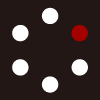
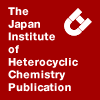
HETEROCYCLES
An International Journal for Reviews and Communications in Heterocyclic ChemistryWeb Edition ISSN: 1881-0942
Published online by The Japan Institute of Heterocyclic Chemistry
e-Journal
Full Text HTML
Received, 25th March, 2012, Accepted, 26th June, 2012, Published online, 29th June, 2012.
DOI: 10.3987/COM-12-12474
■ Synthesis and Structure and Optical Properties of a Zinc(II) Tetrakis(phenylbutadiynyl)porphyrin
Liu-tao Yang, Di Wu,* Hai-feng Xiang, Xiangge Zhou, and Yue-fei Deng*
College of Chemistry, Sichuan University, 610064 Chengdu, China
Abstract
A porphyrin complex, zinc(II) tetrakis(phenylbutadiynyl)porphyrin, is constructed through meso-substitution approach, which exhibits remarkable bathochromism in absorption and fluorescent spectrum. Both the Q bands in the absorption spectrum and the emission band are red-shifted to the saturated red region. The theoretical analysis showed that the meso-phenylbutadiynyl groups significantly extended the π-conjugation from the porphyrin core to the meso aryl groups.Porphyrins have peculiar optical and electronic properties that allow them to play important roles in a variety of potential applications for solar cell dyes,1 photodynamic therapy,2 sensor,3 and so forth, and have been received great efforts. Among these efforts, the bathochromism of the absorption bands is one of the important goals.
It is generally believed that the addition of π-electron subunits can generate red shifts of the electronic spectra. Expanding porphyrin core,4 fusion and substitution of aryl rings at β-pyrrol position5 are reported to be effective approaches to expand π-conjugation. However, because of the spatial limitation around porphyrin core, with the insertion of π-electron (and thereof bulky) groups, all of the efforts have come to a hard-to-overcome problem of large hindrance and hence large distortion of porphyrin plane, which lead to the extreme difficulties in synthesizing and strong inclination to decompose.3c,6 On the other sides, meso-aryl-substituents cannot work so well on the spectral bathochromism due to the obvious dihedral and therefore π-breakage between meso-phenyl rings and porphyrin core in the case of tetra-phenyl porphyrin (TPP). Introducing π-bridges, such as ethyne,7 polyyne,8 ethylene9 and aromatic entities,10 can solve the problem so well that the π-system can extend to the aryl rings on the opposite side of the π-bridges. What is more, such approach can contribute a lot to solving the hindrance problem faced in all the other approaches mentioned above. However, in contrast to the intensive studies of TPP11 and the achievements around core-expanded, aryl-fused porphyrins, the studies on the meso-ethynyl substituted porphyrin are relatively rare.7b,7c,12 A meso-tetrakis(phenylethynyl)porphyrin (3) has been reported, whose absorption bands are significantly red-shifted compared with that of the Zn(II) tetraphenylporphyrin (ZnTPP, 1).12,13 How would it be if more ethynyl groups are inserted into the meso-π-bridge? Here we describe the synthesis and spectroscopic properties of the Zn(II) 5, 10, 15, 20-tetrakis(phenylbutadiynyl) porphyrin (4). The theoretical studies were performed to provide more insight into the effects of alkynyl on electronic properties.
The key step in the synthetic approach to the target compound 4 is the reaction of meso-tetraethynylporphyrin (2) and phenylbromoethyne through Sonogashira coupling (Scheme 1) with moderate yield.12 The solution in THF and solid of 4 is green and blue, respectively. Phenylbromoethyne was prepared according to the literature method.14
The chemical structure of 4 was identified by 1H and 13C NMR spectrum. 1H NMR spectrum showed the expected eight proton resonances at 8.97 ppm as a singlet for the β-pyrrole-Hs, and 20 proton resonances divided into three parts around 7.42-7.80 ppm for the phenyl-Hs. 13C NMR spectrum gave 11 peaks in agreement with the expectation of proposed structure for 4.
