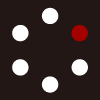
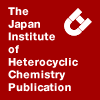
HETEROCYCLES
An International Journal for Reviews and Communications in Heterocyclic ChemistryWeb Edition ISSN: 1881-0942
Published online by The Japan Institute of Heterocyclic Chemistry
e-Journal
Full Text HTML
Received, 18th April, 2012, Accepted, 18th May, 2012, Published online, 18th May, 2012.
DOI: 10.3987/COM-12-12493
■ Synthesis of Substituted Dihydrobenzofurans and Bis-dihydrobenzofurans
Meng-Yang Chang,* Tein-Wei Lee, and Ming-Hao Wu
Department of Medicinal and Applied Chemistry, Kaohsiung Medical University, Kaohsiung 807, Taiwan, R.O.C.
Abstract
Several substituted dihydrobenzofurans and bis-dihydrobenzofurans were prepared in accepted yields from 4,4'-biphenyl via a series of reasonable transformations.Substituted 2,3-dihydrobenzofurans (coumaran) have played an important role in organic synthesis as a result of their high reactivity and suitability as starting materials for a wide range of synthetic heterocycles,1 natural products (e.g., botryomaman, bowdenol, sillusin, xyloketal J, corsifuran A, conocarpan),2 molecules with biological activities and other useful properties, as well as precursors of their derivatives.3 The most frequently employed methods for the preparation of 2,3-dihydrobenzofuran with a C2-methyl group involve IrCl3/AgOTf system,4a PPh3AuOTf,4b Sc(OTf)3/ionic liquid system,4c and HY zeolite4d catalyzed the one-pot tandem Claisen rearrangement—cyclization of a wide range of allyl aryl ethers (1). These literature strategies were easily turned to synthesize a series of 2-methyldihydrobenzofuran analogues (2) (Figure 1).5
Because the structural skeleton of bis-dihydrobenzofuran with different substituents were fewer synthesized,6,7 the further studies were needed for the involvement of substituent at the C2 or C3 position of bis-dihydrobenzofuran. In the course of our efforts with the synthetic application of 4,4’-biphenol (3),7d the skeleton (4) with different di-substituents on the C2 and C2’ position or mono-substitutent on the C2 position was chosen as the key focus. As shown in Figure 2, bis-dihydrobenzofuran had attracted increasing interest as synthetic targets due to their important activity as the pharmacophore (eurycomalin A, 5a),6a useful atropisomeric bisphosphine ligand (BICMAP, 5b) for Suzuki-Miyaura coupling.6b
Herein, a general synthetic route was examined for preparing several substituted skeleton (4) as shown in Scheme 1. These C2- and C2’-substituents (R1 group) included hydrogen, methyl, n-propyl, and benzyl group. First, double O-benzylation of 4,4’-biphenol (3) was treated with K2CO3 and benzyl bromide in acetone to provide 3,3’-dibenzyl-4,4’-biphenol in quantitative yield. AlCl3-mediated Friedel-Crafts alkylation reaction of the resulting product with 2-chloroacetaldehyde (2.0 equiv) gave compound (6a) with a 51% yield. Under the reaction conditions, the temperature should be controlled in -30 oC, otherwise compound (6a) was isolated in low yield (<30%) and polyalkylated regioisomers were yielded.
