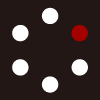
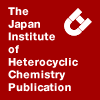
HETEROCYCLES
An International Journal for Reviews and Communications in Heterocyclic ChemistryWeb Edition ISSN: 1881-0942
Published online by The Japan Institute of Heterocyclic Chemistry
e-Journal
Full Text HTML
Received, 25th July, 2012, Accepted, 24th August, 2012, Published online, 27th August, 2012.
DOI: 10.3987/REV-12-SR(N)5
■ Distribution of Cyclic and Acyclic Bis-bibenzyls in the Marchantiophyta (Liverworts), Ferns and Higher Plants and Their Biological Activities, Biosynthesis, and Total Synthesis
Yoshinori Asakawa* and Agnieszka Ludwiczuk
Faculty of Pharmaceutical Sciences, Tokushima Bunri University, 180 Nishihamabouji, Yamashiro-machi, Tokushima, 770-8514, Japan
Abstract
The Marchantiophyta (liverworts) are rich sources of cyclic and acyclic bis-bibenzyls which are very rare natural products in the plant kingdom. At present more than 70 of bis-bibenzyls have been found in liverworts. The structurally unique cyclic and acyclic bis-bibenzyls shows various biological activities such as antimicrobial, antifungal, cytotoxicity, antiobesitic, muscle relaxation, antitripanosomal activity, among others. They are biosynthesized from dimerization of lunularic acid via dihydrocoumaric acid and prelunularin. The present paper deals with the distribution of bis-bibenzyls in liverworts, fern and higher plants and their biological activity, biosynthesis, and total synthesis.INTRODUCTION
The bryophytes (mosses, liverworts and hornworts) have not been studied chemically since almost one century because they are generally very tiny, and their identification and collection of a large amount are very difficult. Among three classes, only liverworts contain beautiful oil bodies in the cells. The bryophytes are distributed everywhere in the world except in the sea. The most charming countries of the bryophytes are New Zealand, Argentina and Japan. There are so many endemic genera in Southern hemispheric countries. On contrary, in North America, Europe and Africa, the distribution of the endemic genus of bryophytes is very poor. The bryophytes have been considered to be useless for human diets, however, 24 species of the bryophytes have been used in China as medicinal plants.1 For example, one of the very popular liverwort, Marchantia polymorpha shows antipyretic, antidotal, antihepatic and diuretic activity. Conocephalum conicum also demonstrates antimicrobial and antifungal activity as well as elimination of gall stone. Frullania tamarisci and Reboulia hemisphaerica contains antiseptic and hemostatic activity, respectively. Although several liverworts possess such pharmacologically important substances, their isolation was neglected for one century.
In this paper, the distribution of cyclic- and acyclic bis-bibenzyls in liverworts, their biological activity, biosynthesis, and total synthesis are reported.
1. DISTRIBUTION OF CYCLIC AND ACYCLIC BIS-BIBENZYLS IN THE PLANT KINGDOM
The occurrence, conformation, biosynthesis, biological activity, and synthesis of bis-bibenzyls have been reviewed by Asakawa’s group,2-5 and Keseru and Nogradi.6,7 Such macrocyclic and acyclic bis-bibenzyls are very rare plant metabolites possessing structures that occur exclusively in the Marchantiophyta. Such characteristic bis-bibenzyls are not only very significant chemical markers of several Marchantiophyta families but also important for considering the phylogeny of the bryophytes and the evolutionary processes of the lower terrestrial spore-forming plants.
In Table 1, the distribution of cyclic and acyclic bis-bibenzyls has been shown. Since two cyclic bis-bibenzyls, riccardin A (1) and marchantin A (14) were isolated from the thalloid liverworts, Riccardia multifida and Marchantia polymorpha, more than 70 bis-bibenzyls have been isolated from the Jungermanniales, Metzgeriales and Marchantiales species.5,8 The isolated bis-bibenzyls are divided into two groups, cyclic and acyclic. The cyclic bis-bibenzyls are further divided into six groups, where bibenzyls are connected with 1) one ether (C1-C2’) and one biphenyl linkage (C12-C10’, C14-C10’, C14-C12’); 2) one ether (C14-C11’) and one biphenyl (C6-C2’); 3) one ether (C6-C2’) and one biphenyl (C13-C10’); 4) two ethers (C1-C2’ and C13-C12’, C14-C11’, C13-C10’, C12-C11’); 5) two biphenyls (C6-C2’ and C14-C12’) and 6) two biphenyls (C6-C3’ and C14-C11’). Among these type 4 (two ether linkage between C1-C2’ and C14-C11’) and type 5 (two biphenyls between C6-C2’ and C14-C12’) are most abundant. The former type is distributed in the Marchantiales and the latter in the Jungermanniales.
Among riccardin type bis-bibenzyls (type 1), riccardin C (3) have been distributed in the Marchantiaceae and Plagiochasma species belonging to the Aytoniaceae. The type five, where one biphenyl connected between C6-C2’ and second between C14-C12’ is distributed in the stem-leafy liverwort, Plagiochila fruticosa collected in the southern Shikoku, Japan.
Plagiochin- and isoplagiochin types (type 1 and 2) of bis-bibenzyls have been distributed in the Plagiochila, Heteroscyphus and Herbertus species belonging to the Jungermanniales. Cyclic bis-bibenzyls are restricted to Aneuraceae, Blasiaceae which belong to the Metzgeriales, and the Aytoniaceae, Marchantiaceae, Monocleaceae and Ricciaceae belonging to the Marchantiales.
