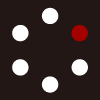
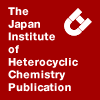
HETEROCYCLES
An International Journal for Reviews and Communications in Heterocyclic ChemistryWeb Edition ISSN: 1881-0942
Published online by The Japan Institute of Heterocyclic Chemistry
e-Journal
Full Text HTML
Received, 27th February, 2012, Accepted, 26th March, 2012, Published online, 3rd April, 2012.
DOI: 10.3987/COM-12-12457
■ Facile Synthesis of 2,3-Disubstituted Pyrroles from 2-Substituted 1-Pyrrolines
Yurie Fujiwara, Mitsunori Oda,* Taku Shoji, Takako Abe, and Shigeyasu Kuroda
Department of Chemistry, Faculty of Science, Shinshu University, Asahi, Matsumoto 390-8621, Japan
Abstract
2-Substituted-1-pyrrolines react with various arylaldehyde acetals in the presence of a Lewis acid and base to give 2-substituted 3-arylmethylidene-1-pyrrolines, which are transformed to 2,3-disubstituted pyrroles by base-catalyzed double-bond isomerization.Pyrrole derivatives are an important family of compounds especially because of their significance in the synthesis of natural products and pharmaceuticals.1 In addition to reliable classical methods such as the Knorr,2 Paal–Knorr,3 and Hantzsch syntheses,4 synthetic methodologies for pyrroles have been continuously developed.5 Although the development of nitrogen-containing five-membered rings is a recent topic of interest, synthetic routes for pyrroles by inexpensive or easily available hydrogenated materials such as pyrrolines and pyrrolidines are also still important.6 Previously, we reported the synthesis of 1,3-disubstituted pyrroles 1 simply by heating pyrrolidine (2) and aldehydes 3 without any catalyst in a pressure vessel, as shown in Scheme 1.7 In this paper, we describe a new facile synthesis of 2,3-disubstituted pyrroles 4 from 2-substituted 1-pyrrolines 5 with acetals 6 by a two-step sequence, which involves an aldol-type condensation followed by base-catalyzed double-bond isomerization.
For the first step, we found that a conventional aldol-type condensation8 of 2-phenyl-1-pyrroline (5a)9 with benz-aldehyde under continuous azeotropic distillation of water provided 3-benzylidene-2-phenyl- 1-pyrroline (7a) in 73% yield.10,11 However, it took a very long time (175 h) to complete the reaction (Scheme 2).
Then, we examined reactions with benzaldehyde dimethyl acetal assisted by a Lewis acid and triethyamine12 (Table 1). The results indicate that the yield depends on the Lewis acid used. Among the Lewis acids used, TiCl4 was the most effective, giving the most satisfactory yield of 7a (Table 1, entry 3). This reaction proceeded much faster than the conventional method.
Several 2-substituted 1-pyrrolines were subjected to condensation with some acetals under these conditions. The results are summarized in Table 2. Benzaldehyde diethyl acetal reacted similarly, but the reaction proceeded slightly slower, giving 7a in a similar yield (Table 2, entry 1). Various dimethyl acetals such as p-bromobenzaldehyde, o-methylbenzaldehyde, 1-naphthylcarbaldehyde, and 2-thienylcarbaldehyde dimethyl acetals react with 5a to give 7b–7e (Table 2, entry 2–5), respectively, and the yield mainly depends on the structure of the aryl group. Using alkanal dimethyl acetals, such as acetaldehyde and propanal dimethyl acetals, did not form the expected condensation products. None of the dimethyl ketals – acetone, benzophenone, acetophenone, and cyclohexane dimethyl ketals – react with 5a under these conditions. Reactions of other 2-(substituted phenyl)-1-pyrrolines with 6a provide satisfactory yields of 7f–g (Table 2, entries 6 and 7, respectively). However, 7h was obtained in low yield (Table 2, entry 8) probably because of the sterically bulky t-Bu group. Moreover, 2-(methoxycarbonyl)-1- pyrroline was found to be inactive under these conditions (Table 2, entry 9). We propose the following reaction mechanism, shown in Scheme 3, to explain the change of product yields. A reaction of TiCl4 and triethylamine with 4 produces the enamine 9, which then reacts with the methyloxonium ion 8 generated in situ to yield the adduct 10.13 The elimination of methanol subsequently forms the final product 7. The reason why alkanal dimethyl acetals does not give the expected product may be ascribed to that the methyloxonium ion derived from alkanal dimethyl acetals undergoes elimination of proton to give the corresponding methylvinyl ether, which is observed in the crude reaction mixture accompanied with the starting material 4.
