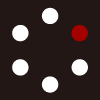
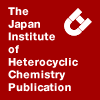
HETEROCYCLES
An International Journal for Reviews and Communications in Heterocyclic ChemistryWeb Edition ISSN: 1881-0942
Published online by The Japan Institute of Heterocyclic Chemistry
e-Journal
Full Text HTML
Received, 8th February, 2012, Accepted, 19th March, 2012, Published online, 23rd March, 2012.
DOI: 10.3987/COM-12-12442
■ One Step Synthesis of Optically Active Diazabicyclo[3.3.0]octanones or Diazabicyclo[4.3.0]nonanones by Asymmetric Conjugate Addition of Cyclic Hydrazine
Chigusa Seki,* Masafumi Hirama, Takeshi Sato, Saya Takeda, Yoshihito Kohari, Kazuhiro Ishigaki, Masafumi Ohuchi, Kiyoshi Yokoi, Hiroto Nakano, Koji Uwai, Nobuhiro Takano, Kazuyuki Umemura, and Haruo Matsuyama*
Department of Applied Chemistry, Graduate School of Engineering, Muroran Institute of Technology, 27-1 Mizumoto, Muroran, Hokkaido 050-8585, Japan
Abstract
Asymmetric conjugate addition-cyclization of lithium amide of 6-membered hydrazine 4, piperidazine, (or 5-membered hydrazine 6, pyrazolidine) to N-(E)-cinnamoyl-(1S)-2,10-camphorsultam (1S)-3 afforded β-amino acid derivative (S)-1 {or (S)-2} for the synthesis of polyamine alkaloids in good yield with good stereoselectivity (up to 86% ee).A number of β-amino acids have been isolated in free form and show interesting pharmacological properties.1 In addition, many classes of natural products contain β-amino acid derivatives as fragments. Macrocyclic lactams containing a biogenetic base such as spermine or spermidine alkaloids, which have a framework of β-amino acid such as β-phenyl-β-alanine, are particularly interested as synthetic targets in view of their broad biological activity, antibiotics, and antihypertensives.2,3 The conjugate addition of nitrogen anion such as lithium amide reagents (R2N- Li+) to α,β-unsaturated ester derivatives is one of the most versatile synthetic methods for the introduction of a new asymmetric center at the β-position of the carbonyl containing motif. Yamamoto et al. have reported nucleophilic reactions of R2NM, such as conjugate addition to enoates, and asymmetric synthesis of β-lactams was accomplished using amide cuprate reagents as a new class of nitirogen nucleophiles.4 The introduction of chirality using a chiral auxiliary is a useful way of producing a wide variety of optically active organic compounds. (1S)-(–)-2,10-camphorsultam and its antipode, accessible from inexpensive (–)- and (+)-camphorsulfonic acid in two simple operations, were introduced by Oppolzer et al.5 and rank today among the most practical auxiliaries. Almost all of their N-acyl derivatives are stable and can be (1) readily purified by crystallization, (2) directly analyzed by 1H NMR to determine their stereochemical purity, and (3) cleaved (e.g. with LiAlH4, LiOH, etc.) under mild conditions without loss of the induced chirality and with excellent recovery of the auxiliary. Our continuing interest in the development of synthetic pathway to the polyamine macrocycles prompted us to investigate the synthesis of optically active bicyclic lactam, (S)-(–)-9-pheny-1,6-diazabicyclo[4.3.0]nonan-7-one (1) and (S)-(–)-4-phenyl-1,5-diazabicyclo[3.3.0]octan-2-one (2). Starting from (S)-1, naturally occurring (S)-celacinnine6 could be synthesized via nine-membered azalactam7 and the synthesis of (S,S)-homaline8 could be achieved starting from (S)-2 via eight-membered azalactam. We investigated asymmetric conjugate addition of lithium amide of 6-membered hydrazine 4 (or 5-membered hydrazine 6) to N-(E)-cinnamoyl-(1S)-2,10-camphorsultam (1S)-3 to afford β-amino acid derivative (S)-1 {or (S)-2} in good yield with good stereoselectivity.