Spectroscopic properties
The red-shifted absorption and emission spectra of porphyrin 4 indicate the expansion of π-conjugation system. As shown in Figure 1 and Table 1, the electron spectrum exhibits characteristic porphyrin absorptions: a sharp Soret band at 491 nm and two Q bands in the lower energy region. Unlike the usually weak Q00 bands for the porphyrins, the intensity of Q00 band for 4 is exceptionally high with a large absorption coeffient of 1.95×104 L·mol-1·cm-1, due to the large splitting of the HOMO and HOMO-1 band.15 It is worth mentioning that the Q00 bands(corresponding to the S0→S1 transition) and the S1→S0 emission band were both remarkably red-shifted into the saturated red region (690 and 697 nm), which make 4 emit bright red fluorescence with a quantum yield of 1.8% under UV lamp. The bands of 4 are significantly red-shifted compared to that of the reference porphyrins as seen in Table 1. By symmetrical inserting four C≡C groups between phenyl groups and porphyrin core with respect to porphyrin 3, the Soret band was further red-shifted by 14 nm, and the Q-band by 11 nm. These can be evidences that a larger π-system is formed.
Note that this large π-conjugated porphyrin is constructed in the approach of meso-substitution. π-conjugated porphyrins have been reported through all kinds of ways from fusion of aryl rings to core expansion. A core-expanded dodecaphyrin (604 nm for Soret band)16 and a core-expanded and phenanthrene-fused six-membered ring, hexaphyrin (597 nm for Soret band)3c can serve as good examples. However, the steric hindrances around porphyrins ineglectably hamper the construction of large porphyrin rings. In this reason, the molecular structures have to distort remarkably out of the plane in order to make space for the newly inserted groups at the cost of both the bathochromism of electronic spectra and stability, which leads to the difficulties coming across the synthesis, purifications, measurements, reservations and further applications. The inserting C≡C groups can push the meso-phenyl groups far away from the close nearby positions around porphyrin core where fusion aryl groups are generally crowded, so that benefit in the planarity of porphyrin in larger π-system of porphyrins, and consequently result in further red shift of the spectra.
It is interesting to further ascertain the roles of C≡C groups. They act as 1) π-bridges so that the π-system can be expanded from porphyrin core to the other end of the π-bridges, and meanwhile 2) donors of two π-electrons for each ethynyl group, which contribute in a certain amount to π-expansion. In most cases, the spectral variation from 1 to 3 as instance, the B-band gives a red shift of 55 nm, which can be attributed to the composition of the two factors mentioned above. However, in the case of those from 3 to 4, the B-band has a bathochromism of only 14 nm. We can infer that the main contribution of C≡C groups is not electron donation but the π-system communication in that four C≡C groups can donate the same amount of π-electrons in the variation from 1 to 3 and from 3 to 4, while they differ mainly in the aspect that the meso-phenyl groups change obviously in their position from 1 to 3, but subliminally from 3 to 4. The crystal structure may help manifest the inference, but we are not so lucky to get the crystal probably due to its poor stability. The theoretical optimization can be an alternate.
Computational Details
To gain more insight into the effect of alkynyl groups on molecular structures and electronic properties of these porphyrins, quantum chemical studies were carried out applying density functional theory (DFT) at B3LYP/6-31G* level for porphyrin 4 and the reference porphyrins. All theoretical calculations were performed without considering the influence of solvation.
The optimized molecular structures are displayed in Figure 2. Alkynyl bridges can substantially affect the molecular structures. Firstly, without alkynyl groups, the 24 atoms in the porphyrin core deviate from a plane by 0.052 Å (Table 2), while with more or less C≡C triple bonds inserted, porphyrin cores can stay exactly in the same planes(0.00 Å for RMS deviation). Secondly, the average dihedrals between the meso-aryl substituents and the plane of porphyrin core obviously decrease with the insertion of alkynyl groups. In contrast to the nearly perpendicular (70.15o as shown in Table 2) position of phenyl rings to the plane of porphyrin core in the case of 1, porphyrin 3 and 4 have the dihedral of only 0.40 o and 0.18 o respectively. The more alkynyl groups, the smaller the angles are, which indicates that the C≡C triple bonds can contribute a lot to the planarity of the whole molecules. This can be explained in a way that with the interval of C≡C bridges, repulsive force along with the steric hindrance between meso-phenly groups can be reduced and the phenyl rings can easily turn back into the plane of porphyrins for the purpose of sharing π-obitals and lowering the energy.