Then, the second step, which is the base-catalyzed double-bond isomerization of pyrroline 7 to the corresponding pyrrole 4, was examined. The results under various conditions with several bases are
shown in Table 3. t-BuOK in DMSO worked efficiently as a catalyst for double-bond isomerization to give 4a in high yield (Table 3, entry 5), though the reactions at elevated temperatures and with a larger amount of the t-BuOK resulted in lower yields (Table 3, entries 4 and 6, respectively). Under optimal conditions with t-BuOK/DMSO, other pyrrolines 7 were also converted to the corresponding pyrroles in good-to-high yields (Table 4, entry 1–5). However, compounds with the strong electron-donating groups at the 2 position in 7 gave 4 in low yields (Table 4, entries 6 and 7). Therefore, the isomerization must proceed through repeated deprotonation and protonation steps. The electron-donating groups may reduce the acidity of the C–H bonds of the tetrahedral carbons of 7, and consequently decrease the yield of these reactions.
We have demonstrated transformation of 2-substituted 1-pyrrolines to 2,3-disubstituted pyrroles in two steps, which involve condensation with acetals assisted by a combination of TiCl4 and triethylamine, followed by the double-bond isomerization catalyzed by t-BuOK in DMSO. Although limited acetals can be employed in the condensation step, the reactions were completed in a reasonably short time. The results suggest a new facile synthetic method for 2,3-disubstituted pyrroles, which can be applied to the synthesis of bioactive compounds such as pharmaceuticals.
EXPERIMENTAL
Melting points were measured on a Yanaco MP-3. IR spectra were recorded on JEOL Diamond-20 and JASCO FT/IR-4100 spectrometers. UV spectra were measured on a Shimadzu UV-2550 spectrometer. 1H and 13C-NMR spectra were recorded with tetramethylsilane as internal standard on JEOL λ400 and ECA500 NMR instruments. Mass spectra were measured on a JMS-700 mass spectrometer. Column chromatography was done with Silica gel 60N from Kanto Chem., Inc. Trimethylsilyl triflate, titanium tetrachloride, stannic tetrachloride, benzaldehyde acetals, t-BuOK, and borontrifluoride-ether complex were purchased from Tokyo Kasei Industrial Co. 2-Phenyl- and 2-(4-methoxyphenyl)-1-pyrrolines were synthesized from N-vinylpyrrolidin-2-one by the method of Sorgi et al.9f 2-(4-Dimethylaminophenyl)-, and 4-t-butyl-1-pyrrolines were prepared from 2-methoxy-1-pyrroline according to a literature procedure.9d
3-Benzylidene-2-phenyl-1-pyrroline (7a) from 5a and benzaldehyde
A mixture of 5a (1.06 g, 7.27 mM), benzaldehyde (0.763 g, 7.19 mM), p-toluenesulfonic acid (72 mg, 0.42 mM) in 50 mL of benzene was charged in a 100-mL flask, which was connected with a Dean-Stark trap to remove water continuously. The mixture was refluxed on an oil bath for 175 h to completion of the reaction. The resulted mixture was washed with a saturated aqueous NaHCO3 solution (100 mL) and brine, and then, was dried over MgSO4. After evaporation of the solvent, the residue was purified by silica gel column chromatography to give 1.31 g (73% yield) of 7a as colorless needles. Mp 110–112 ˚C (lit., 110–111˚C10a and 108–109˚C10b). 1H NMR (CDCl3) δ = 3.09 (dt, J = 5.6, 3.2 Hz, 2H), 4.22 (t, J = 5.6 Hz, 2H), 6.84 (t, J = 3.2 Hz, 1H), 7.30 (t, J = 7.2 Hz, 1H), 7.39 (t, J = 7.6 Hz, 2H), 7.44 (t, J = 7.2 Hz, 2H), 7.44–7.49 (m, 3H), 7.65 (m, 2H) ppm; 13C NMR (CDCl3) δ = 30.9, 59.6, 127.89, 127.93, 128.47, 128.58, 128.8, 128.9, 129.6, 134.5, 134.0, 142.1, 175.0 ppm.