N-(E)-cinnamoyl-(1S)-2,10-camphorsultam (1S)-3 was obtained in 91% yield by deprotonation of (1S)- (–)-2,10-camphorsultam (1S)-5 with NaH in anhydrous toluene, followed by addition of (E)-cinnamoyl chloride and a catalytic amount of copper(I) chloride.9 The conjugate addition of 6-membered hydrazine 4 (5 equiv), piperidazine, to (1S)-3 (1 equiv) proceeded at 0 oC in THF (10 mL) as a solvent to afford cyclization product (S)-9-pheny-1,6-diazabicyclo[4.3.0]nonan-7-one (S)-1 in 80% yield with 44% ee and (1S)-(–)-2,10-camphorsultam (1S)-5 (Table 1, entry 1). The conjugate addition of lithium amide of 4 (3 equiv) to (1S)-3 (1 equiv) proceeded successfully at 0 oC in THF to give (S)-1 in 79% yield with 57% ee and (1S)-5 (entry 2). It is found that increasing the amount of lithium amide of 6-membered hydrazine 4, from 3 equiv to 5 equiv, the chemical yield and stereoselectivity of (S)-1 was increased up to 84% yield with 66% ee and (1S)-5 (entry 3).
Then we have investigated the optimization of the reaction conditions for the conjugate addition of lithium amide reagent of 6-membered hydrazine 4 (5 equiv) to (1S)-3 (1 equiv) in the several solvents and the chemical yields of (S)-1 are moderate to good (64-85%) (Table 2, entries 1-5). The stereoselectivity of (S)-1 is summarized in Table 2 as follows: THF (66% ee), tert-butyl methyl ether (76% ee), Et2O (86% ee), cyclopentyl methyl ether (CPME) (74% ee) or toluene (75% ee) (Table 2, entries 1-5). The results of Table 2 show that using Et2O as a solvent at 0 oC was the better reaction conditions and (S)-1 was obtained in 83% yield with 86% ee (entry 3).10 The good stereoselectivity of (S)-1 in Et2O might be dependent on the aggregation state of lithium amide of 4, because solvated structures of dimeric or higher aggregate lithium amides can be estimated in Et2O.
Similarly, the conjugate addition of lithium amide of 5-membered hydrazine 6 (5 equiv), pyrazolidine, to N-(E)-cinnamoyl-2,10-camphorsultam (1S)-3 (1 equiv) at 0 oC also proceeded successfully to give cyclization product (S)-4-phenyl-1,5-diazabicyclo[3.3.0]octan-2-one (S)-2 and (1S)-(–)-2,10- camphorsultam (1S)-5 (Scheme 1). The chemical yield and steroselectivity of conjugate addition of lithium amide reagent of 5-membered hydrazine 6 to (1S)-3 was examined in the several solvents and the results are shown in Tabel 2. The stereoselectivity of (S)-2 is as follows: THF (70% ee), tert-butyl methyl ether (76% ee), Et2O (77% ee),11 cyclopentyl methyl ether (CPME) (77% ee) or toluene (75% ee) (Table 2, entries 6-10). The results of Table 2 show that using Et2O (or CPME) as a solvent at 0 oC was the better reaction conditions and (S)-2 was obtained in 90% yield with 77% ee (or 69% yield with 77% ee) (entries 8 and 9).
The conjugate addition of 5-membered hydrazine 6 was further studied using other cinnamoyl substrates 7a-e containing electron-donating (R = OMe, Me) and electron–withdrawing substituents (R= NO2, F, Cl) on the aromatic ring. The conjugate addition of 6 (5 equiv) to (1S)-7a-e (1 equiv) underwent in Et2O-THF at room temperature, however, the stereoselectivities of 8a-e are moderate (46% ee - 64% ee) (Scheme 2). The conjugate addition of lithium amide of 6 (5 equiv) to (1S)-7a (R = OMe) (1 equiv) underwent smoothly at 0 oC in diethyl ether to give (S)-8a (R = OMe) in 88% yield with 64% ee (Scheme 2). It was found that the reaction of lithium amide of 6 (5 equiv) and (1S)-7b (R = NO2) (1 equiv) also afforded (S)-8b (R = NO2) in 70% yield with 86% ee.12 The electron-deficient cinnamoyl substrate (1S)-7b (R = NO2) showed better stereoselectivity on the conjugate addition of lithium amide of 6.
