, H. D. Du, I. J. Kang, and L. C. Chen, Heterocycles, 2011, 83, 331; CrossRef (b) M. Dabiri and S. Bashiribod, Molecules, 2009, 14, 1126; CrossRef (c) P. Friedländer, Ber. Dtsch. Chem. Ges., 1882, 15, 2572. CrossRef
7. A. Combes, Bull. Soc. Chim. Fr., 1888, 49, 89.
8. (a) I. M. Baltork, S. Tangestaninejad, M. Moghadam, V. Mirkhani, S. Anvar, and A. Mirjafari, Synlett, 2010, 20, 3104; CrossRef (b) B. Gabriele, R. Mancuso, E. Lupinacci, R. Spina, G. Salerno, L. Veltri, and A. Dibenedetto, Tetrahedron, 2009, 65, 8507; CrossRef (c) J. Horn, S. P. Marsden, A. Nelson, D. House, and G. G. Weingarten, Org. Lett., 2008, 10, 4117; CrossRef (d) R. Sarma and D. Prajaparti, Synlett, 2008, 19, 3001; CrossRef (e) B. Gabriele, R. Mancuso, G. Salerno, G. Ruffolo, and P. Plastina, J. Org. Chem., 2007, 72, 6873; CrossRef (f) X. Y. Liu, P. Ding, J. S. Huang, and C. M. Che, Org. Lett., 2007, 9, 2645; CrossRef (g) C. S. Cho and J. U. Kim, Tetrahedron Lett., 2007, 48, 3775; CrossRef (h) R. P. Korivi and C. H. Cheng, J. Org. Chem., 2006, 71, 7079. CrossRef
9. (a) X. Zhang, B. Q. Liu, X. Shu, Y. Gao, H. P. Lv, and J. Zhu, J. Org. Chem., 2012, 77, 501; CrossRef (b) J. Tang, L. M. Wang, D. Mao, W. B. Wang, L. Zhang, S. Y. Wu, and Y. S. Xie, Tetrahedron, 2011, 67, 8465; CrossRef (c) X. J. Li, Z. J. Mao, Y. G. Wang, W. X. Chen, and X. F. Lin, Tetrahedron, 2011, 67, 3858; CrossRef (d) A. Kumar and V. K. Rao, Synlett, 2011, 2157; CrossRef (e) A. Kulkarni and B. Torok, Green. Chem., 2010, 12, 875; CrossRef (f) K. Cao, F. M. Zhang, Y. Q. Tu, X. T. Zhuo, and C. A. Fan, Chem. Eur. J., 2009, 15, 6332; CrossRef (g) S. K. Guchhait, K. Jadeja, and C. Madaan, Tetrahedron Lett., 2009, 50, 6861; CrossRef (h) H. Huang, H. L. Jiang, K. X. Chen, and H. Liu, J. Org. Chem., 2009, 74, 5476; CrossRef (i) S. Kikuchi, M. Iwai, and S.-i. Fukuzawa, Synlett, 2007, 2639. CrossRef
10. (a) M. Zhang, T. Roisnel, and P. H. Dixneuf, Adv. Synth. Catal., 2010, 352, 1896; CrossRef ; CrossRef (c) M. Zhang, B. Xiong, W. Yang, D. N. T. Kumar, and Y. Q. Ding, Monatsh. Chem., 2012, 143, 471. CrossRef
11. W. Yang, H. Fu, Q. J. Song, M. Zhang, and Y. Q. Ding, Organometallics, 2011, 30, 77. CrossRef
12. M. Zhang, B. Xiong, W. Yang, L. Chen, F. Wu, Q. Wang, and Y. Q. Ding, Synth. Commun., 2011, DOI:10.1080/00397911.2011.569866. CrossRef
13. (a) S. Enthaler, K. Junge, and M. Beller, Angew. Chem. Int. Ed., 2008, 47, 3317; CrossRef (b) A. Correa, G. O. Mancheno, and C. Bolm, Chem. Soc. Rev., 2008, 37, 1108. CrossRef
14. (a) H. Adibi, H. A. Samimi, and M. Beygzadeh, Catal. Commun., 2007, 8, 2119; CrossRef (b) N. Iranpoor and H. Adibi, Bull. Chem. Soc. Jpn., 2000, 73, 675. CrossRef