With the results in hand, synthesis of skeleton (4), with different substitutents, was next examined. Initially, treatment of model substrate (6a) with NaBH4 (for a) in MeOH afforded compound (7a) in 88% yield. Furthermore, a basic skeleton of bis-dihydrobenzofuran (4a) was provided by the two-step reaction with mesylation of alcohol (7a) (89%) followed by debenzylative annulation of compound (8a) (90%).8 In another way, attempts to extend this synthetic approach of bis-dihydrobenzofuran (4a) to Mitsunobu cyclization were unsatisfactory due to compound (4a) was only isolated in 44% yield via the hydrogenolysis of compound (7a) and the intramolecular etherification of the resulting diol compound.9
In comparison with two routes, the presented transformation of mesylation/debenzylative annulation provided a higher yield (80%) than hydrogenolysis/Mitsunobu annulation process did (44%). After changing the nucleophiles, three compounds (7b)~(7d) were provided in 76~89% yields by the Grignard addition. Under the above mentioned conditions, the reaction of compounds (7b)~(7d) was treated with mesyl chloride and triethylamine, the mesylated products (8b)~(8d) were isolated in 77~90% yields. Furthermore, compounds (4b)~(4d) were achieved in 85~91% yields via debenzylative annulation of compounds (8b)~(8d) with hydrogen in the presence of a catalytic amount of 10% palladium on activated carbon. According to the synthetic protocol, synthesis of four mono-dihydrobenzofurans (4e)~(4h) with different C2-substituent (R1 = H, Me, n-Pr, Bn) was achieved with good yield as shown in Scheme 2. The structural frameworks of compounds (4a) and (4e) were determined using single-crystal X-ray analysis (Figure 1).10
Changing the nucleophile (R1 group) from alkyl group to aromatic group was next studied. As shown in Scheme 3, Grignard addition of compound (6a) with phenylmagnesium bromide in THF provided compound (7e) in 80% yield. Compound (9) was isolated in 69% yield of two-steps via mesylation of compound (7e) and followed by hydrogenolysis of the resulting product.11 During the reaction condition, the skeleton of bis-dihydrobenzofuran was not observed.12
Furthermore, compound (6c) was also afforded in only 21% yield of two-steps via double O-benzylation and Friedel-Crafts alkylation with 2-chloropropanaldehyde (Scheme 4). Wittig mono-olefination of compound (6c) with Ph3P=CH2 (1.0 equiv, generated from Ph3PCHOMeCl and n-BuLi) was converted to olefinic aldehyde with a 62% yield. After NaBH4-mediated reduction of the corresponding aldehyde and mesylation, compound (8i) with an unsymmetrical skeleton was isolated from the symmetrical compound (6c) with a 76% yield of two-steps. Finally, debenzylative annulation of compound (8i) provided compound (4i) in 78% yield. Interestingly, compound (4i) was isolated as a sole diastereomer.
In summary, we have successfully presented a concise synthetic method for producing a series of novel bis-dihydrobenzofurans (4a)~(4d), mono-dihydrobenzofurans (4e)~(4i) involving the intermolecular Friedel-Crafts alkylation, nucleophilic addition, mesylation, and intramolecular debenzylative annulation. The overall prepared procedures are facile and simple for synthesizing the functionalized skeleton of bis-dihydrobenzofurans.
ACKNOWLEDGEMENTS
The authors would like to thank the National Science Council of the Republic of China for its financial support (NSC 99-2113-M-037-006-MY3).
References