Based on the X-ray structure of N-crotonoyl-(1S)-2,10-camphorsultam reported by Oppolzer et al.13 and good enentioselectivity (up to 86% ee) of the β-amino acid derivatives (S)-1 obtained from the reaction of (1S)-3 and 4, the asymmetric conjugate addition course was proposed as follows (Figure 2). Due to the bulky camphorsultam group in (1S)-3, the nitrogen nucleophile (lithium amide of 4) may attack on the β-carbon atom of the double bond of (1S)-3 from the down side of the plane (si-face). Then cyclization of β-amino acid derivative took place successfully to afford (S)-1 and (1S)-5.
In summary, we could accomplish the synthesis of β-amino acid derivative (S)-1 {or (S)-2} for the synthesis of polyamine alkaloids in good yield with good stereoselectivity (up to 86% ee) by asymmetric conjugate addition-cyclization of lithium amide of 6-membered hydrazine 4 (or 5-membered hydrazine 6) to N-(E)-cinnamoyl-(1S)-2,10-camphorsultam (1S)-3.
References
1. For β-amino acid, see: (a) S. Shinagawa, T. Kawamura, S. Harada, M. Arai, and H. Okazaki, J. Med. Chem., 1987, 30, 1458; CrossRef (b) A. Bongini, G. Cardillo, M. Orena, G. Porzi, and S. Sandri, Tetrahedron, 1987, 43, 4377; CrossRef (c) K. Kawabata, Y. Inamoto, K. Sakane, T. Iwamoto, and S. Hashimoto, J. Antibiot., 1990, 43, 513; CrossRef (d) H. Ohki, G. Cashiragi, L. Colombo, G. Rassu, and P. Spanu, J. Org. Chem., 1991, 56, 6523; CrossRef (e) D. C. Cole, Tetrahedron, 1994, 50, 9517; CrossRef (f) F. A. Davis, R. T. Reddy, and R. E. Reddy, J. Org. Chem., 1992, 57, 6387; CrossRef (g) J. Jiang, K. K. Schumacher, M. M. Joullie, F. A. Davis, and R. E. Reddy, Tetrahedron Lett., 1994, 35, 2121. CrossRef
2. For reviews of polyamine alkaloids, see: (a) U. Bachrach, ‘Function of Naturally Occuring Polyamines’, Academic press, New York, 1973; (b) M. Hesse and N. Schmid, ‘Macrocyclic Spermidine and Spermine Alkaloids’, Int Reviews of Science, ed. by H. D. Hey and K. Wiesner, Butterworth, London, 1976, Organic Chemistry Series II, Vol. 9, p. 265; (c) M. M. Badawi, K. Bernauer, P. Van den Broek, D. Groger, A. Gueggisberg, S. Johne, I. Kompis, F. Schneider, H.-J. Veith, M. Hesse, and H. Schmid, Pure Appl. Chem., 1973, 33, 81; CrossRef (d) H. H. Wasserman and J. S. Wu, Heterocycles, 1982, 17, 581; CrossRef (e) R. A. Campbell, D. R. Morris, and D. Bartos, ‘Advances in Polyamine Research’, Raven press, New York, 1978, Vols. 1 and 2; (f) B. Ganem, Acc. Chem. Res., 1982, 15, 290; CrossRef (g) H. H. Wasserman and R. P. Robinson, Heterocycles, 1984, 21, 279. CrossRef
