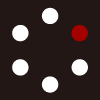
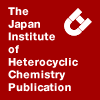
HETEROCYCLES
An International Journal for Reviews and Communications in Heterocyclic ChemistryWeb Edition ISSN: 1881-0942
Published online by The Japan Institute of Heterocyclic Chemistry
e-Journal
Full Text HTML
Received, 1st July, 2012, Accepted, 20th July, 2012, Published online, 30th July, 2012.
DOI: 10.3987/COM-12-S(N)84
■ STEREOSELECTIVE SYNTHESIS OF 2-SUBSTITUTED 1-IODO-1,3-dIENES BY THE ADDITION OF MeMgSnBu3 TO THE CONJUGATED TERMINAL ENYNES; STEREOCONTROLLED SYNTHESIS OF (2E,4Z,6E)-DEHYDRODENDROLASIN
Reiko Kawahama and Jun'ichi Uenishi*
Kyoto Pharmaceutical University, Misasagi, Yamashina-ku, Kyoto 607-8412, Japan
Abstract
Addition of MeMgSnBu3 to the conjugated enynes 1 and 5 were thoroughly studied. The addition reactions were proceeded stereoselectively at –20 °C, but a thermal isomerization occurred when the reaction was carried out at 0 °C. (2E,4Z,6E)-Dehydrodendrolasin 14 was synthesized using the isomerized 2-methyl-1-iodo-1,3-diene product 17.INTRODUCTION
Stereoselective construction of conjugated polyene is a challenging task in organic synthesis. Particularly, Pd-catalyzed cross-coupling is an important and powerful carbon-carbon bond forming reaction for the synthesis of conjugated polyenes.1 Suzuki-Miyaura reaction catalyzed by Pd as illustrated in Scheme 1 is valuable for the synthesis of sensitive product because of its mild reaction conditions and high stereospecificity.2
Since Pd-catalyzed cross-coupling reactions for diene synthesis between alkenylmetal and alkenyl halide proceed with retention of stereochemistry, the preparation of stereochemically pure starting materials, namely, 2-substituted 1-haloalkene and/or 2-substituted 1-metalloalkene is very important to obtain the target diene stereoselectively. In this regard, when R is a simple alkyl group such as methyl and ethyl group, carbometallation reactions3 to the terminal alkynes developed by Negishi,4 Normant and Alexakis,5 and others6 are potentially useful and reliable. In these reactions, the addition of an R group provided from the corresponding organometallic reagents to terminal alkyne makes the 2-position of the intermediate alkene a positively charged carbon and 1-position a negatively charged carbon. On the other hand, Oshima and Nozaki reported the addition reaction of methylmagnesiumtributylstannane (MeMgSnBu3) generated from methyl Grignard reagent and tributylstannyllithium reagent to terminal alkynes in 1984.7 They have proposed that the reaction proceeds via 1,2-dimetalloalkene intermediate, 2-methylmagnesium-1-tributylstannyl alkene, as shown in Scheme 2.
In this case, both of the alkenyl carbons can be considered negatively charged in the intermediate after the addition. Therefore, a variety of electrophiles can be introduced not only on the 2-position but also on the terminal position of the alkene. In fact, Oshima and Nozaki have used methyl iodide, ethyl iodide, allyl bromide, and benzaldehyde as the electrophiles to synthesize a variety of 2-substituted 1-tributylstannylalkenes. 7 The terminal alkenyltributystannyl group is useful because it can be used as a good substrate for Stille coupling directly, and a substrate for many cross-coupling reactions after a transformation to iodoalkene by treating with iodine. We have applied this reaction to the conjugated terminal enynes and have synthesized several 2-susbtituted 1-iodo-1,3-dienes.8 Herein, we detail this addition reaction including unexpected isomerization reaction and its application to the stereoselective synthesis of furanoterpenoid, (2E,4Z,6E)-dehydrodendrolasin, which is a remaining geometric isomer not to be synthesized in the series of dehydrodendrolasin synthesis.