A typical procedure of Lewis acid ad triethylamine-assisted condensation of 2-substituted 1-pyrrolines with dialkoxymethylarenes
To a solution of 5 (1.00 mM), triethylamine (417 μL, 3.00 mM), dimethyl acetal (2.00 mM) in solvent (10 mL) at 0 ˚C was added dropwise Lewis acid (3.00 mM). Then, the mixture was heated in an oil bath under nitrogen atmosphere using a balloon. The reaction was monitored by TLC analysis and the resulted reaction mixture was poured into a saturated aqueous NaHCO3 solution (50 mL). The solids formed were removed by filtration and the filtrate was extracted three times with 20 mL of chloroform. The combined organic layer was washed with brine and was dried over MgSO4. The solvent was removed and the residue was purified by silica gel chromatography to give the product.
3-(4-Bromobenzylidene)-2-phenyl-1-pyrroline (7b); Brown needles, mp 83–86 ˚C. 1H NMR (CDCl3) δ = 3.03 (dt, J = 5.8, 2.8 Hz, 2H), 4.23 (t, J = 5.8 Hz, 2H), 6.76 (t, J = 2.8 Hz, 1H), 7.28 (d, J = 8.8 Hz, 2H), 7.46–7.52 (m, 5H), 7.62–7.64 (m, 2H) ppm; 13C NMR (CDCl3) δ = 30.9, 59.7, 121.8, 126.5, 128.5, 128.7, 129.7, 130.3, 131.7, 134.3, 136.0, 142.8, 174.8 ppm; UV (EtOH) λmax = 227 (logε = 4.03), 235 (4.01), 252sh (4.02), 287sh (4.40), 295 (4.19), 300 (4.48), 312 (4.35) nm; MS (70 eV): m/z (rel int) 313 (M+, 98), 312(58), 311 (M+, 100), 310 (40), 285 (11), 283 (12), 232 (18), 231 (14), 230 (13), 204 (18), 203 (21), 202 (14), 156 (25), 129 (47), 128 (32), 127 (14), 117 (47), 101 (11), 91 (16), 77 (21). Anal. Calcd for C17H14BrN: C, 65.40; H, 4.52; N, 4.49%. Found C, 65.24; H, 4.62; N, 4.33%.
3-(2-Methybenzylidene)-2-phenyl-1-pyrroline (7c); Brown solids, mp 80–84 ˚C. 1H NMR (CDCl3) δ = 2.21 (s, 3H), 2.98 (dt, J = 6.3, 2.8 Hz, 2H), 4.15 (t, J = 6.3 Hz, 2H), 6.98 (t, J = 2.8 Hz, 1H), 7.17–7.24 (m, 3H), 7.44–7.48 (m, 4H), 7.66–7.70 (m, 2H) ppm; 13C NMR (CDCl3) δ = 20.0, 30.6, 59.1, 125.5, 125.8, 127.3, 127.8, 128.4, 128.7, 129.6, 130.2, 134.4, 135.9, 136.9, 142.5, 174.6 ppm; UV (EtOH) λmax = 229 (logε = 4.03), 236 (4.03), 248 (4.01), 287sh (4.18), 295 (4.19), 318sh (3.83) nm; MS (70 eV): m/z (rel int) 247 (M+, 100), 246 (41), 232 (20), 219 (11), 156 (18), 129 (33), 128 (22), 117 (26), 116(10), 115 (18), 91 (11), 77 (14). HRMS m/z Calcd for C18H17N (M+) 247.1361, found: 247.1356.
