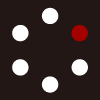
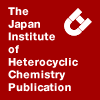
HETEROCYCLES
An International Journal for Reviews and Communications in Heterocyclic ChemistryWeb Edition ISSN: 1881-0942
Published online by The Japan Institute of Heterocyclic Chemistry
e-Journal
Full Text HTML
Received, 29th March, 2012, Accepted, 27th April, 2012, Published online, 2nd May, 2012.
DOI: 10.3987/COM-12-S(N)8
■ Synthesis of Antineoplastic Analogs of Aplysiatoxin with Various Side Chain Structures
Yuki Shu, Ryo C. Yanagita, Harukuni Tokuda, Nobutaka Suzuki, and Kazuhiro Irie*
Division of Food Science and Biotechnology, Graduate School of Agriculture, Kyoto University, Kitashirakawa Oiwake-cho, Sakyo-ku, Kyoto 606-8502, Japan
Abstract
We have recently developed a simplified analog of aplysiatoxin with anti-proliferative activity (1). To investigate the structure–activity relationship of its side chain, an alternative synthetic route of 1 has been established. Via the key intermediate 6, p-hydroxyl or o,m-dihydroxyl derivatives (4 and 5) as well as 1 were synthesized and their biological activities were evaluated. Although the position of the hydroxyl group in the benzene ring did not change the affinity for protein kinase C isozymes or the ability to induce the Epstein-Barr virus early antigen, anti-proliferative activities against several human cancer cell lines of 1 were superior to those of 4.INTRODUCTION
Protein kinase C (PKC) is a family of serine/threonine kinases that play a pivotal role in cell proliferation, differentiation, and apoptosis.1 Eleven PKC isozymes are classified into three groups: conventional (α, βI, βII, γ), novel (δ, ε, η, θ), and atypical (ζ, λ/ι).2 Naturally-occurring tumor promoters such as 12-O-tetradecanoylphorbol 13-acetate (TPA), teleocidin B-4, and aplysiatoxin (ATX) bind to tandem C1 domains (C1A, C1B) in the regulatory regions of conventional and novel PKC isozymes, inducing their translocation to the cellular membrane, driven by an affinity for anionic phospholipids, resulting in the abnormal activation of PKC.3
Bryostatin 1 (bryo-1)4 is also a potent activator of PKC isolated from the marine bryozoan Bugula neritina. Although bryo-1, like tumor promoters, binds to the C1 domains of PKC isozymes and activates them, it exhibits little tumor-promoting activity.5 Bryo-1 has anticancer activity and is currently undergoing clinical trials for the treatment of solid tumors, leukemia, and lymphoma.6 Bryo-1 is also considered to have therapeutic potential for Alzheimer's disease7 (AD) and AIDS8 where PKC isozymes are involved. However, the yield of bryo-1 isolated from natural sources is quite low{Mutter, 2000, Chemistry and clinical biology of the bryostatins}{Mutter, 2000, Chemistry and clinical biology of the bryostatins}.9 Recently, efficient means of producing bryo-related compounds have been reported,10 but it remains difficult to obtain a sufficient amount of bryos for clinical use.
While other natural and synthetic PKC activators such as TPA and teleocidin analogs also have potential for the treatment of cancer11 and AD,12 serious adverse effects such as gross hematuria, a grand mal seizure, syncope, and hypotension were encountered during a phase I clinical trial.11 Pleiotropic effects including tumor-promoting activity of these compounds would thus be of particular concern. Since hydrophobicity of PKC activators generally correlates with their tumor-promoting ability,13 prostratin (12-deoxyphorbol 13-acetate), a less hydrophobic phorbol ester without tumor-promoting activity, is promising in the anti-HIV therapy.14 However, isolation yield of prostratin from natural sources was poor like bryo-1, and its binding potency for PKC isozymes was very weak.
To solve these problems, we have developed simplified analogs of ATX (1 and 2)15 with less hydrophobicity. Compound 1, synthesized in only 22 steps, behaved like bryo-1 in the translocation of PKCδ, a PKC isozyme involved in anti-tumor promotion and anti-proliferative effects in several cancer cell lines,16,17 and in the induction of the Epstein-Barr virus early antigen (EBV-EA). The anti-proliferative activity of 1 against many human cancer cell lines was comparable to that of bryo-1.15 In the EBV-EA induction test, 1 showed weak EA induction and suppressed the induction by the tumor promoter TPA, suggesting 1 as well as bryo-1 to be an anti-tumor promoter rather than tumor promoter.15
We have recently synthesized a dehydroxyl derivative of 1 (3), similar in affinity for PKCδ to 1.18 While the anti-proliferative activities of 3 against 39 human cancer cell lines were almost equivalent to those of 1, effects on some cell lines, e.g., LOX-IMVI melanoma and St-4 stomach cancer cell lines, were different from those of 1.18 The anti-tumor-promoting activity of 3 estimated by the EBV-EA induction test was weaker than that of 1,18 indicating the phenolic hydroxyl group of 1 to be important for the activity. Therefore, it is necessary to investigate the influence of this group on various biological activities for the development of analogs with less adverse effects. Although the synthesis of 1 was established,15 it is not easy to modify its side chain. Notably, derivatives with an o- or p-hydroxyl group on the benzene ring cannot be synthesized by the previous route15 because of the oxidation at the benzyl position in the last stages of the synthesis. In this paper, we describe an alternative route for producing 1 via a key intermediate (6) with a primary hydroxyl group in the side chain. New analogs of 1 with p-hydroxyl (4) or o,m-dihydroxyl groups (5) were synthesized using the key intermediate, and their biological activities were evaluated.
RESULTS AND DISCUSSION
To elucidate the effects of a phenolic hydroxyl group in the benzene ring on biological activities, we planned the synthesis of 4 and 5 as shown in Scheme 1. The production of 4 and 5 could be quite difficult through the previous route15 where oxidative cleavage of the double bond with KMnO4 was employed before final cyclization of the diolide ring. A benzyloxy group at the o- or p-position of the alkyl benzene would activate the aromatic ring to induce a side reaction (e.g., oxidation at the benzyl position). Based on this, we planned an alternative route via the key intermediate 6. This intermediate has a common structure of each derivative (4 and 5), and each side chain can be inserted in the final stages of the synthesis. This route also enables us to synthesize derivatives of 1 with various substituents more easily.
Synthesis of the key intermediate (6) was carried out by referring to our previous synthetic scheme for 115 with appropriate modifications (Scheme 2). Synthesis of 6 began with the preparation of known (4R)-1-(tert-butyldiphenylsilyloxy)hept-6-en-4-ol19 (10, 92% ee) from 1,4-butanediol in three steps including protection of one hydroxyl group with tert-butyldiphenylsilyl (TBDPS) ether, Swern oxidation, and asymmetric Maruoka's allylation.20 Protection of the alcohol (10) with tert-butyl carbonate provided 11. Stereoselective iodocarbonate cyclization by Duan and Smith21 followed by treatment with K2CO3 in MeOH gave an epoxide (12). The hydroxyl group of 12 was protected with triethylsilyl (TES) ether to yield the epoxide unit (7).
The epoxide (7) was coupled with 8, which was prepared from [(4-(1,3-dithian-2-yl)-4-methylpentyl)oxy]triisopropylsilane,15 to yield 13. Selective deprotection of 2-tetrahydropyranyl (THP) and TES groups of 13, followed by protection of a resulting diol with 2,2-dimethoxypropane afforded 14. After several trials, it was found that THP is most appropriate to protect the hydroxyl group of 8. Compound 14 was converted into an aldehyde (15) by Ley oxidation22 followed by Maruoka's asymmetric allylation20 to give 16 (93% de). Deprotection of the isopropylidene group of 16 afforded a diol (17). Subsequent cleavage of the dithiane gave a mixture of spiroketals (18 and 19) at a ratio of 1:1.3. Their configurations were determined by NOESY experiments; NOE cross-peaks were observed between H-12 and C10-OH, and between H-4. and H-9eq. in 18, and between H-4 and H-13 in 19. The undesired isomer (19) was converted into the desired one (18) by chelation with zinc chloride.23
The carboxylic acid unit (9) was synthesized from (R)-1-(benzyloxy)-3-(1,3-dithian-2-yl)propan-2-ol24 prepared from (R)-(−)-glycidyl ether, by protection of a hydroxyl group with TES ether, cleavage of dithiane, and Pinnic oxidation.25 Compound 18 was condensed with 9 by the method of Yamaguchi and co-workers.26 The resultant 20 was converted to a carboxylic acid (21) by oxidative cleavage of the olefin followed by deprotection of TES and TBDPS. Further Yamaguchi's macrolactonization26 afforded the key intermediate 6 in 18 steps from 1,4-butanediol with an overall yield of 0.6%. Although 21 had two hydroxyl groups, only the desired one reacted with the carboxyl group to close the ring because of the long distance between another hydroxyl group and the carboxyl group.
The synthesis of 4 and 5 was completed via the key intermediate 6, which was converted to an aldehyde (22). The side chain units (23 and 24) were prepared by conventional methods, bromination and heating with triphenylphosphine. Wittig reactions of 22 with each side chain unit provided 25 and 26 as 2:1 and 4:5 E/Z mixtures, respectively. Finally, catalytic hydrogenation of 25 and 26 provided 4 and 5, respectively. Compound 1 was also obtained similarly from 22 (see experimental section).
Compounds 4 and 5 were initially evaluated for their affinity for the synthetic C1 domains of PKCα and δ by inhibition of the specific binding of [3H]phorbol 12,13-dibutylate (PDBu) as reported previously.27,28 α-C1A, the major PDBu-binding site of PKCα,28 was selected as representative of conventional PKC isozymes. Both the C1A and C1B domains of PKCδ were employed since binding to these domains could be important for bryo-1-like activities.29 The affinity of 4 for these peptides was almost equal to that of 1 (Table 1). This suggests that the position of the phenolic hydroxyl group at the side chain might not affect the binding to PKCα and δ-C1 peptides. The side chain of 1 would interact with membrane phospholipids nonspecifically in the PKC–ligand–phospholipid ternary complex, as that of ATX.30 In contrast, the affinity of 5 with two phenolic hydroxyl groups for PKCα and δ-C1 peptides decreased, possibly because of decreased hydrophobicity. The affinity of 5 was stronger than that of 2 lacking the dimethyl group at the spiroketal moiety, though 5 is slightly more hydrophilic than 2 (Clog P of 2: 1.9, that of 5: 1.6). Although the side chain of 5 appears to interact non-specifically with membrane phospholipids, the affinity of 5 for PKC isozymes might remain to some extent.