Since 1995, 14 acyclic bis-bibenzyls (87-102) have been isolated from the Marchantiophyta and their structures elucidated. These are mainly distributed in the Radula, Plagiochila, Nardia and Jungermannia belonging to the Jungermanniales, Pellia to the Metzgeriales, and Marchantia and Lunularia to the Marchantiales.
The crude extract of the higher plant, Primula macrocalyx (Primulaceae), which has been used in folk medicine to treat paralysis, scurvy, tuberculosis, and fever was fractionated by column chromatography using a reversed-phase resin to give bis-bibenzyl identified as riccardin C (3). 67 This is the first record of the isolation of the macrocyclic bis-bibenzyl from a higher plant. On the other hand, the acyclic bis-bibenzyl, perrottetin H (92a), has been isolated from the Japanese fern, Hymenophyllum barbatum. 68 Such characteristic bis-bibenzyls are not only very significant chemical markers of several Marchantiophyta families but also important for considering the phylogeny of the bryophytes and the evolutionary processes of the lower terrestrial spore-forming plants.
2. BIOLOGICAL ACTIVITY OF BIS-BIBENZYLS
In earlier reviews,2,3 chemical constituents isolated from liverworts (Marchantiophyta) were discussed, possessing characteristic fragrances, bitterness, pungency, and sweetness as well as allergenic contact dermatitis, cytotoxic, antimicrobial, antifungal, calmodulin inhibitory, cardiotonic, insect antifeedant, 5-lipoxygenase inhibitory, molluscicidal, muscle relaxant, neurotrophic, plant growth regulatory, superoxide release inhibitory, thromboxane synthase inhibitory, and vasopressin antagonist activities. Several reviews dealing with biologically active compounds from liverworts have been published more recently.69-79
2.1. Antibacterial, Antifungal and Antiviral Activity
Six bis(bibenzyls), riccardin C (3), riccardin F (5), isoriccardin C (8), marchantin H (20), neomarchantin A (32), and pakyonol (38) isolated from Plagiochasma intermedium possessed weak in vitro antifungal activity against the fluconazole-sensitive and resistant strains of Candida albicans at MIC’s ranging from 32 to >512µg/cm3. When riccardin C (3) was combined to fluconazole, the synergistic or additive activity of 3 caused the MICs of fluconazole to be reduced from 256 to <8µg/cm3 against three resistant strains of C. albicans.21
Guo and associates80 reported that riccardin D (4) indicated antifungal activity against the fluconazole-resistant Candida albicans strains, QL-14, QL-28, SDEY-24R and SDEY-09R at MIC 16, 32, 16 and 16µg/cm3, respectively. This activity is more potent than fluconazole itself. When riccardin D (4) was mixed with fluconazole, the antifungal activity dramatically increased to 0.3125-0.375µg/cm3.
Wu and colleagues81 demonstrated that the antifungal activity of riccardin D (4) (= plagiochin E) might be attributed to its inhibitory effect on cell wall chitin synthesis in Candida albicans.
Riccardin D (4) exerts its antifungal activity through mitochondrial dysfunction-induced reactive oxygen species accumulation in Candida albicans.82 Riccardin D (4) also induced apoptosis in Candida albicans through activating the metacaspase.83
The known bis-bibenzyls, isoplagiochin D (47), 6',8'-dichloroisoplagiochin C (= bazzanin B) (57), and 6'-chloroisoplagiochuin D (= bazzanin S) (66), isolated from Bazzania trilobata, showed discernible antifungal activity in a micro titer plate test against Pyricularia oryzae and Septoria tritici. Compound 47 also demonstrated inhibitory activity against Botrytis cinerea. Free hydroxyl groups on the benzene rings of bis-bibenzyls play an important role in mediating inhibitory activity against fungi such as Cladosporium cucumerinum.53
Marchantin C (16), neomarchantins A (32) and B (33) isolated from Schistochila glaucescens showed antimicrobial activity against the Gram-positive bacterium, Bacillus subtilis, with MIC 2, 1.5, and 2µg/cm3, and were also active against the dermatophytic fungus Trichophyton mentagrophytes, with MIC values of 0.5, 0.5, and 1µg/cm3. 37
The H1N1 and H5N1 influenza A virus caused pandemics throughout the world in 2009. Influenza A possesses an endonuclease within its RNA polymerase which comprises PA, PB1, and PB2 subunits. In order to obtain potential new anti-influenza compounds, 33 different types of phytochemicals using a PA endonuclease inhibition assay in vitro and an antiinfluenza virus assay were investigated.84 Among them, marchantins A (14), B (15), and E (18), plagiochin A (41), and perrottetin F (91) inhibited influenza PA endonuclease activity at a concentration of 10µM. This is the first evidence that the phytochemicals derived from liverworts can inhibit the influenza A endonuclease.