3-(1-Naphthylmethylidene)-2-phenyl-1-pyrroline (7d); Brown microcrystals, mp 119–120 ˚C. 1H NMR (CDCl3) δ = 3.00 (dt, J = 6.3, 2.5 Hz, 2H), 4.16 (t, J = 6.3 Hz, 2H), 7.46 (t, J = 2.5 Hz, 1H), 7.43–7.52 (m, 6H), 7.63 (d, J = 7.5 Hz, 1H), 7.76–7.81 (m, 3H), 7.85 (dt, J = 8.0, 2.0 Hz, 2H) ppm; 13C NMR (CDCl3) δ = 31.0, 59.0, 123.9, 124.5, 125.2, 125.5, 125.9, 126.2, 128.3, 128.5, 128.6, 128.8, 129.8, 131.5, 133.6, 134.0, 134.3, 144.1, 174.2 ppm; UV (EtOH) λmax = 225 (logε = 4.52), 267sh (3.98), 319sh (4.13), 326 (4.13), 346sh (3.91), 393sh (2.56) nm; MS (70 eV): m/z (rel int) 283 (M+, 100), 282 (50), 180 (13), 179 (22), 178 (14), 118 (11), 117 (22). HRMS m/z Calcd for C21H17N (M+) 283.1361, found: 283.1360.
2-Phenyl-3-(2-thienylmethylidene)-1-pyrroline (7e); Brown microcrystals, mp 95–96 ˚C. 1H NMR (CDCl3) δ = 3.02 (dt, J = 6.0, 2.8 Hz, 2H), 4.26 (t, J = 6.0 Hz, 2H), 7.05 (t, J = 2.8 Hz, 1H), 7.08 (dd, J = 5.0, 3.5 Hz, 1H), 7.12 (d, J = 3.5 Hz, 1H), 7.39 (d, J = 5.0 Hz, 1H), 7.45–7.48 (m, 3H), 7.59–7.62 (m, 2H) ppm; 13C NMR (CDCl3) δ = 31.1, 59.7, 120.3, 126.8, 127.5, 128.4, 128.5, 128.9, 129.5, 134.4, 140.0, 141.6, 174.2 ppm; UV (EtOH) λmax = 254 (logε = 3.91), 316 (4.39), 329sh (4.33), 377 (2.29) nm. MS (70 eV): m/z (rel int) 239 (M+, 100), 238 (53), 211 (21), 135 (12), 117 (18), 77 (11). HRMS m/z Calcd for C15H13NS (M+) 239.0769, found: 239.0772.
3-Benzylidene-2-(4-methoxyphenyl)-1-pyrroline (7f); Brown prisms, mp 142–144 ˚C. 1H NMR (CDCl3) δ = 3.06 (dt, J = 5.6, 2.8 Hz, 2H), 3.86 (s, 3H), 4.18 (t, J = 5.6 Hz, 2H), 6.86 (t, J = 2.8 Hz, 1H), 6.99 (dt, J = 8.8, 2.8 Hz, 2H), 7.29 (t, J = 7.2 Hz, 1H), 7.41 (m, 4H), 7.63 (dt, J = 8.8, 2.8 Hz, 2H) ppm; 13C NMR (CDCl3) δ = 31.1, 55.4, 59.3, 113.9, 126.9, 127.5, 127.8, 128.6, 128.9, 130.2, 137.1, 142.2, 160.8, 174.3 ppm; UV (EtOH) λmax = 222 (logε = 4.16), 224sh (4.15), 230sh (4.06), 282sh (4.36), 290sh (4.37), 304sh (4.22) nm; MS (70 eV): m/z (rel int) 263 (M+, 100), 262 (42), 232 (37), 186 (12), 147 (19), 129 (12). Anal. Calcd for C18H17NO: C, 82.10; H, 6.51; N, 5.32%. Found C, 82.14; H, 6.45; N, 5.22%.
3-Benzylidene-2-(4-dimethylaminophenyl)-1-pyrroline (7g); Brown microcrystals, mp 125–126 ˚C. 1H NMR (CDCl3) δ = 3.03 (s, 6H), 3.04 (dt, J = 5.8, 3.0 Hz, 2H), 4.14 (t, J = 5.8 Hz, 2H), 6.77 (d, J = 8.7 Hz, 2H), 6.94 (t, J = 3.0 Hz, 1H), 7.27 (t, J = 7.3 Hz, 1H), 7.37 (t, J = 7.3 Hz, 2H), 7.43 (d, J = 7.3 Hz, 2H), 7.60 (d, J = 8.7 Hz, 2H) ppm; 13C NMR (CDCl3) δ = 31.4, 40.5, 59.1, 111.8, 122.0, 127.5, 127.7, 128.6, 129.0, 130.1, 137.5, 142.5, 151.5, 174.4 ppm; UV (EtOH) λmax = 223 (logε = 4.08), 232sh (4.01), 270sh (4.39), 293 (4.45), 306 (4.35), 328 (3.96) nm; MS (70 eV): m/z (rel int) 276 (M+, 100), 275 (46), 248 (22), 199 (14), 138 (12), 129 (11), 128 (11), 114 (12). Anal. Calcd for C19H20N2·0.2H2O: C, 81.51; H, 7.34; N, 10.01%. Found C, 81.60; H, 7.44; N, 10.22%.
