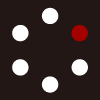
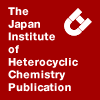
HETEROCYCLES
An International Journal for Reviews and Communications in Heterocyclic ChemistryWeb Edition ISSN: 1881-0942
Published online by The Japan Institute of Heterocyclic Chemistry
e-Journal
Full Text HTML
Received, 29th September, 2012, Accepted, 12th November, 2012, Published online, 22nd November, 2012.
■ DIRECT OXIDATION OF 4-METHYLPYRROLE-2-CARBOXYLATES WITH DDQ IN THE PRESENCE OF A GLYCOL
Kana Takahashi, Ryoji Iwamoto, Ryo Sakata, Takahiro Soeta, Katsuhiko Inomata, and Yutaka Ukaji*
Chemistry Course, Graduate School of Natural Science and Technology, Kanazawa University, Kakuma, Kanazawa, Ishikawa 920-1192, Japan
Abstract
Oxidation of 4-methylpyrrole-2-carboxylates with DDQ in the presence of a glycol proceeded smoothly on the methyl group at the C4 position regioselectively to afford the corresponding pyrrole-2,4-dicarboxylates directly. Direct oxidation of a methyl group of 2,4,6-trimethylphenol and 3-methyl-9H-carbazole into carboxylates was also demonstrated.2,3-Dichloro-5,6-dicyano-1,4-benzoquinone (DDQ) is a highly effective oxidant widely used in organic synthesis and its major functions include dehydrogenation, such as aromatization of hydroaromatic compounds, introduction of a double bond into carbonyl compounds, and oxidation of alcohols. Benzylic oxidation with DDQ in the presence of oxygen nucleophiles gives the corresponding oxygen-functionalized compounds. Furthermore, alternative oxidative cyclization and coupling reactions have been also reported.1,2
During our investigation on the syntheses of phytochromobilin (PΦB), phycocyanobilin (PCB), biliverdin (BV) and their analogs including sterically locked derivatives toward elucidation of the structure and function of phytochromes,3 oxidative functionalization of pyrroles by the use of quinones was explored. In the case that DDQ was used as an oxidant, the α-position of the alkyl substituent at the C4 position was regioselectively oxidized in the presence of AcOH to afford 4-(1-acetoxyalkyl)pyrrole derivatives. In contrast, the corresponding 4-acylpyrroles were produced when MeOH was used instead of AcOH as a nucleophile (Scheme 1).4 The oxidative transformation of 4-methylpyrrole-2-carboxylates with DDQ and MeOH was successfully applied to the convergent synthesis of sterically locked 5Za15Ea-BV derivative.5,6 Herein we describe a regioselective direct oxidation of t-butyl 4-methylpyrrole-2-carboxylates with DDQ in the presence of a glycol as a nucleophile to afford the corresponding 2,4-dicarboxylates.
First, oxidation of t-butyl 3-[2-(allyloxycarbonyl)ethyl]-4-methyl-1H-pyrrole-2-carboxylate (1A), which is a useful synthon for the B- and C-ring components of bilin chromophores,3 was examined by the use of 4.0 equiv of DDQ in the presence of 15 equiv of ethylene glycol (2a) in CH2Cl2 in order to trap the oxidation product as an acetal form. To our surprise, a desired 1,3-dioxolane 6Aa was not obtained but the corresponding 2-hydroxyethyl ester 3Aa was obtained in 50% yield (Table 1, Entry 1). The 2-hydroxyethyl ester 3Aa might be produced as follows: Oxidation of 1A with DDQ occurred at the C4 position to afford an intermediate 5Aa via nucleophilic attack of the glycol 2a to an intermediary azafulvene 4A.4 The second oxidation of 5Aa with DDQ furnished 6Aa, which was subjected to the third oxidation, followed by hydrolysis resulting in the formation of 3Aa as shown in Scheme 2,since the acetal 6Aa might be more easily oxidized by DDQ than 5Aa. Then propylene glycol (2b) instead of ethylene glycol (2a) was used as a nucleophile for the present oxidation. The corresponding 3-hydroxypropyl ester 3Ab was obtained in slightly enhanced chemical yield (Entry 2). The oxidation in the presence of 1,4-butanediol (2d) instead of ethylene glycol (2a) also proceeded to give the corresponding ester 3Ad, but in lower yield along with the formation of aldehyde 8A (Entry 11).