2.2. Antioxidant Activity
Marchantin A (14) also showed free radical scavenging activity at EC50 20µg/cm3. 85 Marchantin H (20) showed non-enzymatic iron-induced lipid peroxidation in rat brain homogenates at IC50 0.51µM.86 It is stronger than desferrioxamine or other classical antioxidants. Compound (20) suppressed NADPH-dependent microsomal lipid peroxidation at IC50 0.32µM without affecting microsomal electron transport of NADPH-cytochrome P450 reductase. It also inhibited copper-catalyzed oxidation of human low-density lipoprotein. Hsiao and colleagues concluded that marchantin H (20) is a potentially effective and versatile antioxidant, and can be used as a chaperone protecting the biomacrocyclic molecule against peroxidative damage.86
2.3. Antiplatelet Activity
Marchantiaquinone (26), obtained from Reboulia hemisphaerica, showed antiplatelet activity at a concentration level of 100µg/cm3.38
2.4. Antithrombin Activity
Perrottetin E (90) showed inhibitory activity against thrombin (IC50 18µM), which is associated with blood coagulation.9
2.5. Cytotoxic and Apoptotic Activity
Riccardin D (= plagiochin E) (4) indicated proliferation inhibitory activity on human glioma A172 cell and induction of apoptosis at 16μM. Compound 4 also possesses strong effects in reversing P-glycoprotein-mediated multidrug resistance. Thus, compound 4 is a potential candidate for reversing drug resistance in cancer chemotherapy.87
The antitumor activity of riccardin F (5) and pakyonol (38) were evaluated in vitro by employing K562 and K562/1702 cells, the well-known Adriamycin-induced multidrug resistance tumor cell lines over-expressing P-glycoprotein. In the presence of riccardin F (5) or pakyonol (38) ID50 of ADR against K562/1702 cells decreased by 2.51- and 4.78-fold, respectively.88
Marchantin C (16), neomarchantins A (32) and B (33), and a mixture of sesquiterpene/bis-bibenzyl dimers, GBB A (83) and GBB B (84) from Schistochila glaucescens showed growth inhibitory effects for the P-388 cell line, with IC50 values of 8.5, 18, 7.6, and 10.3µg/cm3, respectively.37
Marchantins A (14), B (33), D (15), perrottetin F (91), and paleatin B (95) showed DNA polymerase β inhibitory (IC50 range 14.4-97.5µM), cytotoxicity against KB cells (IC50 range 3.7-20µM), and anti-HIV-1 activity (IC50 range 5.3-23.7µg/cm3).5,55 Marchantin A (14) induced cell growth inhibition in human MCF-7 breast cancer cells at IC50 4.0µg/cm3. Fluorescence microscopic and a Western blot analysis indicated that compound 14 actively induced apoptosis of MCF cells through a caspase dependent pathway. The phenolic hydroxyl groups at C1’ and C6’ are responsible for inducing cytotoxic and antioxidant activity. Thus, marchantin A (14) is also a potential candidate drug for chemotherapy.85
Marchantin C (16) influences the migration and invasion of brain cancer cells and is capable to inhibit angiogenesis at low concentrations. The same compound appears to affect matrix metalloproteinase (MMP)-2 activity via the MAPK pathway.89
2.6. Farnesoid X-receptor (FXR) activation
The farnesoid X-receptor (FXR), a member of the nuclear-receptor super-family, controls the expression of critical genes in bile acid and cholesterol homeostasis. Marchantins A (14) and E (18) activated FXR in this receptors assay at a high potency level, comparable to that of the most potent endogenous bile acid, chenodeoxycholic acid.30
2.7. α-Glucosidase Inhibitory Activity
Inhibitory activity of α-glucosidase is associated with anti-obesity and anti-diabetes. Among bis-bibenzyls found in liverworts, only marchantin C (16) thus far has shown inhibitory activity against α-glucosidase (52.2% at 1mM). This activity is lower than that of the 1-deoxynojirimycin (100% at 0.4mM). This is the first report on the α-glucosidase inhibitory activity of macrocyclic bis-bibenzyls.35
2.8. Liver X Receptor Alpha (LXRα) Agonist Activity
Riccardin C (3) isolated from Blasia pusila possesses a nuclear receptor LXRα selective agonist activity. Thus, riccardin C (3) and its seven O-methyl derivatives, including riccardins A (1) and F (5), were synthesized. According to a preliminary structure-activity relationship study of these seven O-methylated riccardins, the three phenolic hydroxyl groups of riccardin C were determined as being indispensable for binding to the LXRα receptor.90
2.9. Tubulin Polymerization Inhibition
Marchantin C (16) strongly inhibited the growth of human cervical tumor engrafts in nude mouse and decreased the quantity of microtubules in a time- and dose-dependent mannterin G2/M phase in human glioma tumor cells and HeLa (human cervical adenocarcinoma cell line) cells at 8-16μM. Marchantin C (16) decreased the polymerization rate of gross tubulin, similar to microtubule depolymerizer vincristine at 8-24μM. These results indicated that marchantin C (16) plays the same role in microtubule depolymerization and apoptotic effects and subsequent anti-tumoral activity in vivo. Marchantin C (16) is a novel microtubule inhibitor that induces mitotic arrest of tumor cells and suppresses tumor cell growth. The structure of marchantin C is distinct from classical microtubule inhibitors like colchicine, vinblastine, and vincristine, and paclitaxel. However, this macrocyclic bis bibenzyl is a potential antitumor agent by inhibiting microtubule polymerization.91,92
Marchantin C (16) and its dimethyl ether, 7,8-dehydro-marchantin C and its dimethyl ether were synthesized and their possible modulatory effect on p-glycoprotein in VCR-resistant KB/VCR cells was investigated93. The results indicated that 16 was the most potent inhibitor of cell proliferation in both KB and KB/VCR ells among these four synthetic compounds, while the three derivatives of 16 have little anti-proliferative activity. Potent apoptosis in KB/VCR cells was induced by treatment with 16µM of dimethyl ether of marchantin C (16) and 0.2µM VCR for 48h.93