Consequently, the electronic distribution has been greatly affected by the addition of C≡C triple bonds. Through these effective π-bridges, phenyl rings can share electrons on their π-obitals with the large π-system of porphyrin and electrons can communicate easily. The increasing electronic probability densities can be found on the phenyl rings in porphyrin in the sequence of 1, 2, 3 and 4, as can be seen in Figure 3, which can serve as good evidence. Thus we can conclude that a larger π-system is formed through the approach of meso-substitution.
In conclusion, we have successfully synthesized a enlarged π-conjugated porphyrin 4 through meso-substitution approach. The new compound exhibits large red shifts of Soret and Q-bands compared with that of porphyrins 1 and 3. There is an intensified and red-shifted Q00 band for 4 centered at saturated red region of 690 nm. The fluorescent band was also red-shifted to 697 nm. The optical spectra along with theoretical analysis showed that the meso-phenylbutadiynyl groups significantly extend the π-conjugation of the porphyrin core. Besides the π-electron donation, C≡C triple bonds can overcome the steric hindrance usually happened in meso-tetraayrlporphyrins, so that the π-system can extend far away to the meso aryl groups. This may result in a possibility to arrange more groups around porphyrin core without overcrowding and therefore avoid the distortion of the porphyrin plane, which may benefit to the further bathochromism of the optical spectra. The energy level of porphyrin 4 was stabilized, and simultaneously the energy gap was narrowed. These properties may have the potential applications in the saturated red dye needed fields such as dye-sensitized solar cells, fluorescent bioimaging and photodynamic therapy.17
EXPERIMENTAL
1H NMR spectra and 13C NMR were recorded with Bruker Avance-400 spectrometers, respectively. The UV-vis absorption and fluorescent spectrum are detected by UV-765 UV-vis spectrophotometer (Shanghai Precision Scientific Instrument Co., Ltd) and Hitachi F-4500 fluorescent emission spectrometer, respectively. MALDI-TOF spectra were obtained on Brucker Daltonics Alphaflex II instrument using anthracene or cinnamic acid matrices. Melting point was measured with a Yanaco M500-D melting point apparatus.
Meso-tetraethynylporphyrin (2) was obtained according to published method.7c Other reagents and solvents were received from the respective commercial suppliers; solvents were purified according to standard procedures.
Zinc tetrakis(phenylbutadiynyl)porphyrin (4). To a degassed dry THF solution (100 mL) of phenylbromoethyne (380 mg, 2.1 mmol), porphyrin 2 (100 mg, 0.21 mmol), (Pd (PPh3)2Cl2) (13 mg, 16 μmol), CuI (3 mg, 16 μmol) and Et3N (48 mL) were added. The reaction mixture was stirred at room temperature for 18 h. The solvent was evaporated to dryness under vacuum. The product was purified by recrystallizing from a mixture of THF and MeOH to give a pure solid (63 mg, 34%). Mp 288−292 °C (decomp.). UV-vis (THF): λmax (ε×105) = 491 (1.197), 636 (0.055), 690 (0.195) nm; 1H NMR (400 MHz, DMSO-d6), δH = 8.97 (s, 8H, pyrrole-H), 7.80 (d, 8H, J = 7.2 Hz, Ar-H), 7.51−7.55 (t, 4H, Ar-H), 7.42−7.45 (t, 8H, Ar-H); 13C NMR (100 MHz, THF-d8), δC = 149.66, 130.72, 128.94, 128.67, 127.35, 126.73, 126.66, 120.32, 99.12, 81.96, 73.13. MALDI-TOF mass (C60H28N4Zn): 869.28 [MH]+.
ACKNOWLEDGEMENTS
This project is sponsored by the Natural Science Foundation of China (Nos. 20901052, J1103315/J0104, 30971070) and the Sichuan Provincial Foundation (08ZQ026-041). We also thank Prof. Dan Xiao in Sichuan University for fluorescence test.