3. For polyamine alkaloids, see: (a) P. Kuehne, A. Linden, and M. Hesse, Helv. Chim. Acta, 1996, 79, 1085; CrossRef (b) K. Schultz and M. Hesse, Helv. Chim. Acta, 1996, 79, 1295; CrossRef (c) U. A. Hausermann, A. Linden, J. Song, and M. Hesse, Helv. Chim. Acta, 1996, 79, 1995; CrossRef (d) P. Kuehne, A. Guggisberg, and M. Hesse, Helv. Chim. Acta, 1997, 80, 1802; CrossRef (e) A. Horni, A. Linden, and M. Hesse, Helv. Chim. Acta, 1998, 81, 1303; CrossRef (f) K. Drandarov, A. Guggisberg, A. Linden, and M. Hesse, Helv. Chim. Acta, 1998, 81, 1773; CrossRef (g) L. Nezbedova, K. Drandarov, C. Werner, and M. Hesse, Helv. Chim. Acta, 2000, 83, 2953; CrossRef (h) A. Guggisberg, K. Drandarov, and M. Hesse, Helv. Chim. Acta, 2000, 83, 3035. CrossRef
4. (a) Y. Yamamoto, N. Asao, and T. Uyehara, J. Am. Chem. Soc., 1992, 114, 5427; CrossRef (b) S. G. Davies, A. C. Garner, R. L. Nicholson, J. Osborne, E. D. Savory, and A. D. Smith, Chem. Commun., 2003, 2134; CrossRef (c) T. Sakai, H. Doi, Y. Kawamoto, K. Yamada, and K. Tomioka, Tetrahedron Lett., 2004, 45, 9261. CrossRef
5. (a) W. Oppolzer, C. Chapuis, and G. Bernardinelli, Helv. Chim. Acta, 1984, 67, 1397; CrossRef (b) W. Oppolzer, Tetrahedron, 1987, 43, 1969; CrossRef (c) W. Oppolzer, Tetrahedron, 1987, 43, 4057; CrossRef (d) W. Oppolzer, Pure Appl. Chem., 1990, 62, 1241. CrossRef
6. For spermidine alkaloid (S)-(–)-celacinnine, see: (a) H. Matsuyama, N. Itoh, A. Matsumoto, N. Ohira, K. Hara, M. Yoshida, and M. Iyoda, J. Chem. Soc., Perkin Trans. 1, 2001, 2924; CrossRef (b) Y. Kuroki, K. Ishihara, N. Hanaki, S. Ohara, and H. Yamamoto, Bull. Chem. Soc. Jpn., 1998, 71, 1221; CrossRef For (+)-celacinnine, see: (c) J. S. McManis and B. Ganem, J. Org. Chem., 1980, 45, 2041; CrossRef (d) H. H. Wasserman, R. P. Robinson, and H. Matsuyama, Tetrahedron Lett., 1980, 21, 3493; CrossRef (e) H. Yamamoto and K. Maruoka, J. Am. Chem. Soc., 1981, 103, 6133; CrossRef For (+)-dihydroperiphylline, see: (f) H. Matsuyama, A. Kurosawa, T. Takei, N. Ohira, M. Yoshida, and M. Iyoda, Chem. Lett., 2000, 1104; CrossRef (g) T. Kaseda, T. Kikuchi, and C. Kibayashi, Tetrahedron Lett., 1989, 30, 4539; CrossRef (h) K. Ishihara, Y. Kuroki, and H. Yamamoto, Synlett, 1995, 41. CrossRef
7. (a) N. Itoh, H. Matsuyama, M. Yoshida, N. Kamigata, and M. Iyoda, Heterocycles, 1995, 41, 415; CrossRef (b) H. H. Wasserman, R. P. Robinson, and H. Matsuyama, Tetrahedron Lett., 1980, 21, 3493; CrossRef (c) H. Matsuyama, M. Kobayashi, and H. H. Wasserman, Heterocycles, 1987, 26, 85; CrossRef (d) M. J. Begley, L. Crombie, D. Haigh, R. C. F. Jones, S. Osborn, and R. A. B. Webster, J. Chem. Soc., Perkin Trans. 1, 1993, 2027. CrossRef