MeMgSnBu3 reacted with enyne 1 in the presence of CuCN (5 mol %) at –20 °C. The resulting 2-methylmagnesium-1-tributylstannyl alkene A was treated with MeI to give 2-methyl-1-tributylstannyl- 1,3-diene 2 (E = Me). However, the attempt to isolate this stannyldiene 2 (E = Me) as a pure form failed because of its sensitivity toward both aqueous work-up and chromatographic purification. In fact, the acidic work-up using acetic acid gave (3E)-2-methyl-6-phenyl-1,3-hexadiene in 93% yield, exclusively. Instead of isolating 2 (E = Me), dry hexane was added to the reaction mixture and the separated hexane layer containing dienylstannane 2 was transferred into a dry flask and it immediately was quenched with a methylene chloride solution of iodine. The desired (1E,3E)-1-iodo-2-methyl-6-phenyl-1,3-hexadiene 3a (E = Me) was isolated as a sole product in 91% yield. We have used a variety of electrophiles, for example, chloromethyl benzyl ether, oxirane, cyclopentenones, and cyclohexenone to give 2-substituted 1-iodo-1,3-dienes 3b, 3c, 3d, 3e, and 3f in 65%, 71%, 67%, 53%, and 61%, respectively.8 As shown in Scheme 3, all of these reactions proceeded stereoselectively as long as the reaction temperature was kept at –20 °C before the extraction with dry hexane.
However, when the above reaction was conducted at 0 °C, isomerization occurred. The result is shown in Scheme 4. Thus, when the reaction of 1 with MeI was carried out at 0 °C, 3a and 4 were obtained as an 86:14 mixture in 80% yield. When (Z)-enyne (5) was used, a 75:25 mixture of 6 and 7 was obtained in 78% yield. 9 Oshima et al. reported that depending on the R’ group of starting terminal alkyne, they have observed the formation of regioisomers, 2-tributylstannyl-1-alkenes, as a minor product. However, using conjugated enynes as a starting material, we did not obtain this type of regioisomer even at 0 °C. Structures of these isomers 4 and 7 were determined by comparison of 1H NMR and its nOe experiments. Therefore, 1H NMR of the major product 3a that possesses the conjugated (1E,3E)-diene system, indicates its J value of 15.5 Hz as a trans alkene and has no nOe between 2-methyl and the terminal proton. However, isomer 4 has a J value of 11.6 Hz and 14% of nOe was observed between the 2-methyl and the terminal proton, indicating its conjugated (1Z,3Z)-diene system. The same pattern was observed in 6 and 7. Therefore, major isomer 6 has a J value of 11.5 Hz as a cis alkene and no nOe between 2-methyl and the terminal proton, but isomer 7 has a J value of 15.6 Hz and 14% of nOe was observed.
Both isomerizations occurred in a stereospecific manner so that we assumed that some thermal isomerization occurred during the reactions. We have found that the isomerization took place when 2-methylmagnesium-1-tributylstannyldiene intermediate A was exposed above –20 °C. When A was stirred at room temperature for a long period of time, the isolated yield and the ratio of isomer 4 did not increase. Furthermore, when A was refluxed for 15 min, it became a complex mixture and neither 3a nor 4 were obtained. These results suggested that either the intermediate A is not stable above 0 °C or decomposition of the intermediates occurred during the isomerization reaction.
A plausible pathway of this isomerization is outlined in Scheme 5. A thermal 1,3-rearrangement of MeMg group on 2-methylmagnesium-1-tributylstannyldiene intermediate A gives an allene intermediate B. The C3-C4 σ-bond of B can rotate freely to form a conformational isomer C. In this conformer, MeMg group will be located farther away from the Bu3Sn group. We considered that the conformer C would exist more stable and favored than B because of electric and/or steric interactions between these two substituents. When MeMg group was rearranged from this position in C, it would give an isomerized 2-methylmagnesium-1-tributylstannyldiene intermediate D. We assumed that the isomer 4 was formed from this intermediate D after the reaction with methyl iodide and subsequent reaction with iodide. The stereospecific isomerization process in the same pathway also can explain the formation of the isomer 7 from 5.