Anti-proliferative activity of 4 against a panel of 39 human cancer cell lines was evaluated as described previously.32 Since compounds with weak affinity for PKCδ were deduced to show low anti-proliferative activity as observed in 2,15 only 4 was subjected to evaluation. The growth inhibitory activity was expressed as the GI50 (M), the concentration required to inhibit cell growth by 50% compared to an untreated control. The average of the log GI50 values of each 39 human cancer cell line was expressed as MG-MID.
Compound 4 showed anti-proliferative activity comparable to 1 and 3; the MG-MID of 4 was –4.97, almost equal to those of 1 and 3 (–4.98 and −5.09, respectively). The cell lines with log GI50 values less than –5.10 in 1 are listed in Table 2. Slightly stronger activity of 3 without phenolic hydroxyl group might be ascribable to its increasing membrane permeating ability.18 While PKC binding ability of 1 and 4 were almost equal to each other, 1 showed stronger anti-proliferative activity against several of the cell lines in Table 2, especially MDA-MB-231, LOX-IMVI, and St-4 cells, than 4. Compound 1 also showed stronger activity against LOX-IMVI than 3. These results suggest that there might be targets other than PKC isozymes that the phenolic hydroxyl group at meta-position interacts with.
The most likely adverse effects of 1 and its derivatives would be tumor-promotion because these compounds possess the skeleton of tumor-promoting ATX. Hence, possible tumor-promoting activity was estimated by the Epstein–Barr virus early antigen (EBV-EA) induction test as described previously.33 EBVs, strictly controlled by host human lymphoblastoid Raji cells, are activated by tumor promoters to produce early antigen.34 As shown in Figure 2a, TPA, a potent tumor promoter, strongly induced EBV-EA production at 100 nM. On the other hand, 4 and 5 as well as 1 showed weak EA induction even at 1000 nM (Figure 2a), with 5 slightly weaker than 1 and 4. Compound 1 exhibited not only weak EA-induction but also an inhibitory effect on the EA-production induced by TPA.15 Similarly, 100 nM of 4 and 5 both significantly suppressed the EA induction by TPA (32 nM) (Figure 2b). These results suggest that anti-tumor-promoting activity might not correlate with the affinity for PKC isozymes and that the existence of a phenolic hydroxyl group would be important for inhibitory activity against tumor promoters as reported previously.18
In summary, we developed an alternative synthetic route for 1 to modify its side chain. Using this route via the key intermediate (6), new derivatives with hydroxyl groups at the p- or o,m-positions of the benzene ring (4 and 5) as well as 1 were synthesized. Many derivatives of 1 with various side chain structures would be available by this route. The affinity for PKCα and δ-C1 peptides and anti-tumor-promoting activity of 4 were almost the same as those of 1. However, the anti-proliferative activity of 4 against several cancer cell lines was slightly different from that of 1. Further study on the structure–activity relationship of the side chain of 1 is necessary for its structural optimization as a therapeutic lead.
EXPERIMENTAL
General remarks. The following spectroscopic and analytical instruments were used: Digital Polarimeter, Jasco P-2200; 1H, 13C, and 2-D NMR, AVANCE III 400 and AVANCE III 500 (Bruker, Germany, ref. TMS, 296 K); HPLC, Waters Model 600E with a Model 2487 UV detector; HR-FAB-MS and HR-EI-MS, JMS-600H and JMS-700 (JEOL, Tokyo, Japan); HPLC was carried out on an SL12S05-2510WT (silica gel, 10 mm i.d. × 250 mm) column (Yamamura Chemical Laboratory, Japan). Wako gelTM C-200 (silica gel, Wako Pure Chemical Industries, Japan) and YMC A60-350/250 gel (ODS, Yamamura Chemical Laboratory) were used for column chromatography. [3H]PDBu (19.6 Ci/mmol) was purchased from PerkinElmer Japan, Yokohama. All other chemicals and reagents were purchased from chemical companies and used without further purification.
Synthesis of the epoxide unit 7. To a solution of (4R)-1-(tert-butyldiphenylsilyloxy)hept-6-en-4-ol (10) (1.77 g, 4.80 mmol) in THF (25 mL) was added dropwise 1 M NaHMDS in THF (6.0 mL, 6.0 mmol, 1.25 equiv.) at 4 °C. After 30 min of stirring, (Boc)2O (1.40 g, 6.42 mmol, 1.33 equiv.) was added, and the reaction mixture was stirred for 6 h at rt. The reaction was quenched with brine (30 mL) and water (10 mL), and the mixture was extracted with EtOAc. The combined organic layers were washed with brine, dried over Na2SO4, filtered, and concentrated in vacuo. The residue was purified by column chromatography (silica gel, 1% EtOAc/hexane) to afford 11 (1.46 g, 3.12 mmol, 65%) as clear oil. Compound 11: [α]D +6.5° (c 1.28, CHCl3, 20.8 °C); 1H NMR δ (400 MHz, CDCl3, 0.038 M) ppm: 1.04 (9H, s), 1.47 (9H, s), 1.59-1.78 (4H, m), 2.34 (2H, t, J = 7.1 Hz), 3.66 (2H, m), 4.69 (1H, m), 5.05-5.12 (2H, m), 5.76 (1H, m), 7.36-7.40 (6H, m), 7.65 (4H, m); 13C NMR δ (125 MHz, CDCl3, 0.107 M) ppm: 19.21, 26.85 (3C), 27.81 (3C), 28.33, 30.04, 38.71, 63.50, 76.42, 81.67, 117.80, 127.61 (4C), 129.55 (2C), 133.56 (2C), 133.93, 135.56 (4C), 153.37; HR-FAB-MS (matrix: 3-nitrobenzyl alcohol): m/z 469.2761 (MH+, calcd for C28H41O4Si, 469.2774).
To a solution of 11 (6.84 g, 14.6 mmol) in toluene (77 mL) was added IBr (4.50 g, 21.8 mmol, 1.5 equiv.) in CH2Cl2 (30 mL) dropwise at −78 °C under an Ar atmosphere. After 2 h of stirring at the same temperature, the reaction was quenched with a 1:1 mixture of 10% aq. Na2S2O3 and saturated aq. NaHCO3 (200 mL), and the reaction mixture was extracted with EtOAc. The combined organic layers were washed with brine, dried over Na2SO4, filtered, and concentrated in vacuo. The residue was purified by column chromatography (silica gel, 15% EtOAc/hexane) to afford an iodide. To a solution of the iodide in anhydrous MeOH (70 mL) was added K2CO3 (7.46 g, 54.0 mmol, 5 equiv.). After 3 h of stirring at rt, the reaction was quenched with H2O (120 mL), and the reaction mixture was extracted with EtOAc. The combined organic layers were washed with brine, dried over Na2SO4, filtered, and concentrated in vacuo. The residue was purified by column chromatography (silica gel, 20% EtOAc/hexane) to yield 12 (2.88 g, 7.50 mmol, 52% in 2 steps) as clear oil. Compound 12: [α]D +7.6° (c 0.45, CHCl3, 18.2 °C); 1H NMR δ (400 MHz, CDCl3, 0.038 M) ppm: 1.05 (9H, s), 1.58-1.70 (5H, m), 1.80 (1H, dt, J = 14.3, 4.2 Hz), 2.51 (1H, dd, J = 5.0, 2.7 Hz), 2.69 (1H, d, J = 3.5 Hz, OH), 2.78 (1H, dd, J = 5.0, 4.1 Hz), 3.09 (1H, m), 3.70 (2H, t, J = 5.7 Hz), 3.90 (1H, m), 7.36-7.43 (6H, m), 7.67 (4H, m); 13C NMR δ (125 MHz, CDCl3, 0.107 M) ppm: 19.21, 26.85 (3C), 28.17, 30.46, 37.11, 46.40, 48.93, 54.71, 63.33, 127.64 (4C), 129.60 (2C), 133.82 (2C), 135.56 (4C); HR-FAB-MS (matrix: 3-nitrobenzyl alcohol): m/z 385.2199 (MH+, calcd for C23H33O3Si, 385.2199).
To a solution of 12 (2.88 g, 7.50 mmol) in CH2Cl2 (60 mL) was added DMAP (3.70 g, 30.1 mmol, 4 equiv.) and Et3N (5.20 mL, 37.7 mmol, 5 equiv.). To this solution was added TES-Cl (1.90 mL, 11.3 mmol, 1.5 equiv.) dropwise at 4 °C under an Ar atmosphere. After 10 min of stirring at the same temperature, the reaction mixture was stirred at rt for an additional 1.5 h. The reaction was quenched with saturated aq. NaHCO3 (100 mL), and the mixture was extracted with CH2Cl2. The combined organic layers were washed with brine, dried over Na2SO4, filtered, and concentrated in vacuo. The residue was purified by column chromatography (silica gel, 2% EtOAc/hexane) to yield 7 (3.0 g, 6.0 mmol, 81%) as clear oil. Compound 7: [α]D +2.3° (c 0.52, CHCl3, 21.6 °C); 1H NMR δ (400 MHz, CDCl3, 0.024 M) ppm: 0.59 (6H, q, J = 7.9 Hz), 0.95 (9H, t, J = 7.9 Hz), 1.04 (9H, s) 1.58-1.63 (5H, m), 1.75 (1H, dt, J = 14.0, 5.9 Hz) 2.44 (1H, dd, J = 5.1, 2.7 Hz), 2.74 (1H, t, J = 4.5 Hz), 3.00 (1H, m), 3.67 (2H, m), 3.88 (1H, t, J = 5.4 Hz), 7.37-7.42 (6H, m), 7.66 (4H, m); 13C NMR δ (125 MHz, CDCl3, 0.107 M) ppm: 5.00 (3C), 6.91 (3C), 19.21, 26.85 (3C), 28.46, 33.57, 40.17, 46.92, 49.57, 63.94, 70.16, 127.60 (4C), 129.53 (2C), 134.01 (2C), 135.56 (4C); HR-FAB-MS (matrix: 3-nitrobenzyl alcohol): m/z 499.3057 (MH+, calcd for C29H47O3Si2, 499.3064).