3-Benzylidene-2-t-butyl-1-pyrroline (7h); A brown oil. 1H NMR (CDCl3) δ = 1.41 (s, 9H), 2.90 (dt, J = 5.8, 3.0 Hz, 2H), 3.95 (t, J = 5.8 Hz, 2H), 7.04 (t, J = 3.0 Hz, 1H), 7.27 (t, J = 7.5 Hz, 1H), 7.38 (t, J = 7.5 Hz, 2H), 7.43 (d, J = 7.5 Hz, 2H) ppm; 13C NMR (CDCl3) δ = 29.5, 31.8, 36.2, 58.0, 126.7, 127.6, 128.6, 129.0, 137.6, 141.0, 181.0 ppm; UV (EtOH) λmax = 277 (logε = 4.28), 286 (4.31), 303 (4.09), 330 (2.91) nm; MS (70 eV): m/z (rel int) 213 (M+, 62), 212 (55), 198 (100), 188 (14), 172 (10), 171 (38), 170 (47), 155 (17), 136 (22), 116 (16), 115 (33), 105 (21), 91 (19). HRMS m/z Calcd for C15H19N (M+) 213.1518, found: 213.1507.
A typical procedure of t-BuOK-catalyzed double-bond isomerization of 7 to 4
A mixture of 7 (0.500 mmol), t-BuOK (33 mg, 0.50 mmol) in DMSO (2 mL) was stirred at room temperature under argon atmosphere for 3–7 h. The reaction mixture was poured into cold water and was neutralized by 0.1 N HCl, and then extracted with Et2O (15 mL x 3). The combined organic layer was washed with brine and was dried over MgSO4. After removal of the solvent, the residue was purified by silica gel chromatography to give the product. Analytical samples were obtained by recrystallization from EtOAc-hexane. These pyrroles were colorless or creamy white just after chromatographic purification. However, the samples were colorized during the recrystallization, as often seen in other pyrroles.
3-Benzyl-2-phenylpyrrole (4a); Purple prisms, mp 107–108 ˚C. 1H NMR (CDCl3) δ = 4.01 (s, 2H), 6.09 (t, J = 2.8 Hz, 1H), 6.80 (t, J = 2.8 Hz, 1H), 7. 12 (tt, J = 7.2, 1.2 Hz, 1H), 7.22-7.30 (m, 5H), 7.34-7.39 (m, 4H), 8.16 (s, 1H) ppm; 13C NMR (CDCl3) δ = 32.6, 111.6, 117.8, 119.3, 125.7, 126.5, 126.8, 128.4, 128.6, 128.8, 129.0, 133.4, 142.3 ppm; IR (KBr) νmax = 3404vs, 3396vs, 761s, 710s, 694s cm–1; UV (EtOH) λmax = 206 (logε = 4.57), 287 (4.17) nm; MS (70 eV): m/z (rel int) 233 (M+, 100), 232 (44), 156 (96), 154 (12), 128 (11). Anal. Calcd for C17H15N: C, 87.52; H, 6.48; N, 6.00%. Found C, 87.33; H, 6.49; N, 5.92%.
3-(4-Bromobenzyl)-2-phenylpyrrole (4b); Red needles, mp 55–58 ˚C. 1H NMR (CDCl3) δ = 3.95 (s, 2H), 6.07 (t, J = 2.4 Hz, 1H), 6.82 (t, J = 2.4 Hz, 1H), 7. 09 (d, J = 8.4 Hz, 2H), 7.34-7.40 (m, 7H), 8.19 (s, 1H) ppm; 13C NMR (CDCl3) δ = 32.0, 111.5, 117.9, 118.6, 119.5, 126.6, 126.8, 128.9, 129.1, 130.3, 131.4, 133.3, 141.3 ppm; IR (KBr) νmax = 3388vs, 1485s, 1011s, 772s, 734s cm–1; UV (EtOH) λmax = 202 (logε = 4.52), 221sh (4.27), 284 (4.15) nm; MS (70 eV): m/z (rel int) 313 (M+, 98), 311 (M+, 100), 232 (19), 230 (13), 156 (100), 154 (17), 128 (13). Anal. Calcd for C17H14BrN: C, 65.40; H, 4.52; N, 4.49%. Found C, 65.42; H, 4.62; N, 4.41%.