Isoplagiochins A (44) and B (45) isolated from Plagiochila fruticosa inhibited the polymerization of tubulin with IC50 values of 50 and 25µM. The dihydro derivatives of both 44 and 45 were found to be inactive (IC50 >100µM), and, when compared with the parent compounds, indicated that a restricted biaryl ring system is favorable for tubulin binding. A Monte Carlo search showed that the presence of two aromatic rings connected by a two-carbon bridge with a double bond may serve to maintain the backbone conformation.46
2.10. Calcium inhibitory activity
Conformational analysis of riccardin A (1) and marchantin A (14) was carried out by systematic unbounded multiple minimum search (SUMM). Mobility of the macrocyclic rings of both compounds was analyzed by a variable temperature (20-100 oC) 1H NMR study. The results indicated the restricted mobility of the macrocyclic ring of riccardin A (1) and gave further evidence for its more rigid nature in comparison to marchantin A (14). Comparing the calcium inhibitory activity of 1 and 14 (ID50: 20 and 1.85µg/cm3) implied the reduced affinity of 1 to calcium ions, which is identical to the calculated differences in steric and electrostatic properties of 1 and 14. Thus introduction of the biphenyl linkage to the macrocyclic ring decreased its mobility and this fact might be responsible for the reduction of biological activity.94
3. BIOSYNTHESIS OF BIS-BIBENZYLS
The naturally occurring bis-bibenzyls are categorized into four structure types, which are made up of macrocyclic rings linked via one biphenyl ether C-O bond, two biphenyl ether C-O bonds, one biaryl C-C bond, and two biphenyl bonds.
Asakawa and Matsuda95 proposed that cyclic bis-bibenzyls, such as riccardin C (3) and marchantin A (14), might be biosynthesized from bibenzyls that correspond chemically to dihydrostilbenes. This assumption was proved by feeding experiments of radioactive and 13C-labelled precursors, like L-[U-14C] phenylalanine, [U-14C] dihydro-p-coumaric acid, [2-13C] acetate, and L-[13COOH] phenylalanine, as shown in Scheme 1.96 The aqueous precursor solutions were applied to 0.5 cm2 samples of aseptic thallus tissue of Marchantia polymorpha and incubated for 24 h. The A- and B-rings of the marchantin molecule are derived from the benzene ring of L-phenylalanine via trans-cinnamic acid and p-coumaric acid. Application of the 13C-labelled precursor with subsequent 13C NMR spectroscopy established that dihydrocoumaric acid is an intermediate in marchantin biosynthesis. Enzymatically hydrogenated dihydrocoumaric acid from coumaric acid condenses with three molecules of malonyl-Co A to form prelunularic acid (105). The latter is aromatized to yield lunularic acid (106) and possibly lunularin (104), which is followed by condensation of lunularin or lunularic acid to afford marchantin A (14). The mechanism of this final coupling step is still unknown.
4. TOTAL SYNTHESIS OF BIS-BIBENZYLS
Since cyclic bis-bibenzyls found in liverworts possess unusual structures and various interesting biological activities, several organic chemists have focused on their total synthesis. Gottsegen and colleagues97 synthesized riccardins A-C (1-3) using a combination of Ullmann, Wittig, and Wurtz reactions and Ni (0)-assisted intermolecular coupling reaction. The total synthesis of riccardin C (3) was also accomplished by Harrowven and associates98-100 using is vanillin as the starting material in the course of the total synthesis of cavicularin (67a) as shown in Scheme 2. Cavicularin (67a) isolated from the liverwort, Cavicularia densa is a structurally interesting phenanthrene-bibenzyl derivative which has a highly strained structure and the benzene ring A was twisted. Although the structure has no chiral centre, it shows positive specific optical rotation, [α]D +168.2 and CD Cotton effects [312 nm (Δε +4.6), 280 (+2.6), 255 (-2.6) and 208 (+24.6). This phenomenon implicated that 67a possessed both planar and axial chirality.101
The total synthesis of riccardin C (3), and its seven O-methylated derivatives were achieved by Hioki and coworkers90 via intramolecular Suzuki-Miyaura coupling to construct the necessary 18-membered biaryl linkage. Riccardin C (3) has been also synthesized by Takiguchi and coworkers102 using an intramolecular SN reaction of α-sulfinylfluorobenzene (A-ring) by internal phenolate (C-ring), providing the key 18-membered ring closure in high yield.
Plagiochin E (4a) was isolated from the Chinese Marchantia polymorpha23 and Asterella angusta12 through bioassay-guided fractionation of the antifungal constituents. A short and efficient total synthesis of plagiochin E (4a) was accomplished by Speicher and associates.103 However, its spectroscopic data were not the same as those of the natural product 4a, but identical with those of riccardin D (4) which was isolated from Plagiochila fruticosa.3 Thus, the structure of 4a should be revised as riccardin D (4). Riccardin G (6), the methyl ether of 4, has been found in Marchantia chenopoda.3
Xi and associates93 accomplished the total synthesis of marchantin C (16) by 12 steps from 2-hydroxy-3-methoxybenzaldehyde with the sufficient yield (23%). Total synthesis of marchantiaquinone (26) was accomplished by López and associates104 by macro-cyclization from a dichloride precursor using an active nickel complex under high dilution conditions, giving marchantin M (24) trimethyl ether, followed by demethylation with boron tribromide and oxidation using silver oxide.
