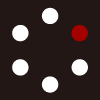
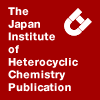
HETEROCYCLES
An International Journal for Reviews and Communications in Heterocyclic ChemistryWeb Edition ISSN: 1881-0942
Published online by The Japan Institute of Heterocyclic Chemistry
e-Journal
Full Text HTML
Received, 18th April, 2012, Accepted, 16th May, 2012, Published online, 17th May, 2012.
DOI: 10.3987/COM-12-12492
■ [1,2]-Wittig Rearrangement of Aromatic Heterocycles
Jingyue Yang, Apiwat Wangweerawong, and Gregory B. Dudley*
Department of Chemistry and Biochemistry, Florida State University, 12 Varsity Way, MC 4390, Tallahassee, U.S.A.
Abstract
Anionic rearrangement of diverse heteroaryl ethers is reported. In many cases, directed metallation followed by formal [1,2]-Wittig rearrangement provides heteroaryl carbinols in good yield.We recently described an unusual and unexpected [1,2]-anionic rearrangement of 2-benzyloxypyridines, in which pyridine-directed metallation of the benzyl ether triggers rearrangement to the α-pyridyl carbinol (1→2, Scheme 1).3 In a remarkable related process, pyridine-directed carbometallation of enol ether 3 results in an analogous rearrangement.4 The pyridine migration event common to both reactions may be classified as a [1,2]-Wittig rearrangement.5 However, typical [1,2]-Wittig rearrangements are believed to proceed through a dissociative mechanism involving migration of an alkyl or aryl radical species. In contrast, the associative mechanism proposed here involves transient hypervalency of the migrating atom, which more closely parallels the [1,2]-Brook rearrangement,6 as well as [1,2]-acyl migrations and related processes.7 Similar [1,4]-pyridyl migrations have been reported.8
Here we report new studies on the anionic rearrangement of aromatic heterocycles. Specifically, we explored: (1) the stereochemical fidelity of aryl migration and (2) scope of heterocycles that participate in this unusual process. Within the context of our proposed mechanism, two alternative stereochemical hypotheses were devised. If no achiral intermediates are involved, then stereochemical information from the starting secondary ether may translate to the tertiary alcohol product. Such an outcome would enable one to leverage enantioenriched secondary alcohols for the stereocontrolled synthesis of chiral tertiary alcohols. On the other hand, if the benzyllithium intermediate undergoes transient ionization, then the achiral benzyl anion would present an opportunity to guide the migration along either enantioface. In such a circumstance, stereochemical information could be increased by asymmetric induction.
Our experiments are more consistent with the latter hypothesis (Eq 1). We converted both enantiomers of 1-phenylethanol into pyridyl ethers R-1d and S-1d; racemic 1d was also prepared as a control. Anionic rearrangement under our previously reported conditions provided chiral tertiary alcohol 2d.3 Chiral HPLC analysis of the alcohol product was inconclusive, so acetylation of the hindered tertiary alcohol was performed using the Vedejs procedure.9 The resulting acetate (6) was resolvable by chiral HPLC; enantiomeric starting ethers provide antipodes of the product acetates, but the stereochemical information was highly eroded. We attribute the loss of stereochemical integrity to a high degree of ionic character in lithiated derivative of 1d, although other explanations such as a traditional [1,2]-Wittig mechanism involving a transient pair of radical intermediates cannot be ruled out. Preliminary attempts to bias the migration using chiral bases10a and additives10b have thus far been unsuccessful.
The second avenue of investigation related to the scope of heteroaromatic systems that participate in the migration (Table 1). 2-Alkoxypyridines had been the focus (entries 1-6),3,11 but other heteroaryl ethers can be employed using the same procedure (entries 7-16).12 Alkyl substituents at C3 (entry 8) and C5 (entry 7) are tolerated. The lepidyl (4-methylquinolyl) group does not migrate (entry 11) because C4 methyl deprotonation occurs preferentially. Electron-deficient systems including pyrazyl (entry 12) and 5-nitro-2-pyridyl (entry 10) decomposed under the reaction conditions, and 3-cyano-2-pyridyl underwent a different rearrangement to aminofuran 7 (entry 9). On the other hand, quinolyl and isoquinolyl groups showed excellent migratory aptitude (entries 13-15). The 4-pyridyl group also migrated (entry 16) as long as another heteroatom (cf. 2-MeO) is strategically located to direct metallation at the benzylic position.
In conclusion, we identified an unusual anionic migration of heteroaromatic rings in what is formally a [1,2]-Wittig rearrangement. Aryl ethers are typically poor substrates in traditional Wittig rearrangements, which are thought to proceed by dissociative radical mechanisms. The heteroaryl ethers in this study enable an associative (addition / elimination) mechanism. Such pathways should be considered to expand the scope of the [1,2]-Wittig rearrangement.13 Moreover, these specific examples12 provide a convenient alternative to the use of heteroaromatic nucleophiles14 for the synthesis of heteroaromatic carbinols.