Synthesis of 8. To a solution of [(4-(1,3-dithian-2-yl)-4-methylpentyl)oxy]triisopropylsilane15 (2.59 g, 7.06 mmol) in THF (20 mL) was added 1 M tetrabutylammonium fluoride in THF (9.2 mL, 9.2 mmol, 1.5 equiv.) at 4 °C. After 1.5 h of stirring at rt, the reaction was quenched with saturated aq. NaHCO3 (80 mL), and the mixture was extracted with EtOAc. The combined organic layers were washed with brine, dried over Na2SO4, filtered, and concentrated in vacuo. The residue was purified by column chromatography (silica gel, 30% EtOAc/hexane) to afford an alcohol. To a solution of the alcohol in CH2Cl2 (15 mL) were added PPTS (357 mg, 1.42 mmol, 0.2 equiv.) and dihydropyran (0.72 mL, 8.0 mmol, 1.25 equiv.). After 40 h of stirring at rt, the reaction was quenched with saturated aq. NaHCO3 (30 mL), and the mixture was extracted with EtOAc. The combined organic layers were washed with brine, dried over Na2SO4, filtered, and concentrated in vacuo. The residue was purified by column chromatography (silica gel, 4% EtOAc/hexane) to yield 8 (1.76 g, 5.79 mmol, 82% in 2 steps) as clear oil. Compound 8 (a racemic mixture): 1H NMR δ (400 MHz, CDCl3, 0.071 M) ppm: 1.09 (6H, s), 1.47-1.63 (8H, m), 1.69-1.85 (3H, m), 2.05-2.09 (1H, m), 2.88 (4H, dd, J = 7.9, 3.3 Hz), 3.38 (1H, dt, J = 9.4, 6.9 Hz), 3.50 (1H, m), 3.72 (1H, dt, J = 9.4, 7.0 Hz), 3.88 (1H, m), 4.03 (1H, s), 4.58 (1H, dd, J = 4.4, 2.7 Hz); 13C NMR δ (125 MHz, CDCl3, 0.141 M) ppm: 19.76, 24.21, 25.40 (2C), 25.50, 26.18, 30.78, 31.45 (2C), 36.57, 37.96, 60.68, 62.49, 68.06, 98.89; HR-EI-MS: m/z 304.1537 (M+, calcd for C15H28O2S2, 304.1531).
Synthesis of the carboxylic acid unit 9. To a solution of (R)-1-(benzyloxy)-3-(1,3-dithian- 2-yl)propan-2-ol24 (560 mg, 1.97 mmol) in THF (15 mL) were added imidazole (400 mg, 5.88 mmol, 3.0 equiv.) and TES-Cl (0.50 mL, 3.0 mmol, 1.5 equiv.) at rt. After 1.5 h of stirring at rt under an Ar atmosphere, the reaction was quenched with brine (25 mL), and the reaction mixture was extracted with EtOAc. The combined organic layers were dried over Na2SO4, filtered, and concentrated in vacuo. The residue was purified by column chromatography (silica gel, 5% EtOAc/hexane) to afford a dithiane. To a solution of the dithiane in MeCN (16 mL) and H2O (4.0 mL) were added NaHCO3 (440 mg, 5.2 mmol, 2.9 equiv.) and methyl iodide (4.39 mL, 70.4 mmol, 39 equiv.). After 18 h of stirring at rt, the reaction was poured into H2O (40 mL), and the mixture was extracted with EtOAc. The combined organic layers were washed with brine (50 mL), dried over Na2SO4, filtered, and concentrated in vacuo. The residue was purified by column chromatography (silica gel, 3% EtOAc/hexane) to afford an aldehyde (475 mg). To a solution of the aldehyde (20.0 mg, 0.065 mmol) in t-BuOH (0.5 mL) was added 2-methyl-2-butene (0.33 mL, 0.066 mmol, 10 equiv.), and then cooled at 4 °C. To the reaction mixture, a solution of NaClO4 (9.5 mg, 0.091 mmol, 1.4 equiv.) in saturated aq. NaH2PO4 (0.5 mL) was added, and the mixture was stirred for 30 min at 4 °C. After being stirred for 1.5 h at rt, the mixture was poured into H2O (8 mL), and extracted with EtOAc. The combined organic layers were washed with brine, dried over Na2SO4, filtered, and concentrated in vacuo. The residue was purified by column chromatography (silica gel, 15% EtOAc/hexane) to yield 9 (18.5 mg, 0.0571 mmol, 73%) as yellow oil. Since 9 was slightly labile at rt, it was prepared just before use and stored at –78 °C. Compound 9: [α]D +16.9° (c 1.00, CHCl3, 8.9 °C); 1H NMR δ (400 MHz, CDCl3, 0.12 M) ppm: 0.60 (6H, q, J = 7.9 Hz), 0.93 (9H, t, J = 7.9 Hz), 2.52 (1H, dd, J = 15.4, 7.0 Hz), 2.69 (1H, dd, J = 15.4, 5.0 Hz), 3.40 (1H, dd, J = 9.6, 6.2 Hz), 3.49 (1H, dd, J = 9.6, 5.1 Hz), 4.29 (1H, m), 4.53 (2H, s), 7.32 (5H, m); 13C NMR δ (125 MHz, CDCl3, 0.099 M) ppm: 4.82 (3C), 6.69 (3C), 39.97, 68.24, 73.44, 73.77, 127.67 (2C), 127.69, 128.39 (2C), 138.00, 176.12; HR-FAB-MS (matrix: glycerol): m/z 325.1827 (MH+, calcd for C17H29O4Si, 325.1835).
Synthesis of the key intermediate 6. To a solution of 8 (45 mg, 0.15 mmol, 1.9 equiv.) in THF (0.3 mL) was added 1.6 M n-BuLi in hexane (110 µL, 0.176 mmol, 2.2 equiv.) under an Ar atmosphere. After 1 h of stirring at rt, the reaction was cooled at 4 °C. A solution of 7 (40 mg, 0.08 mmol) in THF (0.25 mL) was then added, and the reaction mixture was stirred for 3 h at 4 °C. The reaction was quenched with saturated aq. NH4Cl (2.5 mL), and the reaction mixture was extracted with EtOAc. The combined organic layers were washed with brine, dried over Na2SO4, filtered, and concentrated in vacuo. The residue was purified by column chromatography (silica gel, 4.5% EtOAc/hexane) to yield 13 (55 mg, 0.068 mmol, 85%) as clear oil. This procedure was repeated 47 times to give 2.46 g of 13. Compound 13 (a diastereomeric mixture); 1H NMR δ (500 MHz, CDCl3, 0.030 M) ppm: 0.61 (6H, q, J = 8.2 Hz), 0.96 (9H, t, J = 8.0 Hz), 1.04 (9H, s), 1.11 (3H, s), 1.13 (3H, s), 1.53-1.72 (15H, m), 1.82-1.91 (3H, m), 2.02 (1H, dd, J = 15.6, 1.5 Hz), 2.12 (1H, dd, J = 15.6, 8.0 Hz), 2.76-2.98 (4H, m), 3.38 (1H, m), 3.50 (1H, m), 3.67 (2H, t, J = 6.0 Hz), 3.73 (1H, m), 3.87 (1H, m), 3.96 (1H, m), 4.11 (1H, s, OH), 4.33 (1H, br.t, J = 6.6 Hz), 4.58 (1H, t, J = 3.6 Hz), 7.36-7.43 (6H, m), 7.67 (4H, m); 13C NMR δ (125 MHz, CDCl3, 0.030 M) ppm: 5.18 (3C), 6.96 (3C), 19.25, 19.70, 22.30, 22.43, 23.14, 25.34, 25.54, 26.90 (3C), 27.17, 27.42, 28.30, 30.80, 33.04, 33.30, 45.53, 45.63, 45.68, 62.38, 63.63, 64.18, 67.81, 68.32, 70.37, 98.86, 127.60 (4C), 129.51 (2C), 134.14 (2C), 135.60 (4C); Some of the 13C NMR signals were doubled according to the THP diastereomers (δ45.53 and 98.86); HR-FAB-MS (matrix: 3-nitrobenzyl alcohol): m/z 825.4410 (MNa+, calcd for C44H74O5S2Si2Na, 825.4414).
Compound 13 (2.46 g, 3.07 mmol) dissolved in AcOH, THF and H2O (6:2:1 v/v, 63 mL) was stirred for 1.5 h at 55 °C. The solution was concentrated in vacuo with toluene (15 mL). The residue was diluted with EtOAc (15 mL) and quenched with saturated aq. NaHCO3 (25 mL), and the mixture was extracted with EtOAc. The combined organic layers were washed with brine, dried over Na2SO4, filtered, and concentrated in vacuo. The residue was purified by column chromatography (silica gel, 50% EtOAc/hexane) to yield a triol. To a solution of the triol in CH2Cl2 (23 mL) were added 2,2-dimethoxypropane (3.50 mL, 28.8 mmol, 12 equiv.) and 10-camphorsulfonic acid (CSA, 55.3 mg, 0.238 mmol, 0.1 equiv.) at rt. The reaction mixture was stirred at rt for 2 h, and quenched with saturated aq. NaHCO3 (50 mL). The resultant mixture was extracted with EtOAc. The combined organic layers were washed with brine, dried over Na2SO4, and concentrated in vacuo. The residue was dissolved in CH2Cl2 (25 mL), and silica gel (WakogelTM C-200, 1.5 g, 1 equiv. v/v) was added to the solution. The mixture was stirred at rt for 2.5 h, and then filtered and concentrated in vacuo. The residue was purified by column chromatography (silica gel, 25% EtOAc/hexane) to yield 14 (1.33 g, 2.07 mmol, 67% in 2 steps) as clear oil. Compound 14: [α]D +1.5° (c 0.26, CHCl3, 14.0 °C); 1H NMR δ (400 MHz, CDCl3, 0.014 M) ppm: 1.05 (9H, s), 1.13 (6H, s), 1.34 (3H, s), 1.38 (3H, s), 1.52-1.72 (10H, m), 1.82 (1H, m), 2.02 (1H, m), 2.21 (1H, dd, J = 16.3, 2.2 Hz), 2.35 (1H, dd, J = 16.3, 5.3 Hz), 2.68 (2H, m), 2.84 (1H, m), 3.08 (1H, m), 3.62-3.68 (4H, dt, J = 12.4, 6.2 Hz), 3.81 (1H, m), 4.23 (1H, dt, J = 11.7, 2.5 Hz), 7.35-7.42 (6H, m), 7.66 (4H, m); 13C NMR δ (100 MHz, CDCl3, 0.062 M) ppm: 19.23, 19.60, 22.47, 22.62, 24.84, 26.89 (4C), 27.00, 28.01, 28.24, 30.30, 32.67, 32.93, 38.60, 43.40, 43.52, 63.44, 63.81 (2C), 68.04, 68.90, 98.52, 127.61 (4C), 129.54 (2C), 134.01, 134.04, 135.57 (4C); HR-FAB-MS (matrix: 3-nitrobenzyl alcohol): m/z 667.3302 (MNa+, calcd for C36H56O4S2SiNa, 667.3287).