The oxygen atom of the resulting ester carbonyl might be originated from H2O. When the oxidation was carried out in the presence of 1 equiv of H2O, the chemical yield was improved as expected (Entry 3). When the amount of H2O was increased, the chemical yield was decreased (Entries 4 and 5) and the aldehyde 8A was obtained (Entry 5). In the present oxidation, the production of a small amount of a dimeric compound 9 was often observed.7
In order to suppress the production of the dimer, the pyrrole 1A and H2O were added to the mixture of glycol 2b and DDQ in a period of 3 h to obtain the ester 3Ab in enhanced 71% yield (Entry 6).8 Slow addition of 1A and H2O in a longer period did not improve the yield any more (Entry 7). Furthermore, increase of the amount of DDQ or glycol was not so effective (Entries 8 and 9). The use of 2,2-dimethyl-1,3-propanediol (2c) as a glycol realized a similar result (Entry 10).
Next, the oxidation of other pyrroles with DDQ in the presence of a glycol was examined as listed in Table 2, together with the results of oxidation of 1A. The reaction of t-butyl 3-ethyl-4-methyl-1H-pyrrole-2-carboxylate (1B) afforded esters 3Bb and 3Bc in 58% and 59% yields by the use of propylene glycol (2b) and 2,2-dimethyl-1,3-propanediol (2c), respectively (Entries 3 and 4). 3,4-Dimethyl-1H-pyrrole-2-carboxylate (1C) was oxidized regioselectively at the C4 methyl group (Entries 5 and 6). In this case, chemical yield was enhanced up to 71% by the use of 2,2-dimethyl-1,3-propanediol (2c) as a glycol. In the case of 3-phenyl-substituted pyrrole 1D in the presence of 1b, the reaction proceeded rather slowly to give the corresponding aldehyde 8D as a major product, but the yield of an ester 3Db was poor (Entry 7). In the oxidation using the glycol 2c at 40 °C, reaction was still sluggish to give the ester 3Dc in 27 % yield along with the aldehyde 8D (6%) and an acetal 6Dc (13%) (Entry 8).
In order to reveal the reaction mechanism of the present oxidation, the 1,3-dioxolane 6Aa (Scheme 2) was treated with DDQ in the presence of ethylene glycol (2a): The oxidized ester 3Aa was obtained in 63% yield accompanied with aldehyde 8A (7%) (Eq. 1).9 This fact supported that the ester was produced via oxidation of the intermediary acetal 6Aa. Although the oxidation of the 3-phenyl substituted acetal 6Dc with DDQ was also examined at 40 °C, the reaction was sluggish and the yield of ester 3Dc was low (Eq. 2). The exact reason for the poor reactivity of the phenyl-substituted acetal 6Dc toward DDQ oxidation is not yet clear, however, steric hindrance of phenyl group, which prevents the attack of DDQ to 6Dc, might be a possible reason.
It is well known that DDQ oxidation of methyl group on aromatic ring in the presence of MeOH and/or H2O gives the corresponding aldehydes.1,2h,10 Thus, the present DDQ oxidation using a glycol as a nucleophile was applied to such substrates. 2,4,6-Trimethylphenol (mesitol) (10)2h was converted to an ester 11 in 77% yield regioselectively in the presence of glycol 2c (Eq. 3). Carbazole compounds are intriguing because of containing pyrrole skeleton.10c-e 3-Methyl-9H-carbazole (12) was subjected to the present DDQ oxidation to afford the corresponding ester 13 in 66% yield (Eq. 4).