Furanosesquiterpenoid, (2E,4Z,6E)-dehydrodendrolasin 8 was isolated from the marine sponge Ceratosoma brevicaudatum in 1988.10 We have reported the stereoselective total syntheses of its stereoisomers, (2E,4E,6E)- and (2Z,4E,6E)-dehydrodendrolasins, 9 and 10.11 These structures are shown in Figure 1. They consist of a conjugated triene and a furan ring connected by a methylene bridge, and are unstable against acids, heat, and light.
We planned to synthesize its (2Z)-isomer 10 using the addition of MeMgSnBu3 reagent to the corresponding (Z)-enyne 11. However, even though the reaction was carried out at –20 °C, desired (2Z,4E)-iodotriene 12 was obtained as a minor product. Therefore, we could not synthesize 10 using this addition reaction.12 However, its stereoisomer (2E,4Z)-iodotriene 13 was majorly produced a 9 : 1 ratio in 50% yield in this reaction (Scheme 6). Fortunately, the geometric structure of 13 is matched with that of a precursor for the synthesis of 8. After a separation of two isomers by flash column chromatography, the major isomer 13 was reacted with (E)-2-(3-methyl-1-butenyl)-1,3,2-benzodioxaborole under Suzuki coupling conditions using Ag2CO3 as an additive. The cross-coupling was proceeded at room temperature to afford (2E,4Z,6E)-dehydrodendrolasin 8 stereoselectively in 82% yield. The spectroscopic data of synthetic 8 was identical with those of natural 8.11
CONCLUSION
In conclusion, we have examined the addition of MeMgSnBu3 to the conjugated terminal (E)- and (Z)-enynes. The reaction of enynes 1 and 5 proceeded stereospecifically at –20 °C and subsequent reactions with electrophiles and iodine afforded the corresponding 1-iodo-2-substituted-6-phenyl- 1,3-hexadienes 3a-f and 6, respectively. We have found that a thermal isomerization occurs when the above reaction was carried out at 0 °C, and determined the structures of these isomers 4 and 7. We have accomplished the synthesis of (2E,4Z,6E)-dehydrodendrolasin 8 using the isomerized product 13.
EXPERIMENTAL
General. 1H NMR and 13C NMR spectra were measured on JEOL JNM-AL-300 (300 MHz and 75 MHz), spectrometer. Chemical shifts (δ) are reported in parts per million (ppm) relative to the resonance of the solvent or to tetramethylsilane (0.00 ppm) for 1H NMR spectra and for 13C NMR spectra. IR spectra were recorded on a JASCO FT/IR-410 spectrophotometer. UV spectra were measured by a JASCO V-530 spectrometer. Low and high-resolution mass spectra (LRMS and HRMS) were obtained on a JEOL JMS 303HF spectrometer using fast atom bombardment (FAB) ionization. Silica gel (230–400 mesh) was used for flash chromatography. Analytical thin-layer chromatography (TLC) was performed on glass pre-coated with silica gel (0.25 mm thickness). All moisture sensitive reactions were carried out under an argon atmosphere. THF was dried over sodium/benzophenone ketyl, and CH2Cl2 was dried over P2O5, and they were distilled prior to use.
General method of stannylmagnesation and iodination for enyne at –20 °C. To a suspension of dry SnCl2 (364 mg, 1.92 mmol) in anhydrous THF (3 mL) was added butyllithium (3.7 mL of 1.56 M solution in hexane, 5.76 mmol) dropwise during 10 min at –20 °C. After the mixture was stirred for 20 min at the same temperature, MeMgBr (2.0 mL of 0.95 M solution in THF, 1.92 mmol) was dropped to the reaction mixture for 5 min and the mixture was stirred for additional 15 min at the same temperature. Then anhydrous CuCN (5 mg, 0.06 mmol) and enyne (0.32 mmol) in THF were added to the mixture, successively. The mixture was further stirred at –20 °C for 15 min and then MeI (0.4 mL, 6.4 mmol) was added during 2min. After the mixture was stirred for 2 h, dry hexane (30 mL) was added and all the mixture was agitated vigorously. The dry hexane layer was transferred to an ice-cooled flask in which anhydrous K2CO3 (500 mg) was placed via cannula tube. A solution of iodine (2 g) dissolved in dry CH2Cl2 (40 mL) containing K2CO3 (1 g) was added to the reaction mixture slowly at 0 °C until the color becomes deep red. The mixture was diluted with hexane (30 mL) and the mixture was washed with aq. sodium thiosulfate (3 mL), water (3 mL X 2), and brine (3 mL). The extract was dried over MgSO4 and condensed under reduced pressure. The residue was purified by chromatography on Al2O3 eluted with hexane to give iodide 3.