8. (a) M. Pais, R. Sarfati, and F.–X. Jarreau, Bull. Soc. Chim. Fr., 1973, 331; (b) M. Pais, R. Sarfati, F.–X. Jarreau, and R. Goutarel, Tetrahedron, 1973, 29, 1001; CrossRef and references cited their in; natural homaline, [α]D24 –34° (c 1, CHCl3). For the synthesis of (S,S)-homaline, see: (c) N. Itoh, H. Matsuyama, M. Yoshida, N. Kamigata, and M. Iyoda, Bull. Chem. Soc. Jpn., 1995, 68, 3121; CrossRef (d) H. H. Wasserman, G. D. Berger, and K. R. Cho, Tetrahedron Lett., 1982, 23, 465; CrossRef (e) H. H. Wasserman and G. D. Berger, Tetrahedron, 1983, 39, 2459; CrossRef (f) L. Crombie, R. C. F. Jones, Ab. Rasid Mat-Zin, and S. Osborne, J. Chem. Soc., Chem. Commun., 1983, 960; CrossRef (g) L. Crombie, D. Haigh, R. C. F. Jones, and Ab. Rasid Mat-Zin, J. Chem. Soc., Perkin Trans. 1, 1993, 2047. CrossRef
9. (a) W. Oppolzer and J.-P. Barras, Tetrahedron, 1986, 42, 4035; CrossRef (b) D. P. Curran and T. A. Heffner, J. Org. Chem., 1990, 55, 4585; CrossRef (c) X. Cao, F. Liu, W. Lu, G. Chen, G.-A. Yu, and S. H. Liu, Tetrahedron, 2008, 64, 5629; CrossRef (d) S. Hajra, M. Bhowmick, and D. Sinha, J. Org. Chem., 2006, 71, 9237. CrossRef
10. Conjugate addition of lithium amide of 6-membered hydrazine 4 to (1S)-3 (Table 2, entry 3): (1S)-3: white solid (recrystallized from n-hexane), mp 186-187 °C; [α]D24 –98° (c 1.02, CHCl3). To the anhydrous Et2O (5 mL) solution of 4 (5.0 mmol) was added dropwise n-BuLi (1.6M in n-hexane, 3.2 mL, 5.0 mmol) at 0 °C under nitrogen and the solution was stirred for 30 min. Then the anhydrous Et2O (5 mL) solution of (1S)-3 (345 mg, 1.0 mmol) was added and the solution was stirred at 0 °C for 24 h, and then at room temperature for 24 h. To this solution was added ammonium chloride (200 mg) and then water was added. The product was extracted with AcOEt and the organic layer was dried (Na2SO4). After purification of crude product by flash column chromatography on silica gel (50% AcOEt in n-hexane), (S)-1 was obtained in 83% yield (180 mg, 0.83 mmol) with 86% ee and (1S)-5 in 96% yield (207 mg, 0.96 mmol). (S)-1: oil; Rf = 0.10 (AcOEt/n-hexane = 2/1); [α]D24 –140° (c 0.50, CHCl3, 86% ee), lit.,6a [α]D24 –167° (c 1.30, CHCl3, 99% ee). IR (CHCl3) 1677 cm-1 (C=O); 1H NMR [ppm] (500 MHz, CDCl3, 20 °C): δ 1.37-1.48 (1H, m, 4-CH), 1.59-1.67 (1H, m, 4-CH), 1.70-1.79 (2H, m, 3-CH2), 2.26 (1H, td, J = 11.2, 2.4 Hz, 2-CH), 2.52 (1H, dd, J = 16.4, 11.4 Hz, 8-CH), 2.88 (1H, dd, J = 16.4, 8.8 Hz, 8-CH), 2.98 (1H, dd, J = 12.4, 11.6 Hz, N(CO)CH, 5-CH), 3.06 (1H, d, J = 10.0 Hz, 2-CH), 3.85 (1H, dd, J = 11.2, 8.3 Hz, PhCH, 9-CH), 4.17 (1H, d, J = 11.2 Hz, C(O)NCH, 5-CH), 7.29-7.41 (5H, m, Ph); 13C NMR [ppm] (125 MHz, CDCl3, 20 °C): δ 22.7 (4-C), 23.9 (3-C), 39.9 (8-C), 42.0 (5-C), 55.4 (2-C), 67.4 (9-C), 128.1 (p-phenyl-C), 127.5 and 128.7 (o- and m-phenyl-C), 139.0 (phenyl C), 168.5 (C=O); m/z 216 (M+); HRMS Calcd for C13H16N2O: M, 216.1263. Found: m/z, 216.1273 (M+).