As described above, the regioselective oxidation of t-butyl 4-methyl-1H-pyrrole-2-carboxylate was achieved with DDQ in the presence of a glycol to give the corresponding pyrrole-2,4-dicarboxylates.11 This method could be also applied to the DDQ oxidation of methyl groups attached to aromatic rings into the corresponding esters. The direct oxidation of methylarenes to the carboxylic acids and its derivatives is very important, since such carbonyl derivatives are versatile building blocks in the synthesis of pharmaceutical chemicals. A variety of oxometal oxidants have been employed for such oxidative processes.12 The present reaction would provide an efficient method for metal-free oxidation to produce useful carboxylic acid derivatives.
ACKNOWLEDGEMENTS
The present work was financially supported in part by the Mitani Foundation for Research and Development and a Grant-in-Aid for Scientific Research (B) from Japan Society for the Promotion of Science (JSPS).
References
1. D. Walker and J. D. Hiebert, Chem. Rev., 1967, 67, 153; CrossRef A. B. Turner, 'Synthetic Reagents,' Vol. 3, ed. by J. S. Pizey, Halstead-Wiley, New York, 1977, 193; D. R. Buckle and I. L. Pinto, 'Comprehensive Organic Synthesis,' Vol. 7, ed. by B. M. Trost and I. Fleming, Pergamon Press, New York, 1991, pp.135–139.
2. Examples of oxidation reaction using DDQ. Aromatization: a) P. P. Fu and R. G. Harvey, Chem. Rev., 1978, 78, 317; CrossRef b) U. Kämpfen and A. Eschenmoser, Tetrahedron Lett., 1985, 26, 5899; CrossRef Benzylic oxidation: c) H. Lee and R. G. Harvey, J. Org. Chem., 1983, 48, 749; CrossRef d) T. Ishiguro, H. Mizuguchi, K. Tomioka, and K. Koga, Chem. Pharm. Bull., 1985, 33, 609; CrossRef e) E. J. Corey and Y. B. Xiang, Tetrahedron Lett., 1987, 28, 5403; CrossRef Oxidation of alcohol: f) H.-D. Becker, A. Björk, and E. Adler, J. Org. Chem., 1980, 45, 1596; CrossRef g) L. Wang, J. Li, H. Yang, Y. Lv, and S. Gao, J. Org. Chem., 2012, 77, 790; CrossRef Oxidative coupling and cyclization reaction: h) H.-D. Becker, J. Org. Chem., 1965, 30, 982; CrossRef i) Y. Zhang and C.-J. Li, J. Am. Chem. Soc., 2006, 128, 4242; CrossRef j) L. Liu and P. E. Floreancig, Org. Lett., 2010, 12, 4686. CrossRef
3. H. Khawn, L.-Y. Chen, H. Kinoshita, and K. Inomata, Chem. Lett., 2008, 37, 198; CrossRef K. Inomata, Bull. Chem. Soc. Jpn., 2008, 81, 25; CrossRef L.-Y. Chen, H. Kinoshita, and K. Inomata, Chem. Lett., 2009, 38, 602; CrossRef K. Inomata, H. Khawn, L.-Y. Chen, H. Kinoshita, B. Zienicke, I. Molina, and T. Lamparter, Biochemistry, 2009, 48, 2817; CrossRef K. Nishiyama, A. Kamiya, M. A. S. Hammam, H. Kinoshita, S. Fujinami, Y. Ukaji, and K. Inomata, Bull. Chem. Soc. Jpn., 2010, 83, 1309; CrossRef R. Yang, K. Nishiyama, A. Kamiya, Y. Ukaji, K. Inomata, and T. Lamparter, Plant Cell, 2012, 24, 1936 and references cited therein. CrossRef
4. R. Iwamoto, Y. Ukaji, and K. Inomata, Chem. Lett., 2010, 39, 176. CrossRef
5. L.-Y. Chen, R. Iwamoto, Y. Ukaji, and K. Inomata, Chem. Lett., 2011, 40, 632. CrossRef
6. Related oxidation reaction using o-chloranil: R. Sakata, R. Iwamoto, S. Fujinami, Y. Ukaji, and K. Inomata, Heterocycles, 2011, 82, 1157. CrossRef
7. The dimeric compound 9 might be formed by Friedel-Crafts-type reaction of the azafulvene 4A with unreacted 1A followed by further oxidation with DDQ as shown in Scheme 3.