1. For reviews on the synthesis of substituted dihydrobenzofurans, see: (a) X. L. Hou, H. Y. Cheung, T. Y. Hon, P. L. Kwan, T. H. Lo, S. Y. Tong, and H. N. C. Wong, Tetrahedron, 1998, 54, 1955; CrossRef (b) A. W. Erian, S. M. Sherif, and H. M. Gaber, Molecules, 2003, 8, 793; CrossRef (c) F. Bertolini and M. Pineschi, Org. Prep. Proced. Int., 2009, 41, 385. CrossRef
2. For botryomaman, see: (a) W. Pongcharoen, V. Rukachaisirikul, S. Phongpaichit, and J. Sakayaroj, Chem. Pharm. Bull., 2007, 55, 1404; CrossRef For bowdenol, see: (b) F. N. Melo, V. R. Navarro, M. S. Silva, E. V. Da-Cunha, J. M. Barbosa, and R. Barz-Filho, Nat. Prod. Lett., 2001, 15, 261; CrossRef For sillusin, see: (c) B.-S. Yun, H.-C. Kang, H. Koshino, S.-H. Yu, and I.-D. Yoo, J. Nat. Prod., 2001, 64, 1230; CrossRef For xyloketal J, see: (d) F. Xu, Y. Zhang, J. Wang, J. Pang, C. Huang, X. Wu, Z. She, L. L. P. Vrijmoed, E. B. G. Jones, and Y. Lin, J. Nat. Prod., 2008, 71, 1251; CrossRef For corsifuran A, see: (e) S. H. von Reuss and W. A. Konig, Phytochemistry, 2004, 65, 3113; CrossRef For conocarpan, see: (f) C. D. Chauret, C. B. Bernard, J. T. Arnason, and T. Durst, J. Nat. Prod., 1996, 59, 152. CrossRef
3. (a) A. P. Monte, D. Marona-Lewicka, N. V. Cozzi, and D. E. Nichols, J. Med. Chem., 1993, 36, 3700; CrossRef (b) D. E. Nichols, S. E. Snyder, R. Oberlender, M. P. Johnson, and X. M. Huang, J. Med. Chem., 1991, 34, 276; CrossRef (c) A. P. Monte, S. R. Waldman, D. Marona-Lewicka, D. B. Wainscott, D. L. Nelson, E. Sanders-Bush, and D. E. Nichols, J. Med. Chem., 1997, 40, 2997; CrossRef (d) J. M. Janusz, P. A. Young, M. W. Scherz, K. Enzweiler, L. I. Wu, L. Gan, S. Pikul, K. L. McDow-Dunham, C. R. Johnson, C. B. Senanayake, D. E. Kellstein, S. A. Green, J. L. Tulich, T. Rosario-Jansen, I. J. Magrisso, K. R. Wehmeyer, D. L. Kuhlenbeck, T. H. Eichhold, and R. L. M. Dobson, J. Med. Chem., 1998, 41, 1124; CrossRef (e) L. Piters, S. van Dyck, M. Gao, R. Bai, E. Hamel, A. Vlietinck, and G. Lemiere, J. Med. Chem., 1999, 42, 5475; CrossRef (f) G. Q. Shi, J. F. Dropinski, Y. Zhang, C. Santini, S. P. Sahoo, J. P. Berger, K. L. MacNaul, G. C. Zhou, A. Agrawal, R. Alvaro, T. Q. Cai, M. Hernadez, S. D. Wright, D. E. Moller, J. V. Heck, and P. T. Meinke, J. Med. Chem., 2005, 48, 5589; CrossRef (g) K. Kataoka, T. Shiota, T. Takeyasu, T. Minoshima, K. Watanabe, H. Tanaka, T. Mochizuki, K. Taneda, M. Ota, H. Tanabe, and H. Yamaguchi, J. Med. Chem., 1996, 39, 1262; CrossRef (h) P. S. Luize, T. Ueda-Nakamura, B. P. D. Filho, D. A. G. Cortez, and C. V. Nakamura, Bio. Pharm. Bull., 2006, 29, 2126. CrossRef
4. (a) V. H. Grant and B. Liu, Tetrahedron Lett., 2005, 46, 1237; CrossRef (b) N. W. Reich, C.-G. Yang, Z. Shi, and C. He, Synlett, 2006, 1278; CrossRef (c) F. Zulfiqar and T. Kitazume, Green Chem., 2000, 2, 296; CrossRef (d) H. J. Kim, J.-N. Kim, and K. H. Choi, Bull. Korean Chem. Soc., 2004, 25, 1726. CrossRef
5. S. Akai, N. Morita, K. Iio, Y. Nakamura, and Y. Kita, Org. Lett., 2000, 2, 2279. CrossRef
6. (a) P.-C. Kuo, C.-R. Su, A. G. Damu, and T.-S. Wu, Heterocycles, 2004, 63, 2123; CrossRef (b) T, Mino, Y. Naruse, S. Kobayashi, S. Oishi, M. Sakamoto, and T. Fujita, Tetrahedron Lett., 2009, 50, 2239. CrossRef
7. (a) A. M. Costero, C. Andreu, E. Monrabal, A. Tortajada, L. E. Ochando, and J. M. Amigo, Tetrahedron, 1996, 52, 12499; CrossRef (b) Y. Imai, J. Kitazawa, T. Sato, N. Tajima, R. Kuroda, Y. Matsubara, and Z.-i. Yoshida, Tetrahedron, 2007, 63, 1995; CrossRef (c) G. Angelovski and P. Eilbracht, Tetrahedron, 2003, 59, 8265; CrossRef (d) M.-Y. Chang, T.-W. Lee, and M.-H. Wu, Org. Lett., 2012, 14, 2198. CrossRef