To a solution of 14 (480 mg, 0.745 mmol) in CH2Cl2 (16 mL) were added N-methylmorpholine N-oxide (131 mg, 1.12 mmol, 1.5 equiv.) and molecular sieve 4Å (MS4Å, 480 mg, 1.0 equiv. w/w). Tetrapropylammonium perruthenate (TPAP, 12.2 mg, 0.0347 mmol, 4.69 mol%) was then added, and the reaction mixture was stirred for 30 min at rt. The mixture was concentrated in vacuo, and the residue was purified by column chromatography (silica gel, 10% EtOAc/hexane) to yield 15 (360 mg, 0.56 mmol, 75%) as clear oil. Compound 15: [α]D +2.9° (c 0.56, CHCl3, 23.3 °C); 1H NMR δ (400 MHz, CDCl3, 0.050 M) ppm: 1.05 (9H, s), 1.12 (6H, s), 1.34 (3H, s), 1.37 (3H, s), 1.51-1.72 (6H, m), 1.83 (1H, m), 2.05 (3H, m), 2.23 (1H, dd, J = 16.3, 2.0 Hz), 2.34 (1H, dd, J = 16.3, 5.4 Hz), 2.43 (2H, m), 2.70 (2H, m), 2.83 (1H, m), 3.07 (1H, m), 3.67 (2H, t, J = 6.3 Hz), 3.82 (1H, m), 4.23 (1H, dt, J = 11.7, 2.6 Hz), 7.35-7.42 (6H, m), 7.66 (4H, m), 9.78 (1H, t, J = 1.9 Hz); 13C NMR δ (100 MHz, CDCl3, 0.050 M) ppm: 19.23, 19.58, 22.61 (2C), 24.74, 26.84, 26.89 (3C), 27.04, 28.02, 29.34, 30.28, 32.69, 38.63, 40.09, 43.16, 43.65, 63.08, 63.80, 67.85, 68.85, 98.54, 127.60 (4C), 129.54 (2C), 134.01, 134.04, 135.57 (4C), 202.75; HR-FAB-MS (matrix: 3-nitrobenzyl alcohol): m/z 643.3340 (MH+, calcd for C36H55O4S2Si, 643.3311).
1 M TiCl4 in CH2Cl2 (0.37 mL, 0.37mmol, 0.32 equiv.) was diluted with CH2Cl2 (3.7 mL) at 4 °C under an Ar atmosphere. Ti(Oi-Pr)4 (0.34 mL, 1.2 mmol, 1.0 equiv.) was added, and the solution was stirred for 1 h at rt. Ag2O (232 mg, 1.00 mmol, 0.83 equiv.) was added, and the solution was stirred for 5 h at rt in the dark. (S)-(–)-1,1'-bi-2-naphthol (430 mg, 1.5 mmol, 1.3 equiv.) was added after the solution was diluted with CH2Cl2 (7.4 mL). Following 2 h of stirring at rt, the dark red mixture was added to a solution of 15 (742 mg, 1.15 mmol) in Et2O (11.1 mL) at −15 °C. Allyl-SnBu3 (10.4 mL, 33.6mmol, 30 equiv.) was added, and the mixture was stirred for 1 h at the same temperature. The resulting reaction mixture was kept in a freezer at −20 °C for 18 h without stirring, and then stirred for 2 h at 4 °C. The reaction was quenched with saturated aq. NH4Cl (25 mL), and the mixture was extracted with EtOAc. The combined organic layers were filtered and washed with brine, dried over Na2SO4, filtered, and concentrated in vacuo. The residue was purified by column chromatography (silica gel, 6% EtOAc/hexane; ODS, 95% MeOH) to yield 16 (390 mg, 0.57 mmol, 50%, 93% de) as clear oil. Diastereomeric excess was determined by the modified Mosher's method.35 Compound 16: [α]D +3.6° (c 0.80, CHCl3, 15.3 °C); 1H NMR δ (400 MHz, CDCl3, 0.018 M) ppm: 1.05 (9H, s), 1.12 (3H, s), 1.13 (3H, s), 1.34 (3H, s), 1.38 (3H, s), 1.44-1.69 (9H, m), 1.84 (2H, m), 1.98 (1H, m), 2.15 (1H, m), 2.22 (1H, dd, J = 16.3, 2.2 Hz), 2.35 (2H, m), 2.68 (2H, tt, J = 13.6, 4.4 Hz), 2.84 (1H, m), 3.08 (1H, m), 3.60 (1H, m), 3.67 (2H, t, J = 6.3 Hz), 3.80 (1H, m), 4.23 (1H, dt, J = 9.0, 2.5 Hz), 5.13 (1H, s), 5.16 (1H, m), 5.83 (1H, m), 7.36-7.44 (6H, m), 7.66 (4H, m); 13C NMR δ (100 MHz, CDCl3, 0.018 M) ppm: 19.25, 19.61, 22.48, 22.72, 24.86, 26.92 (3C), 26.96, 27.05, 28.06, 30.33, 32.06, 32.73, 32.95, 38.65, 41.87, 43.54, 43.64, 63.52, 63.86, 68.08, 68.93, 71.42, 98.55, 118.18, 127.61 (4C), 129.54 (2C), 134.08, 134.81, 135.01, 135.59 (4C); HR-FAB-MS (matrix: 3-nitrobenzyl alcohol): m/z 707.3589 (MNa+, calcd for C39H60O4S2SiNa, 707.3600).
Compound 16 (495 mg, 0.724 mmol) was dissolved in AcOH, THF, and H2O (4:2:1 v/v, 8 mL). The mixture was stirred for 50 min at 55 °C. The reaction was concentrated in vacuo with toluene. The residue was diluted with EtOAc (10 mL) and quenched with saturataed aq. NaHCO3 (20 mL), and the mixture was extracted with EtOAc. The combined organic layers were washed with brine, dried over Na2SO4, filtered, and concentrated in vacuo. The residue was purified by column chromatography (silica gel, 30 % EtOAc/hexane) to afford 17 (350 mg, 0.54 mmol, 75%) as a clear oil. Compound 17: [α]D +3.6° (c 1.32, CHCl3, 12.2 °C); 1H NMR δ (500 MHz, CDCl3, 0.011 M) ppm: 1.04 (9H, s), 1.13 (3H, s), 1.13 (3H, s), 1.44-1.73 (9H, m), 1.80 (1H, m), 1.93-2.00 (3H, m), 2.18 (2H, m), 2.33 (1H, m), 2.83-3.01 (4H, m), 3.62 (1H, m), 3.69 (2H, t, J = 6.2 Hz), 3.89 (1H, m), 4.04 (1H, br.s, OH), 4.52 (1H, m, OH), 4.56 (1H, br.s), 5.15 (2H, m), 5.83 (1H, m), 7.35-7.44 (6H, m), 7.67 (4H, m); 13C NMR δ (125 MHz, CDCl3, 0.011 M) ppm: 19.24, 22.39, 22.63, 23.07, 26.92 (3C), 27.36, 27.54, 28.61, 32.06, 32.75, 34.30, 42.09, 44.48, 45.12, 45.59, 63.21, 64.17, 71.10, 71.29, 71.54, 118.33, 127.63 (4C), 129.55 (2C), 134.04 (2C), 134.67, 135.62 (4C); HR-FAB-MS (matrix: 3-nitrobenzyl alcohol): m/z 645.3498 (MH+, calcd for C36H57O4S2Si, 645.3468).
To a solution of 17 (350 mg, 0.54 mmol) in MeCN (2.0 mL), CH2Cl2 (2.0 mL), and H2O (2.0 mL) was added Hg(ClO4)2・6H2O (545 mg, 1.08 mmol, 2.0 equiv.) at 4 °C. After 45 min of stirring at the same temperature, the reaction mixture was poured into EtOAc (15 mL) and saturated aq. Na2S2O3 (25 mL). The organic layer was separated, and the aqueous layer was extracted with EtOAc. The combined organic layers were washed with brine, dried over Na2SO4, filtered, and concentrated in vacuo. The residue was purified by column chromatography (5% → 10% EtOAc/hexane) to afford 18 (104 mg, 0.194 mmol, 36%) and 19 (133 mg, 0.248 mmol, 46%).