EXPERIMENTAL
Representative procedure (Table 1, Entry 14): Quinolyl ether (100 mg, 1.0 equiv) in THF (1 mL) was added dropwise to a solution of LDA (1.3 equiv) in THF at room temperature, and the solution was stirred overnight. The resulting mixture was diluted with H2O (5 mL) and extracted with EtOAc (4 x 5 mL). The combined organic extracts were washed with brine, dried (Na2SO4), filtered, concentrated under vacuum, and purified on silica gel to yield 2j as a yellow solid (75%); mp 100-102 °C. 1H-NMR (400MHz, CDCl3) δ 8.10 (app. q, J = 8.6, 8.4, 8.2 Hz, 2H), 7.81-7.73 (m, 2H), 7.57-7.52 (m, 3H), 7.34-7.29 (m, 3H), 7.25-7.21 (m, 1H), 6.72 (bs, 1H), 2.01 (s, 3H); 13C NMR (100MHz, CDCl3) δ 164.3, 146.5, 145.5, 137.3, 129.9, 128.9, 128.3, 127.5, 127.2, 127.1, 126.6, 126.3, 118.5, 75.0, 28.6; IR (cm-1) 3359, 2978, 1619, 1599, 1505, 1446, 1370, 1309, 1216, 1128, 1067, 913, 830, 765, 751, 702; HRMS (EI+) Calcd for [C17H15ON]+: 249.1154, found: 249.1157.
References
1. Current address: Department of Chemistry, University of Texas, Austin, TX, USA.
2. Current address: Department of Chemistry, Yale University, New Haven, CT, USA.
3. J. Yang and G. B. Dudley, J. Org. Chem., 2009, 74, 7998. CrossRef
4. J. Yang and G. B. Dudley, Adv. Synth. Catal., 2010, 352, 3438. CrossRef
5. (a) J. A. Marshall, The Wittig rearrangement. ‘Comprehensive Organic Synthesis,’ ed. by B. M. Trost and I. Fleming, Pergamon, Oxford, 1991, Vol. 3, pp. 975–1014; CrossRef (b) K. Tomooka, H. Yamamoto, and T. Nakai, Liebigs Ann., 1997, 1275. CrossRef
6. A. G. Brook, Acc. Chem. Res., 1974, 7, 77. CrossRef
7. (a) M. P. Sibi and V. Snieckus, J. Org. Chem., 1983, 48, 1935; CrossRef (b) P. Zhang and R. E. Gawley, J. Org. Chem., 1993, 58, 3223; CrossRef (c) R. Worayuthakarn, S. Boonya-udtayan, E. Arom-oon, P. Ploypradith, S. Ruchirawat, and N. Thasana, J. Org. Chem., 2008, 73, 7432; CrossRef (d) A. Baroudi, J. Alicea, and I. V. Alabugin, Chem.-Eur. J., 2010, 16, 7683; CrossRef (e) A. Baroudi, J. Alicea, P. Flack, J. Kirincich, and I. V. Alabugin, J. Org. Chem., 2011, 76, 1521. CrossRef
8. (a) J. Clayden and U. Hennecke, Org. Lett., 2008, 10, 3567; CrossRef (b) For related migrations of other arene rings, see J. Clayden, W. Farnaby, D. M. Grainger, U. Hennecke, M. Mancinelli, D. J. Tetlow, I. H. Hillier, and M. A. Vincent, J. Am. Chem. Soc., 2009, 131, 3410; CrossRef (c) A. M. Fournier and J. Clayden, Org. Lett., 2012, 14, 142. CrossRef
9. E. Vedejs and O. Daugulis, J. Org. Chem., 1996, 61, 5702. CrossRef
10. (a) J. A. Marshall and J. Lebreton, Tetrahedron Lett., 1987, 28, 3323; CrossRef (b) S. Wu, S. Lee, and P. Beak, J. Am. Chem. Soc., 1996, 118, 715. CrossRef
11. Other studies involving 2-benzyloxypyridine and its derivatives: (a) M. R. Rosana, Y. Tao, A. E. Stiegman, and G. B. Dudley, Chem. Sci., 2012, 3, 1240; CrossRef (b) T. Wang, T. Intaranukulkit, M. R. Rosana, R. Slegeris, J. Simon, and G. B. Dudley, Org. Biomol. Chem., 2012, 10, 248; CrossRef (c) P. A. Albiniak and G. B. Dudley, Synlett, 2010, 841; CrossRef (d) K. W. C. Poon, P. A. Albiniak, and G. B. Dudley, Org. Synth., 2007, 84, 295; (e) E. O. Nwoye and G. B. Dudley, Chem. Commun., 2007, 1436; CrossRef (f) K. W. C. Poon and G. B. Dudley, J. Org. Chem., 2006, 71, 3923. CrossRef
12. See Supporting Information for characterization data and copies of 1H and 13C NMR spectra.
13. For a recent application of this strategy, see J. P. González, M. Edgar, M. R. J. Elsegood, and G. W. Weaver, Org. Biomol. Chem., 2011, 9, 2294. CrossRef
14. G. R. Dick, E. M. Woerly, and M. D. Burke, Angew. Chem. Int. Ed., 2012, 51, 2667. CrossRef