3a: colorless oil; Rf = 0.31 (hexane); UV (EtOH) λ max nm (ε) 252 (27200, sh), 246 (29500), 205 (23700); 1H NMR (300 MHz, CDCl3) δ 1.93 (3H, s), 2.39 (2H, td, J = 7.7 and 7.0 Hz), 2.72 (2H, t, J = 7.7 Hz), 5.80 (1H, dt, J = 15.5 and 7.0 Hz), 6.17 (1H, d, J = 15.5 Hz), 6.20 (1H, s), 7.16-7.30 (5H, m); 13C NMR (75 MHz, CDCl3) δ 20.2, 34.5, 35.6, 81.6, 125.9, 128.3, 128.3, 130.4, 131.6, 141.5, 145.0; MS (EI) m/z (rel. intensity) 298 (M+, 50), 207 (70), 171 (base). HRMS (EI) m/z Calcd for C13H15I (M+) 298.0219. Found 298.0232.
3b: pale yellow oil; Rf = 0.39 (hexane); 1H NMR (300 MHz, CDCl3) δ 2.39 (2H, td, J = 8.2 and 6.0 Hz), 2.72 (2H, t, J = 8.2 Hz), 4.35 (2H, s), 4.50 (2H, s), 6.04 (1H, dt, J = 15.7 and 6.0 Hz), 6.12 (1H, d, J = 15.7 Hz), 6.46 (1H, s), 7.15-7.36 (10H, m); 13C NMR (75 MHz, CDCl3) δ 34.8, 35.4, 71.1, 72.0, 83.9, 125.9, 127.7, 128.0, 128.3, 128.4, 128.4, 129.2, 132.0, 138.1, 141.5, 144.8; MS (FAB) m/z 405 (M++H).
3c: pale yellow oil; Rf = 0.28 (20% EtOAc in hexane); IR (neat) 3360 cm-1; 1H NMR (300 MHz, CDCl3) δ 2.39 (2H, td, J = 7.7 and 7.0 Hz), 2.70 (2H, t, J = 7.1 Hz), 2.72 (2H, t, J = 7.7 Hz), 3.69 (2H, t, J = 7.1 Hz), 3.70 (1H, br s), 5.86 (1H, dt, J = 15.8 and 7.0 Hz), 6.09 (1H, d, J = 15.8 Hz), 6.31 (1H, s), 7.15-7.30 (5H, m); 13C NMR (75 MHz, CDCl3) δ 34.6, 35.5, 36.9, 60.9, 83.1, 126.0, 128.1, 128.4, 130.7, 130.8, 141.3, 145.4; MS (FAB) m/z 329 (M++H).
3d: colorless oil; Rf = 0.23 (10% EtOAc in hexane); IR (neat) 1742 cm-1; 1H NMR (300 MHz, CDCl3) δ 1.67 (1H, m), 1.98 (1H, m), 2.08 (1H, m), 2.26-2.36 (2H, m), 2.41 (2H, td, J = 7.4 and 5.5 Hz), 2.73 (2H, t, J = 7.4 Hz), 3.38 (1H, m), 5.75 (1H, d, J = 15.5 Hz), 5.83 (1H, dt, J = 15.5 and 5.5 Hz), 6.24 (1H, s), 7.12=7.30 (5H, m); 13C NMR (75 MHz, CDCl3) δ 26.8, 34.4, 35.3, 38.3, 41.9, 43.9, 77.8, 126.0, 127.3, 128.3, 128.4, 134.3, 141.0, 149.6, 217.9; MS (FAB) m/z 367 (M++H).