To a solution of 19 (148 mg, 0.276 mmol) in CH2Cl2 (6.0 mL) was added ZnCl2 (112 mg, 0.824 mmol, 3.0 equiv.) at rt. After 30 min of stirring at rt, the reaction was quenched with saturated aq. NaHCO3 (20 mL), and the mixture was extracted with EtOAc. The combined organic layers were washed with brine, dried over Na2SO4, filtered, and concentrated in vacuo. The residue was purified by column chromatography (silica gel, 10 % EtOAc/hexane) to afford 18 (134 mg, 0.250 mmol, 90%) as clear oil. Compound 18: [α]D +33.1° (c 0.735, CHCl3, 28.7 °C); 1H NMR δ (500 MHz, CDCl3, 0.056 M) ppm: 0.88 (3H, s), 0.95 (3H, s), 1.05 (9H, s), 1.34 (1H, m), 1.42-1.74 (10H, m), 2.20 (1H, m), 2.29 (2H, m), 3.69 (2H, t, J = 6.4 Hz), 3.78 (1H, t, J = 6.5 Hz), 3.81 (1H, d, J = 11.0 Hz, OH), 4.07 (1H, dt, J = 11.0, 3.1 Hz), 4.15 (1H, m), 5.09 (2H, m), 5.80 (1H, ddt, J = 17.2, 10.1, 7.2 Hz), 7.36-7.43 (6H, m), 7.67 (4H, m); 13C NMR δ (125 MHz, CDCl3, 0.060 M) ppm: 19.26, 22.46, 25.50, 26.37, 26.92 (3C), 28.42, 29.29, 32.26, 33.29, 36.76, 37.98, 40.87, 63.72, 64.08, 65.59, 72.59, 102.76, 117.88, 127.59 (4C), 129.50 (2C), 134.23 (2C), 134.81, 135.60 (4C); HR-FAB-MS (matrix: 3-nitrobenzyl alcohol): m/z 559.3204 (MNa+, calcd for C33H48O4SiNa, 559.3220). Compound 19: [α]D −5.2° (c 1.14, CHCl3, 32.9 °C); 1H NMR δ (500 MHz, CDCl3, 0.048 M) ppm: 0.85 (3H, s), 0.96 (3H, s), 1.05 (9H, s), 1.12 (1H, dt, J = 12.9, 3.3 Hz), 1.32-1.72 (8H, m), 1.89 (1H, td, J = 13.0, 5.0 Hz), 1.98 (1H, m), 2.05-2.20 (3H, m), 3.67 (2H, m), 3.79 (1H, m), 3.89 (1H, m), 4.22 (1H, m), 4.97 (2H, m), 5.75 (1H, ddt, J = 17.2, 10.1, 7.2 Hz), 7.35-7.47 (6H, m), 7.67 (4H, m); 13C NMR δ (125 MHz, CDCl3, 0.071 M) ppm: 19.23, 22.96, 25.70, 26.90 (3C), 27.47, 29.55, 32.07, 33.63, 36.21, 36.46, 38.02, 40.70, 62.42, 63.92, 68.68, 71.33, 102.29, 116.18, 127.61 (4C), 129.54 (2C), 134.12 (2C), 135.56, 135.59 (4C); HR-FAB-MS (matrix: 3-nitrobenzyl alcohol): m/z 559.3222 (MNa+, calcd for C33H48O4SiNa, 559.3220).
To a solution of 9 (260 mg, 0.80 mmol, 1.7 equiv.) in toluene (12.5 mL) were added Et3N (0.14 mL, 1.3 mmol, 2.8 equiv.) and 2,4,6-trichlorobenzoyl chloride (0.14 mL, 0.87 mmol, 1.9 equiv.). After 2 h of stirring at rt, the mixture was added to a solution of 18 (243 mg, 0.453 mmol) and DMAP (166 mg, 1.36 mmol, 3 equiv.) in toluene (12.5 mL) at rt. The mixture was heated to 50 °C and stirred for 1.5 h at the same temperature. The reaction mixture was poured into H2O (30 mL), and the mixture was extracted with EtOAc. The combined organic layers were washed with brine, dried over Na2SO4, filtered, and concentrated in vacuo. The residue was purified by column chromatography (silica gel, 3% EtOAc/hexane) to afford 20 (343 mg, 0.407 mmol, 90%) as clear oil. Compound 20: [α]D +22.3° (c 0.360, CHCl3, 14.7 °C); 1H NMR δ (400 MHz, CDCl3, 0.078 M) ppm: 0.59 (6H, q, J = 7.8 Hz), 0.84 (3H, s), 0.92 (9H, t, J = 7.8 Hz), 0.95 (3H, s), 1.05 (9H, s), 1.35-1.51 (7H, m), 1.64 (4H, m), 2.26 (3H, m), 2.49 (1H, dd, J = 15.3, 8.0 Hz), 2.60 (1H, dd, J = 15.3, 4.6 Hz), 3.37 (1H, dd, J = 9.5, 6.1 Hz), 3.46 (1H, br.s), 3.47 (1H, dd, J = 9.5, 5.3 Hz), 3.68 (2H, m), 4.23 (1H, m), 4.34 (1H, m), 4.52 (2H, d, J = 2.1 Hz), 4.96 (1H, d, J = 8.3 Hz), 5.00 (1H, d, J = 11.9 Hz), 5.09 (1H, t, J = 3.3 Hz), 5.82 (1H, ddt, J = 17.3, 10.2, 7.1 Hz), 7.27-7.43 (11H, m), 7.67 (4H, m); 13C NMR δ (125 MHz, CDCl3, 0.009 M) ppm: 4.92 (3C), 6.79 (3C), 19.26, 21.53, 25.55, 26.50, 26.59, 26.93 (3C), 28.31, 32.15, 34.24, 34.61, 36.88, 40.74, 41.06, 63.91, 64.14, 68.11, 68.44, 71.68, 73.33, 74.27, 100.07, 116.56, 127.58 (4C), 127.59 (3C), 128.33 (2C), 129.46 (2C), 134.30 (2C), 135.21, 135.60 (4C), 138.27, 171.68; HR-FAB-MS (matrix: 3-nitrobenzyl alcohol): m/z 865.4910 (MNa+, calcd for C50H74O7Si2Na, 865.4871).
To a suspension of NaIO4 (21.0 mg, 0.0981 mmol, 8.0 equiv.) in pH 7.2 buffer (1.0 mL) was added KMnO4 (1.93 mg, 0.0122 mmol, 1.0 equiv.) and stirred for 10 min at rt. The mixture was added to a solution of 20 (10.3 mg, 0.0122 mmol) in t-BuOH (1.0 mL). After 1 h of stirring at rt, the reaction was quenched with Na2S2O3 (5.9 mg). The resulting mixture was poured into EtOAc (8 mL) and H2O (8 mL). The organic layer was separated, and the aqueous layer was extracted with EtOAc. The combined organic layers were washed with brine, dried over Na2SO4, filtered, and concentrated in vacuo. The residue was purified by column chromatography (silica gel, 10% EtOAc/hexane containing 0.5% AcOH) to afford a carboxylic acid (6.9 mg). To a solution of the carboxylic acid (35.8 mg, 0.0416 mmol) in THF (2.0 mL) was added a mixture of HF・pyridine, pyridine, and THF (1:2:8 v/v, 1.7 mL). After 11.5 h of stirring at 4 °C, the reaction was diluted with H2O (5 mL) and warmed to rt. The mixture was extracted with EtOAc. The combined organic layers were washed with brine, dried over Na2SO4, filtered, and concentrated in vacuo. The residue was purified by column chromatography (silica gel, 70% EtOAc/hexane containing 0.5% AcOH) to afford 21 (16.4 mg, 0.032 mmol, 52% in 2 steps) as clear oil. Compound 21: [α]D +25.0° (c 0.90, CHCl3, 29.0 °C); 1H NMR δ (500 MHz, CDCl3, 0.065 M) ppm: 0.92 (3H, s), 0.99 (3H, s), 1.39-1.77 (11H, m), 2.09 (1H, br.d, J = 15.3 Hz), 2.47 (1H, dd, J = 14.4, 4.5 Hz), 2.56 (2H, m), 2.71 (1H, dd, J = 14.4, 8.2 Hz), 3.52 (2H, dd, J = 5.2, 1.0 Hz), 3.66 (2H, dd, J = 6.0, 4.4 Hz), 4.14 (1H, br.s), 4.27 (2H, m), 4.58 (2H, s), 5.18 (1H, s), 7.27-7.37 (5H, m); 13C NMR δ (125 MHz, CDCl3, 0.065 M) ppm: 22.95, 24.75, 26.47, 28.14, 28.36, 32.23 (2C), 34.09, 36.85, 39.20, 41.63, 62.65, 64.24, 67.48, 67.57, 69.64, 73.57 (2C), 101.35, 127.92 (2C), 127.93, 128.50 (2C), 137.58, 171.52, 173.38; HR-FAB-MS (matrix: glycerol): m/z 509.2743 (MH+, calcd for C27H41O9, 509.2751).
To a solution of 21 (17.0 mg, 0.0335 mmol) in toluene (5.5 mL) were added Et3N (20 mL, 0.2 mmol, 6.0 equiv.) and 2,4,6-trichlorobenzoyl chloride (5.8 µL, 0.037 mmol, 1.1 equiv.). After 3 h of stirring at rt, the mixture was diluted with toluene (16.5 mL). It was then added dropwise to a solution of DMAP (61.2 mg, 0.05 mmol, 15 equiv.) in toluene (45 mL) at 4 °C over 4.5 h. The resulting mixture was stirred at rt for an additional 30 min, and poured into water (40 mL). The mixture was extracted with EtOAc. The combined organic layers were washed with brine, dried over Na2SO4, filtered, and concentrated in vacuo. The residue was purified by column chromatography (silica gel, 40 % EtOAc/hexane) to afford 6 (7.0 mg, 0.014 mmol, 43%) as clear oil. Compound 6: [α]D +63.3° (c 0.41, CHCl3, 28.3 °C); 1H NMR δ (500 MHz, CDCl3, 0.017 M) ppm: 0.87 (3H, s, H3-15 or 16), 1.01 (3H, s, H3-15 or 16), 1.35-1.55 (7H, m, H2-4, H-5a, H2-10, H2-12), 1.59-1.73 (4H, m, H-5b, H-8a, H2-13), 1.84 (1H, br.s, OH), 2.34 (1H, dd, J = 12.7, 10.9 Hz, H-2a), 2.46 (1H, br.d, J = 15.6 Hz, H-8b), 2.52 (1H, dd, J = 12.7, 2.8 Hz, H-2b), 2.76 (1H, dd, J = 16.9, 3.0 Hz, H-18a), 2.89 (1H, dd, J = 16.9, 11.6 Hz, H-18b), 3.56 (1H, dd, J = 10.1, 5.5 Hz, H-20a), 3.63 (1H, dd, J = 10.1, 3.7 Hz, H-20b), 3.70 (2H, m, H2-14), 3.87 (1H, tt, J = 10.9, 2.9 Hz, H-3), 4.26 (1H, m, H-11), 4.50 (1H, d, J = 12.0 Hz, Bn), 4.57 (1H, d, J = 12.0 Hz, Bn), 5.20 (1H, br.s, H-9), 5.25 (1H, m, H-19), 7.33 (5H, m, Bn); 13C NMR δ (125 MHz, CDCl3, 0.017 M) ppm: 21.27 (C-15 or 16), 25.31 (C-8), 25.89 (C-15 or 16), 27.25 (C-4), 28.24 (C-13), 31.84 (C-12), 34.73 (C-5), 34.83 (C-10), 36.92 (C-6), 37.48 (C-18), 42.75 (C-2), 62.43 (C-14), 63.58 (C-11), 68.46 (C-9), 68.99 (C-19), 70.32 (C-20), 70.74 (C-3), 73.51 (Bn), 100.51 (C-7), 127.71 (2C, Bn), 127.85 (Bn), 128.47 (2C, Bn), 137.78 (Bn), 169.96 (C-17), 170.31 (C-1). These NMR signals were assigned by 1H-1H COSY, HMQC, and HMBC. HR-FAB-MS (matrix: 3-nitrobenzyl alcohol): m/z 491.2643 (MH+, calcd for C27H39O8, 491.2645).