Nogradi and associates accomplished the total synthesis of pakyonol (38) by Ullmann coupling of 3-benzoyloxy-4-(3-formylphenoxy)benzoic acid benzyl ester with Wittig and modified Wittig reactions.105
Fukuyama and associates106 accomplished the total synthesis of plagiochin D (40) using m-anisaldehyde as the starting material, followed by a Wardworth-Emmons condensation reaction, hydrogenation, and a Stille-Kelly reaction using hexamethylditin and tetrakis(triphenylphosphine)palladium, to yield plagiochin D (40), after removal of the MOM group by HBr in methanol.
The total synthesis of isoplagiochin A (44) was achieved by Gerencsér and colleagues107 in 23 steps by coupling methyl 3-(2-methoxy-5-formylphenyl)-4-methoxybenzoate with methyl (2-(hydroxymethyl)-4- methoxyphenoxy)benzoate, in an overall yield of 15%.
Eicher and associates established the total synthesis of isoplagiochin C (46).108 The total synthesis of isoplagiochin D (47), a highly strained macrocyclic bis-bibenzyl with two biaryl units isolated from Plagiochila fruticosa, was achieved by Sumy and colleagues in 1.6% overall yield and in 11 steps, by construction of tetramethoxyisoplagiochin D by a palladium(0)-catalyzed Suzuki-Miyaura cross-coupling reaction, followed by demethylation with boron tribromide in methylene chloride.109 The tetramethyl ether of isoplagiochin D (47) as well as related compounds possessing the (Z)- and (E)-alkenes with more rigid two-carbon biaryl bridges, were synthesized using Sonogashira and McMurry protocols.103
Speicher and coworkers accomplished the total synthesis of 12-chloroisopalgiochin D (52) isolated from Plagiochila species, and 6'-chloroisopalgiochin C (= bazzanin A) (56) and 6,12'-dichloroisoplagiochin D (= bazzanin J) (65) from Bazzania trilobata, by construction of the biaryl moiety using regioselective Suzuki protocols and coupling to acyclic bibenzyl and cyclic bibenzyls by Wittig and MacMurry procedures, followed by hydrogenation and deprotection.110
CONCLUSIONS
In conclusion, the Marchantiophyta (liverworts) are rich sources of cyclic and acyclic bis-bibenzyls among which marchantin A (14) shows interesting biological activity, such as antioxidant, antifungal and antimicrobial activity, cytotoxicity, antiinfluenza, farnesoid X-receptor (FXR) activation, calcium and NO production inhibitory activity. Riccardin C (3), and isoplagiochins A (44) and B (45) are therapeutically interesting compounds since they show antiobesity and inhibition of the polymerization of tubulin, respectively. Some cyclic bis-bibenzyls have optical activity and the structures of two conformers have been established by CD spectral analysis. An acyclic bis-bibenzyl, perrottetin H (92a) and a cyclic bis-bibenzyl, riccardin C (3) have been isolated from the fern, Hymenophyllum barbata68 and the higher plant, Primula species, respectively.67 The presence of such bis-bibenzyls in different plants from the Marchantiophyta is quite interesting phenomenon to consider the plant phylogeny.3,111
ACKNOWLEDGEMENTS
This work was supported in part by a Grant-in-Aid for the Senryaku Research (for A. L.) from the Ministry of Education, Culture, Sports, Science and Technology.
References
1. H. Ding, ‘Zhong guo Yao Yun Bao zi Zhi Wu’, Kexue Jishu Chuban She, Shanghai, 1982.
2. Y. Asakawa, ‘Progress in the Chemistry of Organic Natural Products’, Vol. 42, ed. by W. Herz, H. Grisebach, and G. W. Kirby, Springer, Vienna, 1982, pp. 1-285. CrossRef
3. Y. Asakawa, ‘Progress in the Chemistry of Organic Natural Products’, Vol. 65, ed. by W. Herz, W. B. Kirby, R. E. Moore, W. Steglich, and Ch. Tamm, Springer, Vienna, 1995, pp. 1-618. CrossRef
4. Y. Asakawa, M. Toyota, F. Nagashima, T. Hashimoto, and L. E. Hassane, Heterocycles, 2001, 54, 1057. CrossRef
5. Y. Asakawa, A. Ludwiczuk, F. Nagashima, M. Toyota, T. Hashimoto, M. Tori, Y. Fukuyama, and L. Harinantenaina, Heterocycles, 2009, 77, 99. CrossRef