3-(2-Methylbenzyl)-2-phenylpyrrole (4c); Brown microcrystals, mp 132–133 ˚C. 1H NMR (CDCl3) δ = 2.24 (s, 3H), 3.94 (s, 2H), 5.98 (t, J = 3.0 Hz, 1H), 6.79 (t, J = 3.0 Hz, 1H), 7.10–7.16 (m, 4H), 7.24 (m, 1H), 7.34–7.38 (m, 4H), 8.18 (s, 1H) ppm; 13C NMR (CDCl3) δ = 19.7, 30.5, 111.7, 117.8, 118.9, 125.98, 126.03, 126.5, 126.7, 128.9, 129.0, 130.0, 133.6, 136.4, 140.4, 144.0 ppm; IR (ATR) νmax = 3380s, 763s, 731vs, 694s cm–1; UV (EtOH) λmax = 286 (logε = 4.15) nm; MS (70 eV): m/z (rel int) 247 (M+, 100), 246 (21), 232 (11), 156 (40), 143 (68). HRMS m/z Calcd for C18H17N (M+) 247.1361, found: 247.1356.
3-(1-Naphthylmethy)-2-phenylpyrrole (4d); Brown microcrystals, mp 124–126 ˚C. 1H NMR (CDCl3) δ = 4.44 (s, 2H), 6.01 (t, J = 2.9 Hz, 1H), 6.80 (t, J = 2.9 Hz, 1H), 7.24 (tt, J = 7.2, 1.2 Hz, 1H), 7.31 (dd, J = 6.8, 1.2 Hz, 1H), 7.33–7.37 (m, 2H), 7.39–7.41 (m, 2H), 7.44–7.47 (m, 3H), 7.73 (d, J = 8.0 Hz, 1H), 7.86 (dd, J = 7.5, 2.0 Hz, 1H), 8.01 (dd, J = 7.5, 2.0 Hz, 1H), 8.23 (s, 1H) ppm; 13C NMR (CDCl3) δ = 30.0, 111.8, 117.8, 118.7, 124.0, 125.4, 125.7, 125.8, 126.0, 126.4, 126.6 (2C), 128.6, 128.85, 128.90, 132.1, 133.4, 133.7, 137.9 ppm; IR (ATR) νmax = 3437vs, 803s, 783vs, 767vs, 696s cm–1; UV (EtOH) λmax = 273sh (logε = 4.23), 283 (4.32), 295sh (4.24) nm; MS (70 eV): m/z (rel int) 283 (M+, 100), 282 (51), 155 (48). HRMS m/z Calcd for C21H17N (M+) 283.1361, found: 283.1357.
2-Phenyl-3-(2-thienylmethy)pyrrole (4e); Brown microcrystals, mp 75–76 ˚C. 1H NMR (CDCl3) δ = 4.15 (d, J = 1.0 Hz, 2H), 6.22 (t, J = 3.0 Hz, 1H), 6.81-6.83 (m, 2H), 6.92 (dd, J = 5.0, 3.5 Hz, 1H), 7.12 (dd, J = 5.0, 1.0 Hz, 1H), 7.27 (tt, J = 7.2, 1.2 Hz, 1H), 7.37–7.41 (m, 4H), 8.16 (s, 1H) ppm; 13C NMR (CDCl3) δ = 27.1, 111.3, 117.7, 118.9, 123.2, 124.3, 126.6, 126.7, 126.9, 128.8, 128.9, 133.1, 146.1 ppm; IR (ATR) νmax = 3406s, 3397s, 763s, 690vs cm–1; UV (EtOH) λmax = 227 (logε = 4.13), 284 (4.14) nm; MS (70 eV): m/z (rel int) 239 (M+, 100), 238 (75), 162 (12), 154 (14). HRMS m/z Calcd for C15H13NS (M+) 239.0769, found: 239.0773.