Synthesis of the side chain unit 24. To a solution of 2,5-dibenzyloxybenzyl bromide (68 mg, 0.18 mmol) in toluene (4 mL) was added PPh3 (65 mg, 0.25 mmol, 1.4 equiv.) and the mixture was heated to 80 °C. After 20 h of stirring at the same temperature, a brown precipitate formed. Toluene was removed by decantation and the residue was triturated with ether. The white solid was separated by filtration and washed well with hexane to yield a hydroscopic salt (24) (65.4 mg, 0.101 mmol, 56%). Compound 24: mp. 85-100 °C ; 1H NMR δ (500 MHz, CDCl3, 0.27 M) ppm: 4.42 (2H, s), 4.89 (2H, s), 5.22 (2H, br.d, J = 13.6 Hz), 6.58 (1H, d, J = 8.8 Hz), 6.83 (1H, dt, J = 9.0, 2.9 Hz), 7.13 (3H, m), 7.23 (2H, m), 7.32-7.38 (6H, m), 7.50 (12H, m), 7.72 (3H, m); HR-FAB-MS (matrix: 3-nitrobenzyl alcohol): m/z 565.2297 ([M–Br]+, calcd for C39H34O2P, 565.2296).
Synthesis of 4 and 5. To a solution of 6 (3.6 mg, 7.3 µmol) in CH2Cl2 (0.8 mL) were added N-methylmorpholine-N-oxide (1.3 mg, 0.013 mmol, 1.8 equiv.) and MS4Å (4.0 mg, 1.1 equiv. w/w). Tetrapropylammonium perruthenate (0.13 mg, 0.373 µmol, 5 mol%) was then added, and the reaction mixture was stirred for 40 min at rt. The resultant mixture, loaded directly onto a silica gel column, was purified by column chromatography (silica gel, 40% EtOAc/hexane) to afford 22 (2.6 mg, 5.3 µmol, 73%) as clear oil. Compound 22: [α]D +55.1° (c 0.20, CHCl3, 29.1 °C); 1H NMR δ (500 MHz, CDCl3, 0.019 M) ppm: 0.85 (3H, s), 0.98 (3H, s), 1.34-1.72 (8H, m), 1.81 (1H, m), 2.33 (1H, dd, J = 12.7, 10.9 Hz), 2.49 (3H, m), 2.62 (1H, m), 2.76 (1H, dd, J = 16.9, 3.0 Hz), 2.89 (1H, dd, J = 16.9, 11.4 Hz), 3.56 (1H, dd, J = 10.1, 5.5 Hz), 3.64 (1H, dd, J = 10.1, 3.8 Hz), 3.86 (1H, tt, J = 11.0, 3.0 Hz), 4.20 (1H, m), 4.50 (1H, d, J = 12.0 Hz), 4.57 (1H, d, J = 12.0 Hz), 5.20 (2H, m), 7.27-7.36 (5H, m), 9.79 (1H, t, J = 1.5 Hz); 13C NMR δ (125 MHz, CDCl3, 0.012 M) ppm: 21.33, 25.22, 25.84, 27.24, 28.14, 34.56, 34.69, 36.90, 37.44, 40.12, 42.71, 63.51, 68.17, 68.97, 70.30, 70.76, 73.50, 100.39, 127.71 (2C), 127.85, 128.47 (2C), 137.77, 169.94, 170.27, 202.83; HR-FAB-MS (matrix: 3-nitrobenzyl alcohol): m/z 489.2480 (MH+, calcd for C27H37O8, 489.2488).
To a solution of the phosphonium bromide 2336 (21.5 mg, 0.04 mmol, 5.1 equiv.) in THF (0.4 mL) was added 1.6 M n-BuLi (20 µL, 0.032 mmol, 4.1 equiv.) at 4 °C. After 20 min of stirring at the same temperature, the solution of 22 (3.0 mg, 6.2 µmol) in THF (0.25 mL) was added to the mixture at −78°C. The reaction mixture was stirred for 3 h at the same temperature and warmed to 4 °C. After stirring for 30 min at 4 °C, the mixture was diluted with EtOAc (1.0 mL) and the reaction was quenched with saturated aq. NH4Cl (2.5 mL). The organic layer was separated, and the aqueous layer was extracted with EtOAc. The combined organic layers were washed with brine, dried over Na2SO4, filtered, and concentrated in vacuo. The residue was purified by column chromatography (silica gel, 15% EtOAc/hexane) to afford 25 (1.7 mg, 2.5 µmol, 41%) as clear oil. Compound 25: E-Alkene, 1H NMR δ (400 MHz, CDCl3, 0.004 M) ppm: 0.88 (3H, s), 1.02 (3H, s), 1.25-1.68 (10H, m), 2.31 (2H, m), 2.49 (2H, m), 2.76 (1H, dd, J = 12.3, 3.1 Hz), 2.88 (1H, dd, J = 16.9, 11.5Hz), 3.60 (2H, m), 3.87 (1H, m), 4.23 (1H, m), 4.48 (1H, d, J = 12.0 Hz), 4.57 (1H, d, J = 12.0 Hz), 5.04 (2H, s), 5.20 (2H, m), 6.14 (1H, dt, J = 15.8, 7.0 Hz), 6.33 (1H, d, J = 15.8 Hz), 6.90 (2H, d, J = 8.7 Hz), 7.24-7.44 (12H, m); Z-Alkene, 1H NMR δ (400 MHz, CDCl3, 0.004 M) ppm: 0.86 (3H, s), 0.95 (3H, s), 1.25-1.68 (10H, m), 2.31 (2H, m), 2.49 (2H, m), 2.72 (1H, dd, J = 12.3, 3.0 Hz), 2.88 (1H, dd, J = 16.8, 11.2Hz), 3.60 (2H, m), 3.87 (1H, m), 4.23 (1H, m), 4.50 (1H, d, J = 12.0 Hz), 4.57 (1H, d, J = 12.0 Hz), 5.06 (2H, s), 5.20 (2H, m), 5.60 (1H, dt, J = 11.6, 7.6 Hz), 6.35 (1H, d, J = 11.8 Hz), 6.93 (2H, d, J = 8.8 Hz), 7.24-7.44 (12H, m); HR-FAB-MS (matrix: 3-nitrobenzyl alcohol): m/z 669.3423 (MH+, calcd for C41H49O8, 669.3427).
To a solution of 25 (4.7 mg, 7.0 µmol) in EtOAc (0.7 mL) and MeOH (0.7 mL) was added Pd(OH)2/C (1.3 mg, 30% w/w). The mixture was vigorously stirred under an H2 atmosphere for 4.5 h. The reaction mixture was filtered and concentrated in vacuo. The residue was purified by HPLC (column: SL12S05-2510WT; solvent: i-PrOH : CHCl3 : hexane = 8:12:80; flow rate: 3.0 mL/min; pressure: 510 psi; UV detector: 254 nm; retention time: 22.0 min) to afford 4 (3.2 mg, 6.5 µmol, 93%) as clear oil. Compound 4; [α]D +40.7° (c 0.42, CHCl3, 29.3 °C); 1H NMR δ (500 MHz, CDCl3, 0.017 M) ppm: 0.86 (3H, s, H3-22 or 23), 0.98 (3H, s, H3-22 or 23), 1.34-1.49 (8H, m, H2-4, H2-5, H2-10, H2-13), 1.51-1.66 (5H, m, H-8a, H2-12, H2-14), 2.35 (1H, br.t, J = 5.6 Hz, OH), 2.40 (1H, dd, J = 12.9, 10.9 Hz, H-2a), 2.49 (1H, br.d, J = 15.6 Hz, H-8b), 2.54 (3H, m, H-2b, H2-15), 2.71 (1H, dd, J = 16.6, 3.3 Hz, H-25a), 2.79 (1H, dd, J = 16.6, 11.2 Hz, H-25b), 3.75 (2H, m, H2-27), 3.88 (1H, tt, J = 8.1, 2.8 Hz, H-3), 4.15 (1H, m, H-11), 5.01 (1H, br.s, Ph-OH), 5.18-5.22 (2H, m, H-9, H-26), 6.75 (2H, d, J = 8.5 Hz, H-18, H-20), 7.06 (2H, d, J = 8.4 Hz, H-17, H-21); 13C NMR δ (500 MHz, CDCl3, 0.017 M) ppm: 21.20 (C-22 or 23), 24.62 (C-13), 25.18 (C-8), 25.93 (C-22 or 23), 27.29 (C-4), 31.43 (C-14), 34.58 (C-12), 34.75 (C-15), 34.96 (C-10), 35.48 (C-5), 36.90 (C-25), 37.03 (C-6), 42.73 (C-2), 63.77 (C-11), 64.44 (C-27), 68.81 (C-9), 70.58 (C-3), 71.80 (C-26), 100.26 (C-7), 115.06 (2C, C-18, C-20), 129.49 (2C, C-17, C-21), 135.10 (C-16), 153.51 (C-19), 169.63 (C-24), 171.57 (C-1). These NMR signals were assigned by 1H-1H COSY, HSQC, and HMBC. HR-FAB-MS (matrix: 3-nitrobenzyl alcohol): m/z 491.2649 (MH+, calcd for C27H39O8, 491.2645).