3e: pale yellow oil; Rf = 0.41 (20% EtOAc in hexane); IR (neat) 1742 cm-1; 1H NMR (300 MHz, CDCl3) δ 0.97 (3H, d, J = 6.7 Hz), 1.60 (1H, m), 1.93 (1H, m), 2.00 (1H, m), 2.19 (1H, m), 2.38 (1H, m), 2.42 (2H, td, J = 7.4 and 6.4 Hz), 2.74 (2H, t, J = 7.4 Hz), 2.96 (1H, tt, J = 12.1 and 6.7 Hz), 5.76 (1H, d, J = 15.6 Hz), 5.86 (1H, dt, J = 15.6 and 6.4 Hz), 6.33 (1H, s), 7.14-7.30 (5H, m); 13C NMR (75 MHz, CDCl3) δ 11.8, 24.3, 34.4, 35.3, 36.8, 48.2, 51.3, 78.6, 125.9, 127.1, 128.3, 128.4, 134.2, 141.0, 149.0, 218.9; MS (FAB) m/z 381 (M++H).
3f: colorless oil; Rf = 0.19 (10% EtOAc in hexane); IR (neat) 1704 cm-1; 1H NMR (300 MHz, CDCl3) δ 1.50 (1H, m), 1.63-1.79 (2H, m), 2.03-2.27 (4H, m), 2.36-2.45 (3H, m), 2.74 (2H, t, J = 7.4 Hz), 2.99 (1H, tt, J = 12.4 and 4.0 Hz), 5.72-5.86 (2H, m), 6.17 (1H, s), 7.15-7.31 (5H, m); 13C NMR (75 MHz, CDCl3) δ 25.1, 28.6, 34.4, 35.3, 40.9, 44.8, 45.9, 76.7, 125.9, 127.6, 128.3, 128.5, 134.0, 141.1, 150.5, 210.2; MS (FAB) m/z 381 (M++H).
(3E)-2-Methyl-6-phenyl-1,3-hexadiene 12. The reaction was performed by the same method described in the general procedure except quenching with 3 eq of acetic acid instead of iodine at rt. Crude product was purified by chromatography on Al2O3 to give terminal diene in 93% yield. Yellow oil; Rf = 0.40 (hexane); 1H NMR (300 MHz, CDCl3) δ 1.82 (3H, s), 2.41 (2H, td, J = 7.9 and 6.8 Hz), 2.72 (2H, t, J = 7.9 Hz), 4.87 (2H, s), 5.69 (1H, dt, J = 15.7 and 6.8 Hz), 6.17 (1H, d, J = 15.7 Hz), 7.15-7.30 (5H, m); 13C NMR (75 MHz, CDCl3) δ 18.7, 34.6, 36.0, 114.6, 125.8, 128.3, 128.4, 129.8, 133.4, 141.9, 142.0; MS (EI) m/z (rel. intensity) 172 (M+, 49), 157 (25), 91 (base). HRMS (EI) m/z Calcd for C13H16 (M+) 172.1252. Found 172.1242.
Isomerization conditions and formation of isomerized products. The reaction was conducted at 0 °C in all the process including the addition of Bu3SnMgMe described above.
4: colorless oil; Rf = 0.31 (hexane); 1H NMR (300 MHz, CDCl3) δ 1.93 (3H, s), 2.46 (2H, td, J = 7.8 and 7.3 Hz), 2.74 (2H, t, J = 7.8 Hz), 5.59 (1H, dt, J = 11.6 and 7.3 Hz), 5.90 (1H, d, J = 11.6 Hz), 6.04 (1H, s), 7.16-7.30 (5H, m); 13C NMR (75 MHz, CDCl3) δ 24.4, 31.0, 35.6, 77.7, 128.1, 128.3, 128.4, 131.0, 132.9, 141.6, 144.2; MS (EI) m/z (rel. intensity) 298 (M+, 36), 207 (12), 171 (38), 91 (base). HRMS (EI) m/z Calcd for C13H15I (M+) 298.0219. Found 298.0232.