8. A representative synthetic procedure of skeleton (4) is as follows: Palladium on activated carbon (10%, 20 mg) was added to a solution of skeleton (8) (0.5 mmol) in EtOAc (30 mL) at rt. Then hydrogen was bubbled into the mixture for 10 min, and the mixture was stirred at rt for 20 h. The reaction mixture was filtered and evaporated to yield crude product. Purification on silica gel (hexanes/EtOAc = 6/1) afforded skeleton (4). Representative data for compound (4a): Yield 90% (177 mg); Colorless solid; Mp 141-142 oC (recrystallized from hexanes and EtOAc); HRMS (ESI, M++1) calcd for C25H31O4 395.2222, found 395.2220; 1H NMR (400 MHz): δ 7.36 (br s, 2H), 7.27 (dd, J = 1.2, 8.4 Hz, 2H), 6.82 (d, J = 8.4 Hz, 2H), 4.60 (t, J = 8.8 Hz, 4H), 3.25 (t, J = 8.8 Hz, 4H); 13C NMR (100 MHz): δ 159.13 (2x), 134.19 (2x), 127.45 (2x), 126.62 (2x), 123.46 (2x), 109.31 (2x), 71.35 (2x), 29.79 (2x); Anal. Calcd for C25H30O4: C, 76.11; H, 7.66. Found: C, 76.29; H, 7.91. Single-crystal X-ray diagram: crystal of compound (4a) was grown by slow diffusion of EtOAc into a solution of compound (4a) in DCM to yield colorless prisms. The compound crystallizes in the triclinic crystal system, space group P 1 21/c 1, a=5.8246(2) Å, b=12.8801(4) Å, c=7.7281(3) Å, V=572.71(3) Å3, Z=2, dcalcd=1.382 g/cm3, F(000)=252, 2θ range 3.88~26.37o, R indices (all data) R1 = 0.0724, wR2 = 0.1211. For compound (4e): Yield 80% (85 mg); Colorless solid; Mp 172-173 oC (recrystallized from hexanes and EtOAc); HRMS (ESI, M++1) calcd for C14H13O2 213.0916, found 213.0922; 1H NMR (400 MHz): δ 7.42-7.36 (m, 3H), 7.29-7.26 (m, 1H), 6.89-6.86 (m, 2H), 6.83 (d, J = 8.4 Hz, 1H), 4.83 (br s, 1H), 4.61 (t, J = 8.8 Hz, 2H), 3.26 (t, J = 8.8 Hz, 2H); 13C NMR (100 MHz): δ 159.25, 154.49, 134.24, 133.63, 128.00 (2x), 127.52, 126.59, 123.40, 115.54 (2x), 109.38, 71.39, 29.78; Anal. Calcd for C14H12O2: C, 79.22; H, 5.70. Found: C, 79.47; H, 5.89. Single-crystal X-ray diagram: crystal of compound (4e) was grown by slow diffusion of EtOAc into a solution of compound (4e) in DCM to yield colorless prisms. The compound crystallizes in the monoclinic crystal system, space group P 1 21/c 1, a=8.4518(13) Å, b=6.1787(9) Å, c=21.735(3) Å, V=1063.0(3) Å3, Z=4, dcalcd=1.326 g/cm3, F(000)=448, 2θ range 2.00~26.63o, R indices (all data) R1 = 0.0920, wR2 = 0.1328.
9. However, the releted resolutions of the meso and dl isomers had been not observed from the NMR spectrum. Perhaps the given distance between the stereocenters was so long that the free rotation of bonds in between was easily generated.
10. CCDC860452 (4a) and 860092 (4e) contain the supplementary crystallographic data for this paper. This data can be obtained free of charge via www.ccdc.cam.ac.uk/conts/retrieving.html (or from the CCDC, 12 Union Road, Cambridge CB2 1EZ, UK; fax: 44-1223-336033; e-mail: deposit@ccdc.cam.ac.uk).
11. In particular, two signals of CH2CH2 group on the compound (9) were determined as the singlet by the 1H-NMR analysis (δ = 2.95, s, 8H). For the 13C-NMR analysis of CH2CH2 group, two kinds of CH2 signals were arranged as 36.31 and 32.48, respectively. From this observation, we understood that two overlapped proton signals of CH2 group could form a singlet peak.
12. F. Cullmann, H. Becker, E. Pandolfi, E. Roeckner, and T. Eicher, Phytochemistry, 1997, 45, 1235. CrossRef