6. G. M. Keseru and M. Nogradi, Med. Chem., 1995, 3, 1511. CrossRef
7. G. M. Keseru and M. Nogradi, Nat. Prod. Rep., 1995, 12, 69. CrossRef
8. Y. Asakawa, M. Toyota, M. Tori, and T. Hashimoto, Spectroscopy, 2000, 14, 149. CrossRef
9. F. Nagashima, S. Momosaki, Y. Watanabe, M. Toyota, S. Huneck, and Y. Asakawa, Phytochemistry, 1996, 41, 207. CrossRef
10. T. Yoshida, M. Toyota, and Y. Asakawa, J. Nat. Prod., 1997, 60, 145. CrossRef
11. M. S. Buchanan, M. Toyota, T. Yoshida, and Y. Asakawa, J. Hattori Bot. Lab., 1997, 83, 265.
12. J. Qu, C. Xie, H. Guo, W. Yo, and H. Lou, Phytochemistry, 2007, 68, 1767. CrossRef
13. Y. Asakawa, T. Hashimoto, K. Akazawa, and S. Huneck, J. Hattori Bot. Lab., 1997, 81, 243.
14. T. Yoshida, T. Hashimoto, S. Takaoka, Y. Kan, M. Tori, and Y. Asakawa, Tetrahedron, 1996, 52, 14487. CrossRef
15. M. Toyota, T. Yoshida, J. Matsunami, and Y. Asakawa, Phytochemistry, 1997, 44, 293. CrossRef
16. Z.-Q. Lu, P.-H. Fan, M. Ji, and H.-X. Lou, J. Asian Nat. Prod. Res., 2006, 8, 187. CrossRef
17. C.-L. Wu and H.-R. Lin, Phytochemistry, 1997, 44, 101. CrossRef
18. L. Harinantenaina and Y. Asakawa, Chem. Pharm. Bull., 2004, 52, 1382. CrossRef
19. T. Hashimoto, H. Irita, and Y. Asakawa, J. Hattori Bot. Lab., 1998, 85, 239.
20. A. Bardón, N. Kamiya, M. Toyota, S. Takaoka, and Y. Asakawa, Phytochemistry, 1999, 52, 1323. CrossRef
21. C.-F. Xie, J.-B. Qu, X.-Z. Wu, N. Liu, M. Ji, and H.-X. Lou, Nat. Prod. Res., 2010, 24, 515. CrossRef
22. H. Anton, R. Schoeneborn, and R. Mues, Phytochemistry, 1999, 52, 1639. CrossRef
23. C. Niu, J.-B. Qu, and H.-X. Lou, Chem. Biodivers., 2006, 3, 34. CrossRef
24. E. H. Lahlou, T. Hashimoto, and Y. Asakawa, J. Hattori Bot. Lab., 2000, 88, 271.
25. S. Valcic, J. Zapp, and H. Becker, Phytochemistry, 1997, 44, 89. CrossRef
26. M. Tori, M. Aoki, and Y. Asakawa, Phytochemistry, 1994, 36, 73. CrossRef
27. M. L. So, W. H. Chan, P. F. Xia, and Y. Cui, Nat. Prod. Lett., 2002, 16, 167. CrossRef
28. L. Fang, H.-F. Guo, and H.-X. Lou, Helv. Chim. Acta, 2007, 90, 748. CrossRef
29. A. Hosoda, Master Thesis, Tokushima Bunri University, Japan, 2003.
30. T. Suzuki, N. Tamehiro, Y. Sato, T. Kobayashi, A. Ishii-Watanabe, Y. Shinozaki, T. Nishimaki-Mogami, T. Hashimoto, Y. Asakawa, K. Inoue, Y. Ohno, T. Yamaguchi, and T. Kawanishi, J. Pharmacol. Sci., 2008, 107, 285. CrossRef