3-Benzyl-2-(4-methoxyphenyl)pyrrole (4f); Blue needles, mp 78–79 ˚C. 1H NMR (CDCl3) δ = 3.81 (s, 2H), 3.96 (s, 3H), 6.08 (t, J = 2.8 Hz, 1H), 6.77 (t, J = 2.8 Hz, 1H), 6.92 (d, J = 8.4 Hz, 2H), 7.16–7.31 (m, 7H), 8.08 (s, 1H) ppm; 13C NMR (CDCl3) δ = 32.0, 55.3, 111.3, 114.2, 117.1, 118.4, 125.7, 126.2, 128.3, 128.3, 128.5, 128.9, 142.5, 158.4 ppm; IR (KBr) νmax = 3359s, 3345s, 1509vs, 1254s, 1030s, 708s cm–1; UV (EtOH) λmax = 268sh (logε = 4.19), 276 (4.23) nm; MS (70 eV): m/z (rel int) 263 (M+, 100), 262 (32), 248 (8), 218 (5), 186 (57). Anal. Calcd for C18H17NO·0.05H2O: C, 81.82; H, 6.52; N, 5.30%. Found C, 81.74; H, 6.39; N, 5.30%.
3-Benzyl-2-(4-dimethylaminophenyl)pyrrole (4g); Blue microcrystals, mp 108–111 ˚C. 1H NMR (CDCl3) δ = 2.95 (s, 6H), 3.96 (s, 2H), 6.05 (t, J = 2.5 Hz, 1H), 6.73–6.75 (m, 3H), 7.16 (t, J = 7.0 Hz, 1H), 7.22–7.28 (m, 6H), 8.04 (s, 1H) ppm; 13C NMR (CDCl3) δ = 41.6, 48.0, 104.4, 105.7, 108.8, 109.7, 113.0, 116.0, 117.9, 118.1, 118.4, 119.2, 129.7, 135.0 ppm; UV (EtOH) λmax = 291 (logε = 4.33) nm; IR (ATR) νmax = 3390s, 3348s, 1617s, 1520vs, 820s, 707s cm–1; MS (70 eV): m/z (rel int) 276 (M+, 100), 199 (48), 185 (34), 155 (10), 138 (16), 137 (17), 84 (11). Anal. Calcd for C19H20N2·0.1H2O: C, 82.04; H, 7.32; N, 10.07%. Found C, 82.07; H, 7.33; N, 9.93%.
3-Benzyl-2-t-butylpyrrole (4h); A brown oil. 1H NMR (CDCl3) δ = 1.34 (s, 9H), 3.99 (s, 2H), 5.88 (t, J = 3.0 Hz, 1H), 6.56 (t, J = 3.0 Hz, 1H), 7.13-7.19 (m, 3H), 7.23-7.27 (m, 2H), 7.91 (s, 1H) ppm; 13C NMR (CDCl3) δ = 30.6, 32.4, 33.6, 112.0, 113.9, 116.3, 125.6, 128.2, 128.7, 135.3, 142.9 ppm; IR (ATR) νmax = 3455s, 3423s, 2959vs, 2906s, 1494s, 1453s, 726vs, 709vs cm–1; UV (EtOH) λmax = 262 (logε = 2.97), 270 (2.86) nm; MS (70 eV): m/z (rel int) 213 (M+, 89), 248 (22), 198 (100), 188 (18), 173 (11), 131 (12), 120 (43), 115 (14), 91 (62). HRMS m/z Calcd for C15H19N (M+) 213.1518, found : 213.1511.