11. The conjugate addition of 5-membered hydrazine 6 (5.0 mmol) to (1S)-3 (1.0 mmol) was investigated (Table 2, entry 8): purification by flash column chromatography on silica gel (10% MeOH in CHCl3) gave (S)-2 in 90% yield (182 mg, 0.90 mmol) with 77% ee and (1S)-5 in 94% yield (202 mg, 0.94 mmol). (S)-2: oil; Rf= 0.49 (CHCl3/MeOH = 19/1); [α]D24 –163° (c 1.23, CHCl3, 77% ee), lit.,8c [α]D24 –177o (c 1.90, CHCl3, 87% ee); IR (CHCl3) 1650 cm-1 (C=O); 1H NMR [ppm] (500 MHz, CDCl3, 20 °C): δ 2.32-2.42 (2H, m, 7-CH), 2.44 (1H, q, J = 8.3 Hz, 6-CH), 2.97 (2H, d, J = 8.3 Hz, 3-CH2), 3.21 (1H, t, J = 8.3 Hz, 6-CH), 3.35 (1H, ddd, J = 11.2, 8.3, 4.9 Hz, N(CO)CH, 8-CH), 3.75 (1H, dt, J = 11.2, 7.8 Hz, N(CO)CH, 8-CH), 4.11 (1H, t, J = 9.8 Hz, PhCH, 4-CH), 7.25-7.45 (5H, m, Ph); 13C NMR [ppm] (125 MHz, CDCl3, 20 °C): δ 27.5 (7-C), 23.9 (3-C), 39.5 (8-C), 43.9 (3-C), 53.3 (6-C), 69.0 (4-C), 126.9 (p-phenyl-C), 128.1 and 128.8 (o- and m-phenyl-C), 139.1 (phenyl C), 165.9 (C=O); m/z 202 (M+); HRMS Calcd for C12H14N2O: M, 202.1106. Found: m/z 202.1079 (M+).
12. (1S)-7a (R = OMe): mp 148-149 °C, [α]D25 –93° (c 0.50, CHCl3); (1S)-7b (R = NO2): mp 243-245 °C, [α]D23 –93° (c 0.37, CHCl3); (1S)-7c (R = Me): mp 205-206 °C, [α]D25 –97° (c 0.50, CHCl3); (1S)-7d (R = F): mp 188-191 °C, [α]D23 –96° (c 0.50, CHCl3); (1S)-7e (R= Cl): mp 211-213 °C, [α]D23 –88° (c 0.50, CHCl3); (S)-8a (R = OMe): [α]D25 –110° (c 1.03, CHCl3; 64% ee); (S)-8b (R = NO2): [α]D23 –180° (c 0.38, CHCl3; 86% ee); (S)-8c (R = Me): [α]D25 –133° (c 0.78, CHCl3; 64% ee); (S)-8d (R = F): [α]D23 –121° (c 1.02, CHCl3; 56% ee); (S)-8e (R = Cl): [α]D23 –113° (c 0.97, CHCl3; 50% ee)..
13. The X-ray analysis of N-crotonoyl (1S)-2,10-camphorsultam has been reported: W. Oppolzer, I. Rodriguez, J. Blagg, and G. Bernardinelli, Helv. Chim. Acta, 1989, 72, 123. CrossRef