31. M. Toyota and Y. Asakawa, J. Hattori Bot. Lab., 1999, 86, 161.
32. M. Konoshima, Master Thesis, Tokushima Bunri University Tokushima, Japan, 1998.
33. A. Bardón, N. Kamiya, M. Toyota, and Y. Asakawa, Phytochemistry, 1999, 51, 281. CrossRef
34. Y. Asakawa, M. Toyota, E. Nakaishi, and Y. Tada, J. Hattori Bot. Lab., 1996, 80, 271.
35. L. Harinantenaina, S. Kida, and Y. Asakawa, ARKIVOC, 2007, ii, 22.
36. F. Nagashima, H. Izumo, S. Takaoka, M. Tori, and Y. Asakawa, Phytochemistry, 1994, 37, 433. CrossRef
37. J. M. Scher, E. J. Burgess, S. D. Lorimer, and N. B. Perry, Tetrahedron, 2002, 58, 7875. CrossRef
38. H.-C. Wei, S.-J. Ma, and C.-L. Wu, Phytochemistry, 1995, 39, 91. CrossRef
39. M. Furusawa, T. Hashimoto, Y. Noma, and Y. Asakawa, Chem. Pharm. Bull., 2006, 54, 996. CrossRef
40. M. Toyota, M. Konoshima, and Y. Asakawa, Phytochemistry, 1999, 52, 105. CrossRef
41. F. Nagashima, T. Sekiguchi, S. Takaoka, and Y. Asakawa, Chem. Pharm. Bull., 2004, 52, 556. CrossRef
42. F.-Q. Wang and H.-X. Lou, Acta Pharm. Sin., 2000, 35, 587.
43. K. Nabeta, S. Ohkubo, R. Hozumi, and K. Katoh, Phytochemistry, 1998, 49, 1941. CrossRef
44. M. Sadamori, Master Thesis, Tokushima Bunri University Tokushima, Japan, 2009.
45. J. Heinrichs, H. Anton, S. R. Gradstein, and R. Mues, Plant Syst. Evol., 2000, 220, 115. CrossRef
46. H. Morita, Y. Tomizawa, T. Tsuchiya, Y. Hirasawa, T. Hashimoto, and Y. Asakawa, Bioorg. Med. Chem. Lett., 2009, 19, 493. CrossRef
47. T. Hashimoto, S. Kanayama, Y. Kan, M. Tori, and Y. Asakawa, Chem. Lett., 1996, 25, 741. CrossRef
48. H. Anton, L. Kraut, R. Mues, and M. I. Moralez, Phytochemistry, 1997, 46, 1069. CrossRef
49. T. Hashimoto, H. Irita, S. Takaoka, M. Tanaka, and Y. Asakawa, Tetrahedron, 2000, 56, 3153. CrossRef
50. T. Hashimoto, M. Toyota, H. Irita, and Y. Asakawa, J. Hattori Bot. Lab., 2000, 89, 267.
51. H. Irita, T. Hashimoto, Y. Fukuyama, and Y. Asakawa, Phytochemistry, 2000, 55, 247. CrossRef
52. J. M. Scher, J. Zapp, A. Schmidt, and H. Becker, Phytochemistry, 2003, 64, 791. CrossRef
53. J. M. Scher, J.-B. Speakman, J. Zapp, and H. Becker, Phytochemistry, 2004, 65, 2583. CrossRef
54. S.-H. Shy and C.-L. Wu, J. Asian Nat. Prod. Res., 2006, 8, 723. CrossRef
55. Y. Asakawa, M. Toyota, F. Nagashima, and T. Hashimoto, Nat. Prod. Commun., 2008, 3, 289.
56. U. Martini, J. Zapp, and H. Becker, Phytochemistry, 1998, 47, 89. CrossRef
57. T. Hashimoto, H. Ikeda, S. Takaoka, M. Tanaka, and Y. Asakawa, Phytochemistry, 1999, 52, 501. CrossRef
58. S. Kunz and H. Becker, Phytochemistry, 1994, 36, 675. CrossRef
59. F. Cullmann, H. Becker, E. Pandolfi, E. Roeckner, and T. Eicher, Phytochemistry, 1997, 45, 1235. CrossRef
60. L. Kraut, R. Mues, and H. D. Zinsmeister, Phytochemistry, 1997, 45, 1249. CrossRef
61. M. Flegel, K.-P. Adam, and H. Becker, Phytochemistry, 1999, 52, 1633. CrossRef
62. F. Nagashima, A. Tamada, N. Fujii, and Y. Asakawa, Phytochemistry, 1997, 46, 1203. CrossRef
63. I. Omatsu, Master Thesis, Tokushima Bunri University Tokushima, Japan, 2004.
64. F. Cullmann and H. Becker, J. Hattori Bot. Lab., 1998, 84, 285.
65. F. Cullmann and H. Becker, Z. Naturforsch., 1999, 54c, 147.
66. U. M. Hertewich, J. Zapp, and H. Becker, Phytochemistry, 2003, 63, 227. CrossRef
67. Y. S. Kosenkova, M. P. Polovinka, N. I. Komarova, D. V. Korchagina, N. Y. Kurochkina, V. A. Cheremushkina, and N. F. Salakhutdinov, Chem. Nat. Compd., 2007, 43, 712. CrossRef
68. Y. Oiso, M. Toyota, and Y. Asakawa, Chem. Pharm. Bull., 1999, 47, 297. CrossRef
69. Y. Asakawa, J. Hattori Bot. Lab., 1998, 84, 91.
70. Y. Asakawa, ‘Phytochemicals in Human Health Protection, Nutrition, and Plant Defense’, ed. by J. Romeo, Kluwer Academic/Plenum Publishers, New York, 1999, pp. 319-342. CrossRef
71. Y. Asakawa, Chenia, 2007, 9, 73.
72. Y. Asakawa, Curr. Pharm. Design, 2008, 14, 3067. CrossRef
73. Y. Asakawa, Science and Culture, 2008, 74, 31.
74. Y. Asakawa, ‘Bryology in the New Millenum’, ed. by H. Mohamed, B. B. Baki, A. Nasrulhaq-Boyce, P. K. Y. Lee, Kuala Lumpur, University of Malaya, 2008, pp 367-394.
75. Y. Asakawa, Nat. Prod. Commun., 2008, 3, 77.
76. Y. Asakawa and A. Ludwiczuk, Medicinal Plants in Poland and in the World (Rośliny Lecznicze w Polsce i na Świecie), 2008, 2, 33.
77. Y. Asakawa, A. Ludwiczuk, and F. Nagashima, Phytochemistry, 2012, http://dx.doi.org/10.1016/ j.phytochem.2012.04.0212.
78. F. Nagashima and Y. Asakawa, ‘Recent Research Developments in Phytochemistry’, Vol. 2, Part II, ed. by S. G. Pandalay, Research Signpost, India, 1998, pp 327-382.