References
1. R. J. Sundberg, In Comprehensive Heterocyclic Chemistry, Vol. 2; ed. by A. R. Katritzky, C. W. Rees, and E. F. V. Scriven; Pergamon, Oxford, 1996, pp.119–206. CrossRef
2. L. Knorr, Ber. Dtsch. Chem. Ges., 1884, 17, 1635. CrossRef
3. C. Paal, Ber. Dtsch. Chem. Ges., 1885, 18, 367; CrossRef R. U. Braun and T. J. J. Mueller, Synthesis, 2004, 2391. CrossRef
4. A. Hantzsch, Ber. Dtsch. Chem. Ges., 1890, 23, 1474. CrossRef
5. For recent examples, see the following; a) O. A. Attansai, G. Favi, F. Mantellini, G. Moscatelli, and S. Santeusanio, J. Org. Chem., 2011, 76, 2860; CrossRef b) H.-Y. Wang, D. S. Mueller, R. M. Sachwani, R. Kapadia, H. N. Londino, and L. L. Anderson, J. Org. Chem., 2011, 76, 3203; CrossRef c) G. R. Reddy, T. R. Reddy, S. C. Joseph, K. S. Reddy, L. S. Reddy, P. M. Kumar, G. R. Krishna, C. M. Reddy, D. Rambabu, R. Kapavarapu, C. Lakshmi, T. Meda, K. K. Priya, K. V. L. Parsa, and M. Pal, Chem. Commun., 2011, 47, 7779; CrossRef d) S. Chiba, Synlett, 2012, 21. CrossRef
6. a) V. P. Reddy, A. V. Kumar, and K. R. Rao, Tetrahedron Lett., 2011, 52, 777; CrossRef b) N. K. Pahadi, M. Paley, R. Jana, S. R. Waetzig, and J. A. Tunge, J. Am. Chem. Soc., 2009, 131, 16626; CrossRef c) A. G. Cook, K. A. Switek, K. A. Cutler, and A. M. Witt, Lett. Org. Chem., 2004, 1, 1; CrossRef d) G. Wittig and A. Hesse, Liebigs Ann., 1971, 746, 174. CrossRef
7. M. Oda, Y. Fukuchi, S. Ito, N. C. Thanh, and S. Kuroda, Tetrahedron Lett., 2007, 48, 9159. CrossRef
8. For the conventional aldol-type condensation of imines, see; P. M. Patrick, Jr., J. Am. Chem. Soc., 1952, 74, 2984. CrossRef
9. a) W. Koller and P. Schlack, Chem. Ber., 1963, 96, 93; CrossRef b) T. Severin and H. Poehlmann, Chem. Ber., 1977, 110, 491; CrossRef c) D. F. Fry, C. B. Fowler, and R. K. Dieter, Synlett, 1994, 836; CrossRef d) C. A. Zezza, M. B. Smith, B. A. Ross, A. Arhin, and P. L. E. Cronin, J. Org. Chem., 1984, 49, 4397; CrossRef e) P. Molian, J. Alcántara, and C. López-Leonardo, Synlett, 1995, 363; CrossRef f) K. L. Sorgi, C. A. Maryanoff, D. F. McComsey, and B. E. Maryanoff, Org. Synth., 1998, 75, 215; g) C. Coindet, A. Comel, and G. Kirsch, Tetrahedron Lett., 2001, 42, 6101; CrossRef h) M. Shi, Y.-H. Yang, and B. Xu, Synlett, 2004, 1622. CrossRef
10. Dannhardt et al. obtained 7a by reaction of 5 with 1,4-diphenyl-2,3-diazabutadiene in 26 % yield. The extension of the method was not reported; a) G. Dannhardt and R. Obergrusberger, Arch. Pharm. (Weinheim), 1981, 314, 811; CrossRef For previous other synthesis of 7a, see; b) S. Sugawara and S. Uchida, Tetrahedron, 1959, 5, 48. CrossRef
11. The configuration of the phenyl group of the benzylidene moiety in 7a was not previously discussed. We tentatively assigned the (E)-configuration as depicted in Scheme 2 because of inherent instabilily of the (Z)-isomer by two phenyl groups closely located to each other. PM3 calculations indicate that the (E)-isomer is more stable than the (Z)-isomer by 3.82 kcal/mol in heat of formation.
12. Under conditions with either a Lewis acid or triethylamine, no condensation product was observed. The alkylation of 7a at the 3 position with alkyl halide under conditions using a strong base, such as LDA, was reported; G. Dannhardt and R. Obergrusgerger, Arch. Pharm. (Weinheim), 1979, 312, 498. CrossRef
13. A BF3-assisted aldol reaction of enamines with acetals was previously reported by Takazawa et al.; O. Takazawa, K. Kogami, and K. Hayashi, Bull. Chem. Soc. Jpn., 1984, 57, 1876. CrossRef