6: colorless oil; Rf = 0.38 (hexane); 1H NMR (300 MHz, CDCl3) δ 1.86 (3H, s), 2.49 (2H, td, J = 7.6 and 7.3 Hz), 2.70 (2H, t, J = 7.6 Hz), 5.44 (1H, dt, J = 11.5 and 7.3 Hz), 5.82 (1H, d, J = 11.5 Hz), 5.93 (1H, s), 7.15-7.32 (5H, m); 13C NMR (75 MHz, CDCl3) δ 24.8, 30.5, 36.0, 77.2, 126.0, 128.4, 128.5, 130.1, 131.2, 141.4, 144.3; MS (EI) m/z (rel. intensity) 298 (M+, 7), 32 (base). HRMS (EI) m/z Calcd for C13H15I (M+) 298.0219. Found 298.0205.
7: colorless oil; Rf = 0.32 (hexane); 1H NMR (300 MHz, CDCl3) δ 1.93 (3H, s), 2.50 (2H, td, J = 8.0 and 6.8 Hz), 2.77 (2H, t, J = 8.0 Hz), 5.94 (1H, dt, J = 15.6 and 6.8 Hz), 6.00 (1H, s), 6.46 (1H, d, J = 15.6 Hz), 7.18-7.30 (5H, m); 13C NMR (75 MHz, CDCl3) δ 21.1, 34.7, 35.5, 77.4, 125.9, 128.3, 128.3, 132.1, 134.8, 141.5, 141.7; MS (EI) m/z (rel. intensity) 298 (M+, 33), 283 (2), 207 (6), 171 (10), 91 (56), 17 (base). HRMS (EI) m/z Calcd for C13H15I (M+) 298.0219. Found 298.0209.
Synthesis of 12 and 13. Compounds 12 and 13 were obtained by the reaction described for the synthesis of 3a. These compounds were able to separate by flash chromatography on silica gel. 12: colorless oil; Rf = 0.40 (hexane); 1H NMR (400 MHz, CDCl3) δ 1.96 (3H, s), 3.30 (2H, d, J = 7.5 Hz), 5.58 (1H, dt, J = 11.3 and 7.5 Hz), 5.92 (1H, dd, J = 11.3 and 1.2 Hz), 6.14 (1H, s), 6.26 (1H, br s), 7.21 (1H, t, J = 1.0 Hz), 7.36 (1H, t, J = 1.6 Hz); 13C NMR (75 MHz, CDCl3) δ 24.5, 24.9, 80.4, 110.8, 123.6, 129.4, 130.5, 138.9, 143.0, 144.0; MS (EI) m/z (rel. intensity): 274 (M+), 147 (M–I, base), 129 (45); HRMS (EI) m/z: Calcd for C10H11IO, 273.9855; found 273.9861. 13: colorless oil; Rf = 0.40 (hexane); 1H NMR (300 MHz, CDCl3) δ: 1.95 (3H, d, J = 1.1 Hz), 3.31 (2H, d, J = 7.0 Hz), 5.97 (1H, dt, J = 15.4 and 7.0 Hz), 6.05 (1H, s), 6.30 (1H, s), 6.50 (1H, d, J = 15.4 Hz), 7.24 (1H, s), 7.38 (1H, s); 13C NMR (75 MHz, CDCl3) δ: 21.2, 28.5, 78.1, 111.1, 122.7, 132.7, 133.1, 139.2, 141.6, 143.0; MS (EI) m/z (rel. intensity): 274 (M+), 147 (M–I, base), 129 (39); HRMS (EI) m/z: Calcd for C10H11IO, 273.9855. Found 273.9857.