79. L. Harinantenaina and Y. Asakawa, Nat. Prod. Commun., 2007, 2, 701.
80. X. L. Guo, P. Leng, Y. Yeng, L. G. Yu, and H. Lou, J. Appl. Microbiol., 2008, 104, 831. CrossRef
81. X.-Z. Wu, A.-X. Cheng, L.-M. Sun, and H.-X. Lou, Acta Pharmacol. Sin., 2008, 29, 1478. CrossRef
82. X.-Z. Wu, A.-X. Cheng, L.-M. Sun, S.-J. Sun, and H.-X. Lou, Biochim. Biophys. Acta, 2009, 1790, 770.
83. X.-Z. Wu, W.-Q. Chang, A.-X. Cheng, L.-M. Sun, and H.-X. Lou, Biochim. Biophys. Acta, 2010, 1800, 439.
84. Y. Iwai, K. Murakami, Y. Gomi, T. Hashimoto, Y. Asakawa, Y. Okuno, T. Ishikawa, D. Hatakeyama, N. Echigo, and T. Kuzuhara, PLoS ONE, 2011, 6, e19825. CrossRef
85. W.-J. Huang, C.-L. Wu, C.-W. Lin, L.-L. Chi, P.-Y. Chen, C.-J. Chiu, C.-Y. Huang, and C.-N. Chen, Cancer Lett., 2010, 291, 108. CrossRef
86. G. Hsiao, C.-M. Teng, C.-L. Wu, and F.-N. Ko, Arch. Biochem. Biophys., 1996, 334, 18. CrossRef
87. Y.-Q. Shi, X.-J. Qu, Y.-X. Liao, C.-F. Xie, Y.-N. Cheng, S. Li, and H. X. Lou, Eur. J. Pharmacol., 2008, 584, 66. CrossRef
88. M. Ji, Y. Shi, and H. Lou, Biosci. Trends, 2011, 5, 192. CrossRef
89. J. Shen, G. Li, Q. Liu, O. He, J. Gu, Y. Shi, and H. Lou, Cancer Biol. Ther., 2010, 9, 33. CrossRef
90. H. Hioki, N. Shima, K. Kawaguchi, K. Harada, M. Kubo, T. Esumi, T. Nishimaki-Mogami, J.-I. Sawada, T. Hashimoto, Y. Asakawa, and Y. Fukuyama, Bioorg. Med. Chem. Lett., 2009, 19, 738. CrossRef
91. Y.-Q. Shi, Y.-X. Liao, X.-J. Qu, H.-Q. Yuan, S. Li, J.-B. Qu, and H. X. Lou, Cancer Lett., 2008, 262, 173. CrossRef
92. Y.-Q. Shi, C.-J. Zhu, H.-Q. Yuan, B.-Q. Li, J. Gao, X.-J. Qu, B. Sun, Y.-N. Cheng, S. Li, X. Li, and H.-X. Lou, Cancer Lett., 2009, 276, 160. CrossRef
93. G.-M. Xi, B. Sun, H.-H. Jiang, F. Kong, H.-Q. Yuean, and H.-X. Lou, Bioorg. Med. Chem., 2010, 18, 6725. CrossRef
94. G. M. Keseru and M. Nogradi, J. Mol. Recogn., 1996, 9, 133. CrossRef
95. Y. Asakawa and R. Matsuda, Phytochemistry, 1982, 21, 2143. CrossRef
96. S. Friederich, U. H. Maier, B. Deus-Meumann, Y. Asakawa, and M. H. Zenk, Phytochemistry, 1999, 50, 589. CrossRef
97. A. Gottsegen, M. Nógrádi, B. Vermes, M. Kajtár-Peredy, and E. Bihátsi-Karsai, J. Chem. Soc., Perkin Trans.1, 1990, 315. CrossRef
98. D. Harrowven, T. Woodcock, and P. D. Howes, Angew. Chem. Int. Ed., 2005, 44, 3899. CrossRef
99. D. Harrowven and S. Kostiuk, Nat. Prod. Rep., 2012, 29, 223. CrossRef
100. S. L. Kostiuk, T. Woodcock, L. F. Daidin, P. D. Howes, and D. C. Harrowven, Chem. Eur. J., 2011, 17, 10905. CrossRef
101. M. Toyota, T. Yoshida, Y. Kan, S. Takaoka, and Y. Asakawa, Tetrahedron Lett., 1996, 37, 4745. CrossRef
102. H. Takiguchi, K. Ohmori, and K. Suzuki, Chem. Lett., 2011, 1069. CrossRef
103. A. Speicher, T. Backes, K. Hesidens, and J. Kolz, Beilstein J. Org. Chem., 2009, 5, 71. CrossRef
104. G. V. Lopez, E. M. Pandolfi, and G. A. Seoane, Synthesis, 2000, 1403. CrossRef
105. M. Nógrádi, B. Vermes, M. Kajtár-Peredy, and V. P. Novikov, Liebigs. Ann. Chem., 1990, 299. CrossRef
106. Y. Fukuyama, H. Yaso, K. Nakamura, and M. Kodama, Tetrahedron Lett., 1999, 40, 105. CrossRef
107. J. Gerencser, G. M. Keseru, I. Macsari, M. Nogradi, M. Kajtar-Peredy, and A. Szollosy, J. Org. Chem., 1997, 62, 3666. CrossRef
108. T. Eicher, S. Fey, W. Puhl, E. Büchel, and A. Speicher, Eur. J. Org. Chem., 1998, 877. CrossRef
109. T. Esumi, M. Wada, E. Mizushima, N. Sato, M. Kodama, Y. Asakawa, and Y. Fukuyama, Tetrahedron Lett., 2004, 45, 6941. CrossRef
110. A. Speicher, J. Kolz, and R. P. Sambanje, Synthesis, 2002, 2503. CrossRef
111. Y. Asakawa, A. Ludwiczuk, and F. Nagashima, ‘Progress in the Chemistry of Organic Natural Products’, Vol. 95, ed. by D. A. Kinghorn, H. Falk, and J. Kobayashi, Springer, Vienna, 2012, pp. 1-765.