To a solution of the phosphonium bromide 24 (27.0 mg, 0.042 mmol, 11.0 equiv.) in THF (0.5 mL) was added 1.6 M n-BuLi in hexane (20 µL, 0.032 mmol, 8.4 equiv.) at 4 °C. After stirring for 30 min at the same temperature, a portion of the mixture (0.25 mL) was added to a solution of the aldehyde 22 (1.7 mg, 3.5 µmol) in THF (0.15 mL) at −78°C. The mixture was stirred for 1.5 h at the same temperature, and warmed at −10 °C. After 1.5 h of stirring at −10 °C, the mixture was diluted with EtOAc (1.0 mL), and the reaction was quenched with saturated aq. NH4Cl (2.5 mL). The organic layer was separated, and the aqueous layer was extracted with EtOAc. The combined organic layers were washed with brine, dried over Na2SO4, filtered, and concentrated in vacuo. The residue was purified by column chromatography (silica gel, 20% EtOAc/hexane) to afford 26 (1.1 mg, 1.4 µmol, 41%) as clear oil. Compound 26: E-Alkene, 1H NMR δ (400 MHz, CDCl3, 0.004 M) ppm: 0.88 (3H, s), 1.02 (3H, s), 1.33-1.68 (9H, m), 2.25-2.52 (5H, m), 2.70 (1H, dd, J = 6.5, 3.0 Hz), 2.86 (1H, dd, J = 11.4, 3.5 Hz), 3.56 (2H, m), 3.85 (1H, m), 4.22 (1H, m), 4.44 (1H, d, J = 12.0 Hz), 4.53 (1H, d, J = 12.0 Hz), 5.02 (4H, s), 5.20 (2H, m), 6.27 (1H, dt, J = 15.9, 7.0 Hz), 6.72-6.86 (3H, m), 6.94-7.44 (16H, m); Z-Alkene, 1H NMR δ (400 MHz, CDCl3, 0.004 M) ppm: 0.82 (3H, s), 0.95 (3H, s), 1.33-1.68 (9H, m), 2.25-2.52 (5H, m), 2.74 (1H, dd, J = 6.5, 3.0 Hz), 2.90 (1H, dd, J = 11.4, 3.5 Hz), 3.56 (2H, m), 3.85 (1H, m), 4.22 (1H, m), 4.48 (1H, d, J = 12.0 Hz), 4.56 (1H, d, J = 12.0 Hz), 5.02 (2H, s), 5.04 (2H, s), 5.20 (2H, m), 5.75 (1H, dt, J = 11.8, 7.3 Hz), 6.57 (1H, J = 11.8 Hz), 6.72-6.86 (2H, m), 6.94-7.44 (16H, m); HR-FAB-MS (matrix: 3-nitrobenzyl alcohol): m/z 774.3740 (M+, calcd for C48H54O9, 774.3768).
To a solution of 26 (2.5 mg, 3.2 µmol) in EtOAc (0.45 mL) and MeOH (0.45 mL) was added Pd(OH)2/C (1.0 mg, 40% w/w). The mixture was vigorously stirred under an H2 atmosphere for 2.5 h. The reaction mixture was filtered and concentrated in vacuo. The residue was purified by column chromatography (silica gel, 70% EtOAc/hexane) to afford 5 (1.6 mg, 3.2 µmol, quant.) as clear oil. Compound 5: [α]D +53.7° (c 0.14, CHCl3, 12.4 °C); 1H NMR δ (400 MHz, CDCl3, 0.006 M) ppm: 0.87 (3H, s, H3-22 or 23), 0.94 (3H, s, H3-22 or 23), 1.41 (7H, m, H2-4, H2-5, H-10a, H-12a, H-13a), 1.50-1.76 (6H, m, H-8a, H-10b, H-12b, H-13b, H2-14), 2.31 (1H, t, J = 6.0 Hz, 27-OH), 2.39-2.64 (5H, m, H2-2, H-8b, H2-15), 2.78 (2H, m, H2-25), 3.77 (2H, m, H2-27), 3.91 (1H, tt, J = 10.7, 3.1 Hz, H-3), 4.27 (1H, m, H-11), 4.89 (1H, s, 17-OH), 5.20 (2H, m, H-9, H-26), 5.75 (1H, s, 20-OH), 6.57 (1H, dd, J = 8.5, 3.0 Hz, H-19), 6.69 (1H, d, J = 8.5 Hz, H-18), 6.76 (1H, d, J = 2.9 Hz, H-21); 13C NMR δ (125 MHz, CDCl3, 0.006 M) ppm: 21.16 (C-22 or 23), 24.13 (C-13), 25.25 (C-8), 25.91 (C-22 or 23), 27.25 (C-4), 27.72 (C-14), 29.20 (C-15), 34.28 (C-12), 34.55 (C-5), 35.04 (C-10), 36.95 (C-6), 37.07 (C-25), 42.88 (C-2), 62.63 (C-11), 64.17 (C-27), 68.93 (C-9), 70.63 (C-3), 72.49 (C-26), 100.44 (C-7), 113.12 (C-19), 116.42 (C-21), 116.63 (C-18), 130.29 (C-16), 147.19 (C-17), 150.52 (C-20), 169.42 (C-24), 172.99 (C-1). These NMR signals were assigned by 1H-1H COSY, HSQC, and HMBC. HR-FAB-MS (matrix: glycerol): m/z 506.2508 (M+, calcd for C27H38O9, 506.2516).
Synthesis of 1. To a solution of 2-benzyloxybenzyl bromide (163 mg, 0.588 mmol) in toluene (8.5 mL) was added PPh3 (216 mg, 0.824 mmol, 1.4 equiv.) and heated to 110 °C. After 12.5 h of stirring at the same temperature, the reaction mixture was kept in a freezer at –20 °C for 1 h. The mixture was filtered and washed well with hexane to yield (3-benzyloxybenzyl)triphenylphosphonium bromide (310 mg, 0.59 mmol, quant.) as a white solid. (3-Benzyloxybenzyl)triphenylphosphonium bromide: mp. 228-233 °C; 1H NMR δ (500 MHz, CDCl3, 0.33 M) ppm: 4.78 (2H, s), 5.37 (2H, d, J = 14.4 Hz), 6.66 (1H, d, J = 7.5 Hz), 6.85 (2H, m), 7.02 (1H, t, J = 7.9 Hz), 7.33 (5H, m), 7.60 (6H, m), 7.75 (9H, m); HR-FAB-MS (matrix: 3-nitrobenzyl alcohol): m/z 459.1881 ([M–Br]+, calcd for C32H28OP, 459.1878).
To a solution of the phosphonium bromide (19.0 mg, 0.035 mmol, 5.7 equiv.) in THF (9 mL) was added 1.6 M n-BuLi in hexane (20 µL, 0.035 mmol, 5.2 equiv.) at 4 °C. After 30 min of stirring at the same temperature, a portion of the mixture (0.52 mL) was added to the aldehyde 22 (3.0 mg, 6.2 µmol) at –78 °C. The mixture was stirred for 2 h at the same temperature and warmed at 4 °C. After 4 h of stirring at 4 °C, the reaction mixture was diluted with EtOAc (1.0 mL) and the reaction was quenched with saturated aq. NH4Cl (2.5 mL). The organic layer was separated, and the aqueous layer was extracted with EtOAc. The combined organic layers were washed with brine, dried over Na2SO4, filtered, and concentrated in vacuo. The residue was purified by column chromatography (silica gel, 25% EtOAc/hexane) to afford a mixture of alkenes (1.0 mg, 1.5 µmol). To a solution of the alkenes (2.0 mg, 3.0 µmol) in EtOAc (0.5 mL) and MeOH (0.5 mL) was added Pd(OH)2/C (1.0 mg, 0.5 equiv. w/w). The mixture was vigorously stirred under an H2 atmosphere for 6 h. The reaction mixture was filtered and concentrated in vacuo. The residue was purified by column chromatography (silica gel, 40% EtOAc/hexane) to afford 1 (1.5 mg, 3.1 µmol, 25% in 2 steps) as clear oil. Compound 1: [α]D +43.6° (c 0.15, CHCl3, 15.7 °C: lit15, +47°); 1H NMR and FAB-MS data coincided with those reported previously.15
Inhibition of specific binding of [3H]PDBu to PKC C1 peptides. The binding of [3H]PDBu to the PKC C1 peptides (α-C1A, δ-C1A, and δ-C1B) was evaluated by the procedure of Sharkey and Blumberg27 with slight modifications as reported previously28 with 50 mM Tris-maleate buffer (pH 7.4 at 4 °C), 10 – 40 nM each PKC C1 peptide, 20 nM [3H]PDBu (19.6 Ci/mmol), 50 µg/mL 1,2-dioleoyl-sn- glycero-3-phospho-l-serine (Sigma), 3 mg/mL bovine γ -globulin (Sigma), and various concentrations of inhibitors. Binding affinity was evaluated on the basis of the concentration required to cause 50% inhibition of the specific binding of [3H]PDBu, IC50, which was calculated with Microsoft Excel. The inhibition constant, Ki, was calculated by the method of Sharkey and Blumberg.27
Anti-proliferative activity against a panel of 39 human cancer cell lines. A panel of 39 human cancer cell lines established by Yamori and coworkers32 according to the NCI method with modifications was employed, and cell growth inhibitory activity was measured as reported previously.32 In brief, the cells were plated in 96-well plates in RPMI 1640 medium supplemented with 5% fetal bovine serum and allowed to attach overnight. The cells were incubated with each test compound for 48 h. Cell growth was estimated by the sulforhodamine B assay. The 50% growth inhibition (GI50) parameter was calculated as reported previously.32 Absorbance for the control well (C) and the test well (T) were measured at 525 nm along with that for the test well at time 0 (T0). Cell growth inhibition (% growth) by each concentration of drug (10–8, 10–7, 10–6, 10–5, and 10–4 M) was calculated as 100[(T - T0)/(C - T0)] using the average of duplicate points. By processing these values, each GI50 value, defined as 100[(T - T0)/(C - T0)] = 50, was determined.