Synthesis of dehydrohdendrolasin (8). To a degassed solution of 13 (28 mg, 0.1 mmol) and (E)-3-methyl-1-butenyl-1,3,2-benzodioxaborole (28 mg, 0.15 mmol) in THF (1.9 mL), were added palladium tetrakistriphenylphosphine (8 mg, 7 µmol) and silver carbonate (36 mg, 0.13 mmol) at rt. Aqueous solution of potassium hydroxide (2M, 0.18 mL) was added to the mixture and stirred for 17 h. The mixture was diluted with pentane (20 mL) and washed with water (2 mL), brine (1 mL X2), and dried over MgSO4. Solvent was removed and the residue was chromatographed on silica gel eluted with pentane to give 8 (17.8 mg) in 82% yield. Colorless oil; Rf = 0.51 (1.25% EtOAc in hexane). UV (cyclohexane) λmax nm (ε) 284 (31300), 273 (42800), 264 (35400), 252 (21000, sh), 209 (16700);1H NMR (300 MHz, CDCl3) δ: 1.02 (6H, d, J = 7.0 Hz), 1.86 (3H, s, C4-Me), 2.37 (1H, oct, J = 6.7 Hz, C8-H), 3.30 (2H, d, J = 6.8 Hz), 5.63 (1H, dd, J = 15.0 and 7.0 Hz, C7-H), 5.77 (1H, dt, J = 15.4 and 6.8 Hz, C2-H), 5.88 (1H, d, J = 11.2 Hz, C5-H), 6.30 (1H, d, J = 0.8 Hz, furan ring), 6.44 (1H, dd, J = 15.0 and 11.2 Hz, C6-H), 6.71 (1H, d, J = 15.4 Hz, C3-H), 7.24 (1H, s, furan ring), 7.37 (1H, d, J = 1.5 Hz, furan ring); 13C NMR (75 MHz, CDCl3) δ: 20.6, 22.5 (2C), 28.8, 31.5, 111.1, 122.5, 123.6, 128.1, 128.3, 128.7, 131.4, 139.1, 141.6, 142.9; MS-EI (%) m/z: 216 (M+), 78 (base). HRMS (FAB) m/z: Calcd for C15H20O, 216.1514. Found 216.1524.
ACKNOWLEDGEMENTS
This work was partially carried out at the Okayama University of Science as part of R. K.’s graduate study, and was financially supported by Grant-in-Aid for Scientific Research from the Ministry of Education, Science and Culture, Japan.
References
1. Leading review, E. Negishi, G. Wang, H. Rao, and Z. Xu, J. Org. Chem., 2010, 75, 3151. CrossRef
2. A. Suzuki, Angew. Chem. Int. Ed., 2011, 50, 6722 and cited therein. CrossRef
3. I. Marek and J. F. Normant, ‘Carbometallation Reactions’ in ‘Metal-catalyzed Cross-coupling Reactions’, ed. by F. Diederich and P. J. Stang, Wiley-VCH Weinheim, 1998, pp. 271-337. CrossRef
4. E. Negishi, Acc. Chem. Res., 1987, 20, 65. CrossRef
5. J. F. Normant and A. Alexakis, Synthesis, 1981, 841. CrossRef
6. P. Knochel, ‘Comprehensive Organic Synthesis’, Vol. 4, eds by I. Fleming, B. M. Trost, M. F. Semmelhack,. Pergamon Press, Oxford, 1991, pp 865-911.
7. J. Hibino, S. Matsubara, Y. Morizawa, K. Oshima, and H. Nozaki, Tetrahedron Lett., 1984, 25, 2151; CrossRef S. Matsubara, J. Hibino, Y. Morizawa, K. Oshima, and H. Nozaki, J. Organomet. Chem., 1985, 285, 163. CrossRef
8. J. Uenishi, R. Kawahama, A. Tanio, and S. Wakabayashi, Chem. Commun., 1993, 1438. CrossRef
9. Compound 6 was obtained as a single isomer in 84% yield when reaction was carried out at –20 °C.
10. M. B. Ksebati and F. J. Schmitz, J. Nat. Prod., 1988, 51, 857. CrossRef
11. J. Uenishi, R. Kawahama, A. Tanio, S. Wakabayashi, and O. Yonemitsu, Tetrahedron, 1997, 53, 2439. CrossRef
12. Preparation of 12 used for the synthesis of 10 was performed by Negishi’s carbometallation reaction, see in ref. 11.