References
1. (a) A. Yella, H.-W. Lee, H. N. Tsao, C.-Y. Yi, A. K. Chandiran, M. K. Nazeeruddin, E. W.-G. Diau, C.-Y. Yeh, S. M. Zakeeruddin, and M. Grätzel, Science, 2011, 334, 629; CrossRef (b) Y.-C. Chang, C.-L. Wang, T.-Y. Pan, S.-H. Hong, C.-M. Lan, H.-H. Kuo, C.-F. Lo, H.-Y. Hsu, C.-Y. Lin, and E. W.-G. Diau, Chem. Commun., 2011, 47, 8910; CrossRef (c) C.-J. Jiao, N.-N. Zu, K.-W. Huang, P. Wang, and J.-S. Wu, Org. Lett., 2011, 13, 3652; (d) T. Bessho, S. M. Zakeeruddin, C.-Y. Yeh, and E. W.-G. Diau, Angew. Chem. Int. Ed., 2010, 49, 6646; CrossRef (e) A. J. Mozer, M. J. Griffith, G. Tsekouras, and P. Wagner, J. Am. Chem. Soc., 2009, 131, 15621; CrossRef (f) M. Wahadoszamen, T. Yamamura, A. Momotake, Y. Nishimura, and T. Arai, Heterocycles, 2009, 79, 331. CrossRef
2. (a) X.-C. Zhu, W.-T. Lu, Y.-Z. Zhang, A. Reed, B. Newton, Z. Fan, H.-T. Yu, P. C. Ray, and R.-M. Gao, Chem. Commun., 2011, 47, 10311; CrossRef (b) M. O. Senge, M. Fazekas, M. Pintea, M. Zawadzka, and W. J. Blau, Eur. J. Org. Chem., 2011, 5797; CrossRef (c) D. G. Hilmey, M. Abe, M. I. Nelen, and C. E. Stilts, J. Med. Chem., 2002, 45, 449. CrossRef
3. (a) H. Kotani, K. Ohkubo, M. J. Crossley, and S. Fukuzumi, J. Am. Chem. Soc., 2011, 133, 11092; CrossRef (b) M. Ishida, Y. Naruta, and F. Tani, Angew. Chem. Int. Ed., 2010, 49, 91; CrossRef (c) D. Wu, A. B. Descalzo, F. Weik, F. Emmerling, Z. Shen, X.-Z. You, and K. Rurack, Angew. Chem. Int. Ed., 2008, 47, 193. CrossRef
4. (a) S. Saito and A. Osuka, Angew. Chem. Int. Ed., 2011, 50, 4342; CrossRef (b) A. Osuka and S. Saito, Chem. Commun., 2011, 47, 4330; CrossRef (c) A. Hassner and E. Fogler, Heterocycles, 2009, 77, 301. CrossRef
5. (a) T. Okujima, G.-N. Jin, N. Matsumoto, J. Mack, S. Mori, K. Ohara, D. Kuzuhara, C. Ando, N. Ono, H. Yamada, H. Uno, and N. Kobayashi, Angew. Chem., 2011, 123, 5817; CrossRef (b) J. Sankar, S. Mori, S. Saito, H. Rath, M. Suzuki, Y. Inokuma, H. Shinokubo, K. S. Kim, Z. S. Yoon, J.-Y. Shin, J. M. Lim, Y. Matsuzaki, O. Matsushita, A. Muranaka, N. Kobayashi, D. Kim, and A. Osuka, J. Am. Chem. Soc., 2008, 130, 13568; CrossRef (c) C.-X. Cai, H. Uoyama, M. Nakamura, and H. Uno, Heterocycles, 2012, 84, 829. CrossRef
6. H.-J. Xu, J. Mack, A. B. Descalzo, Z. Shen, N. Kobayashi, X.-Z. You, and K. Rurack, Chem. Eur. J., 2011, 17, 8965. CrossRef
7. (a) T. Ishizuka, L. E. Sinks, K. Song, S.-T. Hung, A. Nayak, K. Clays, and M. J. Therien, J. Am. Chem. Soc., 2011, 133, 2884; CrossRef (b) T.-H. Huang, Y.-J. Chen, S.-S. Lo, W.-N. Yen, C.-L. Mai, M.-C. Kuo, and C.-Y. Yeh, Dalton Trans., 2006, 2207; CrossRef (c) W.-N. Yen, S.-S. Lo, M.-C. Kuo, C.-L. Mai, G.-H. Lee, S.-M. Peng, and C.-Y. Yeh, Org. Lett., 2006, 8, 4239; CrossRef (d) Z. Shen, H. Uno, Y. Shimizu, and N. Ono, Org. Biomol. Chem., 2004, 2, 3442; CrossRef (e) A. Krivokapic, A. R. Cowley, and H. L. Anderson, J. Org. Chem., 2003, 68, 1089. CrossRef