EBV-EA induction test. Human B-lymphoblastoid Raji cells (5 × 105 / mL) were incubated at 37 °C under a 5% CO2 atmosphere in 1 mL of RPMI 1640 medium (supplemented with 10% fetal bovine serum) with 4 mM sodium n-butyrate (a synergist) and 10, 100, or 1000 nM of each test compound for the induction test, or 100 nM of each test compound in the presence of 32 nM of TPA for the inhibition test. In the induction test, each test compound was added as 2 µL of a DMSO solution (5, 50, and 500 µM stock solution) along with 2 µL DMSO; the final DMSO concentration was 0.4%. In the inhibition test, TPA was added as 2 µL of a DMSO solution (16 µM stock solution), 10 min after the addition of each test compound (2 µL of a DMSO solution: 50 µM). After incubation for 48 h, smears were made from the cell suspension, and the EBV-EA-expressing cells were stained by a conventional indirect immunofluorescence technique with an NPC patient's serum (a gift from Kobe University) and FITC-labeled anti-human IgG (DAKO, Glostrup, Denmark) as reported previously.33,34 In each assay, at least 500 cells were counted and the proportion of the EA-positive cells was recorded. Cell viability exceeded 60% in each experiment.
ACKNOWLEDGEMENTS
This work was supported by a Grant-in-Aid for Scientific Research on Innovative Areas “Chemical Biology of Natural Products” (No. 23102011 to K.I.) from The Ministry of Education, Culture, Sports, Science and Technology, Japan. We also thank the Screening Committee for Anticancer Drugs supported by a Grant-in-aid for Scientific Research on Innovative Areas “Scientific Support Programs for Cancer Research” from The Ministry of Education, Culture, Sports, Science and Technology, Japan. This study was partly carried out with the JEOL JMS-700 MS spectrometer in the Joint Usage/Research Center (JURC) at the Institute for Chemical Research, Kyoto University.
References
1. Y. Nishizuka, Nature, 1984, 308, 693; CrossRef Y. Nishizuka, FASEB J., 1995, 9, 484.
2. A. C. Newton, Chem. Rev., 2001, 101, 2353. CrossRef
3. Y. Kishi and R. R. Rando, Acc. Chem. Res., 1998, 31, 163. CrossRef
4. G. R. Pettit, C. L. Herald, D. L. Doubek, D. L. Herald, E. Arnold, and J. Clardy, J. Am. Chem. Soc., 1982, 104, 6846. CrossRef
5. M. Gschwendt, G. Fürstenberger, S. Rose-John, M. Rogers, W. Kittstein, G. R. Pettit, C. L. Herald, and F. Marks, Carcinogenesis, 1988, 9, 555. CrossRef
6. J. D. Winegarden, A. M. Mauer, T. F. Gajewski, P. C. Hoffman, S. Krauss, C. M. Rudin, and E. E. Vokes, Lung Cancer, 2003, 39, 191; CrossRef M. L. Varterasian, R. M. Mohammad, M. S. Shurafa, K. Hulburd, P. A. Pemberton, D. H. Rodriguez, V. Spadoni, D. S. Eilender, A. Murgo, N. Wall, M. Dan, and A. M. Al-Katib, Clin. Cancer Res., 2000, 6, 825.
7. T. J. Nelson and D. L. Alkon, Trends Biochem. Sci., 2009, 34, 136. CrossRef
8. R. Mehla, S. Bivalkar-Mehla, R. Zhang, I. Handy, H. Albrecht, S. Giri, P. Nagarkatti, M. Nagarkatti, and A. Chauhan, PLoS One, 2010, 5, e11160. CrossRef
9. R. Mutter and M. Wills, Bioorg. Med. Chem., 2000, 8, 1841. CrossRef
10. B. M. Trost and G. Dong, Nature, 2008, 456, 485; CrossRef G. E. Keck, Y. B. Poudel, T. J. Cummins, A. Rudra, and J. A. Covel, J. Am. Chem. Soc., 2011, 133, 744; CrossRef P. A. Wender and A. J. Schrier, J. Am. Chem. Soc., 2011, 133, 9228. CrossRef
11. D. Schaar, L. Goodell, J. Aisner, X. X. Cui, Z. T. Han, R. Chang, J. Martin, S. Grospe, L. Dudek, J, Riley, J. Manago, Y. Li, E. H. Rubin, A. Conney, and R. K. Strair, Cancer Chemother. Pharmacol., 2006, 57, 789. CrossRef
12. A. P. Kozikowski, Y. Chen, T. Subhasish, N. E. Lewin, P. M. Blumberg, Z. Zhong, M. A. D'Annibale, W. L. Wang, Y. Sheng, and B. Langley, ChemMedChem, 2009, 4, 1095. CrossRef
13. S. Zayed, B. Sorg, and E. Hecker, Planta Med., 1984, 50, 65; CrossRef Z. Szállási, L. Krsmanovic, and P. M. Blumberg, Cancer Res., 1993, 53, 2507.
14. K. R. Gustafson, J. H. Cardellina, II, J. B. McMahon, R. J. Gulakowski, J. Ishitoya, Z. Szallasi, N. E. Lewin, P. M. Blumberg, O. S. Weislow, J. A. Beutler, R. W. Buckheit, G. M. Cragg, P. A. Cox, J. P. Bader, and M. R. Boyd, J. Med. Chem., 1992, 35, 1978. CrossRef
15. Y. Nakagawa, R. C. Yanagita, N. Hamada, A. Murakami, H. Takahashi, N. Saito, H. Nagai, and K. Irie, J. Am. Chem. Soc., 2009, 131, 7573. CrossRef
16. P. J. Reddig, N. E. Dreckschmidt, H. Ahrens, R. Simsiman, C.-P. Tseng, J. Zou, T. D. Oberley, and A. K. Verma, Cancer Res., 1999, 59, 5710.
17. Z. Lu, A. Hornia, Y.-W. Jiang, Q. Zang, S. Ohno, and D. A. Foster, Mol. Cell Biol., 1997, 17, 3418.
18. R. C. Yanagita, H. Kamachi, K. Tanaka, A. Murakami, Y. Nakagawa, H. Tokuda, H. Nagai, and K. Irie, Bioorg. Med. Chem. Lett., 2010, 20, 6064. CrossRef
19. G. Zhu and E. Negishi, Org. Lett., 2007, 9, 2771. CrossRef
20. H. Hanawa, D. Uraguchi, S. Konishi, T. Hashimoto, and K. Maruoka, Chem. Eur. J., 2003, 9, 4405. CrossRef
21. J. J. W. Duan and A. B. Smith, III, J. Org. Chem., 1993, 58, 3703. CrossRef
22. S. V. Ley, J. Norman, W. P. Griffith, and S. P. Marsden, Synthesis, 1994, 639. CrossRef
23. D. A. Evans, B. W. Trotter, P. J. Coleman, B. Côté, L. C. Dias, H. A. Rajapakse, and A. N. Tyler, Tetrahedron, 1999, 55, 8671. CrossRef
24. G. Solladie and J. Hutt, Tetrahedron Lett., 1987, 28, 797. CrossRef
25. B. S. Bal, W. E. Childers, Jr., and H. W. Pinnick, Tetrahedron, 1981, 37, 2091. CrossRef
26. J. Inanaga, K. Hirata, H. Saeki, T. Katsuki, and M. Yamaguchi, Bull. Chem. Soc. Jpn., 1979, 52, 1989. CrossRef
27. N. A. Sharkey and P. M. Blumberg, Cancer Res., 1985, 45, 19.
28. K. Irie, K. Oie, A. Nakahara, Y. Yanai, H. Ohigashi, P. A. Wender, H. Fukuda, H. Konishi, and U. Kikkawa, J. Am. Chem. Soc., 1998, 120, 9159; CrossRef M. Shindo, K. Irie, A. Nakahara, H. Ohigashi, H. Konishi, U. Kikkawa, H. Fukuda, and P. A. Wender, Bioorg. Med. Chem., 2001, 9, 2073. CrossRef
29. K. Bögi, P. S. Lorenzo, Z. Szállási, P. Ács, G. S. Wagner, and P. M. Blumberg, Cancer Res., 1998, 58, 1423.
30. A. M. Jeffrey and R. M. J. Liskamp, Proc. Natl. Acad. Sci. U. S. A., 1986, 83, 241. CrossRef
31. P. A. Wender, J. L. Baryza, C. E. Bennett, F. C. Bi, S. E. Brenner, M. O. Clarke, J. C. Horan, C. Kan, E. Lacôte, B. Lippa, P. G. Nell, and T. M. Turner, J. Am. Chem. Soc., 2002, 124, 13648. CrossRef
32. T. Yamori, A. Matsunaga, S. Sato, K. Yamazaki, A. Komi, K. Ishizu, I. Mita, H. Edatsugi, Y. Matsuba, K. Takezawa, O. Nakanishi, H. Kohno, Y. Nakajima, H. Komatsu, T. Andoh, and T. Tsuruo, Cancer Res., 1999, 59, 4042.
33. Y. Ito, S. Yanase, J. Fujita, T. Harayama, M. Takashima, and H. Imanaka, Cancer Lett., 1981, 13, 29. CrossRef
34. H. zur Hausen, G. W. Bornkamm, R. Schmidt, and E. Hecker, Proc. Natl. Acad. Sci. U. S. A., 1979, 76, 782. CrossRef
35. I. Ohtani, T. Kusumi, Y. Kashman, and H. Kakisawa, J. Am. Chem. Soc., 1991, 113, 4092. CrossRef
36. M. C. Carreño, R. D. Mazery, A. Urbano, F. Colobert, and G. Solladié, J. Org. Chem., 2003, 68, 7779. CrossRef