8. (a) H. Nobukuni, Y. Shimazaki, F. Tani, and Y. Naruta, Angew. Chem., 2007, 119, 9133; CrossRef (b) Y.-J. Chen, S.-S. Chen, S.-S. Lo, T.-H. Huang, C.-C. Wu, G.-H. Lee, S.-M. Peng, and C.-Y. Yeh, Chem. Commun., 2006, 1015; CrossRef (c) L.-Y. Zhu, Y.-P. Yi, Z.-G. Shuai, K. Schmidt, and E. Zojer, J. Phys. Chem. A, 2007, 111, 8509. CrossRef
9. (a) M. J. Frampton, H. Akdas, A. R. Cowley, J. E. Rogers, J. E. Slagle, P. A. Fleitz, M. Drobizhev, A. Rebane, and H. L. Anderson, Org. Lett., 2005, 7, 5365; CrossRef (b) M. Chachisvilis, V. S. Chirvony, A. M. Shulga, B. Källebring, S. Larsson, and V. Sundström, J. Phys. Chem., 1996, 100, 13857. CrossRef
10. (a) P. Thamyongkit and J. S. Lindsey, J. Org. Chem., 2004, 69, 5796; CrossRef (b) S. Cho, M.-C. Yoon, C. H. Kim, N. Aratani, G. Mori, T. Joo, A. Osuka, and D. Kim, J. Phys. Chem. C, 2007, 111, 14881; CrossRef (c) F. Hajjaj, Z. S. Yoon, M.-C. Yoon, J. Park, A. Satake, D. Kim, and Y. Kobuke, J. Am. Chem. Soc., 2006, 128, 4612; CrossRef (d) M. Taniguchi and J. S. Lindsey, Tetrahedron, 2010, 66, 5549. CrossRef
11. (a) S. K. Sugunan, B. Robotham, R. P. Sloan, J. Szmytkowski, K. P. Ghiggino, M. F. Paige, and R. P. Steer, J. Phys. Chem. A, 2011, 115, 12217; CrossRef (b) X.-F. Liu and X.-W. Xiao, J. Organomet. Chem., 2011, 696, 2767; CrossRef (c) T. Takanami, S. Nakajima, S. Nakadai, F. Hino, and K. Suda, Heterocycles, 2009, 77, 365; CrossRef (d) L.-L. Li, P. Y. Cai, Y.-F. Deng, L.-T. Yang, X. He, L.-S. Pu, D. Wu, J. Liu, H.-F. Xiang, and X.-G. Zhou, J. Porphyrins Phthalocyanines, 2011, 15, 1; CrossRef (e) B. Temelli and S. S. Ozdemir, Heterocycles, 2012, 85, 851. CrossRef
12. M.-C. Kuo, L.-A. Li, W.-N. Yen, S.-S. Lo, C.-W. Lee, and C.-Y. Yeh, Dalton Trans., 2007, 1433. CrossRef
13. . CrossRef
14. X.-P. Nie and G.-J. Wang, J. Org. Chem., 2006, 71, 4734. CrossRef
15. Y. Takeuchi, A. Matsuda, and N. Kobayashi, J. Am. Chem. Soc., 2007, 129, 8271. CrossRef
16. R. Kumar, R. Misra, and T. K. Chandrashekar, Org. Lett., 2006, 8, 4847. CrossRef
17. (a) P.-C. Lo, X.-B. Leng, and D. K. P. Ng, Coordin. Chem. Rev., 2007, 251, 2334; CrossRef (b) H. Imahori, T. Umeyama, and S. Ito, Acc. Chem. Res., 2009, 42, 1809; CrossRef (c) E. F. F. Silva, C. Serpa, J. M. Dabrowski, and C. J. P. Monteiro, Chem. Eur. J., 2010, 16, 9273; CrossRef (d) C. E. Stilts, M. I. Nelen, D. G. Hilmey, S. R. Davies, S. O. Gollnick, A. R. Oseroff, S. L. Gibson, R. Hilf, and M. R. Detty, J. Med. Chem., 2000, 43, 2403. CrossRef