8. Slow addition procedure was also effective even in the case of ethylene glycol (2a) and 1,4-butanediol (2d) to give the corresponding esters 3Aa and 3Ad in 57% and 62% yields accompanied with the aldehyde 8A in 7% and 17% yields, respectively.
9. It was reported that 1,3-dioxolanes and 1,3-dioxanes with an aromatic group at C2 position, especially with an electron-donating p-methoxyphenyl group, were oxidized by DDQ into the corresponding carboxylates and their equivalents, for example: a) J.-I. Iwamura, N. Iwamoto, and N. Hirao, Nippon Kagaku Kaishi, 1977, 1009; CrossRef b) K. Nakayama and J. D. Rainier, Tetrahedron, 1990, 46, 4165; CrossRef c) B. Wünsch and S. Nerdinger, Eur. J. Org. Chem., 1999, 503; CrossRef d) H. Tanabe, X. He, P. Kothandaraman, and M. Yamane, Synlett, 2010, 1190. CrossRef
10. Recent examples of DDQ oxidation of methyl groups attached to aromatic rings to the corresponding aldehydes: a) J. H. P. Utley and G. G. Rozenberg, Tetrahedron, 2002, 58, 5251; CrossRef b) S. Nandi, K. Panda, J. R. Suresh, H. Ila, and H. Junjappa, Tetrahedron, 2004, 60, 3663; CrossRef c) R. Forke, M. P. Krahl, F. Däbritz, A. Jäger, and H.-J. Knölker, Synlett, 2008, 1870; CrossRef d) M. Schmidt and H.-J. Knölker, Synlett, 2009, 2421; CrossRef e) R. Bautista, P. Bernal, L. E. Montiel, F. Delgado, and J. Tamariz, Synthesis, 2011, 929. CrossRef
11. A representative procedure for the oxidation of 1A (Table 1, Entry 6): To a solution of DDQ (454 mg, 2.0 mmol) and 1,3-propanediol (571 mg, 7.5 mmol) in CH2Cl2 (9 mL) was slowly added a solution of 1A (147 mg, 0.5 mmol) and H2O (9 mg, 0.5 mmol) in CH2Cl2 (6 mL) in a period of 3 h at rt under a nitrogen atmosphere. After stirring for another 16 h at rt, the reaction mixture was quenched by the addition of an aqueous solution containing ascorbic acid (0.7%), citric acid (1.3%), and sodium hydroxide (0.9%). The mixture was extracted with ether and the combined extracts were washed with brine, and dried over Na2SO4. The solvent was evaporated and the residue was separated by TLC on SiO2, which was pretreated with hexane/Et3N (100/1, v/v), (hexane/AcOEt = 2/1, v/v) to afford 3Ab (136 mg, 71%) as an oil. 3Ab: IR (neat) 3308, 2976, 1734, 1716, 1697, 1557, 1541, 1507, 1457, 1417, 1369, 1288, 1141, 1050, 943, 840, 786, 757 cm-1. 1H NMR (400 MHz, CDCl3) δ 1.58 (s, 9H), 1.93–1.99 (m, 2H), 2.54 (br, 1H), 2.60 (t, 2H, J = 8.3 Hz), 3.40 (t, 2H, J = 8.3 Hz), 3.71–3.79 (m, 2H), 4.40 (t, 2H, J = 6.0 Hz), 4.59 (d, 2H, J = 6.0 Hz), 5.22 (d, 1H, J = 10.6 Hz), 5.30 (d, 1H, J = 17.0 Hz), 5.86–5.97 (m, 1H), 7.48 (d, 1H, J = 3.6 Hz), 9.87 (br, 1H). 13C NMR (100 MHz, CDCl3) δ 20.9, 28.2, 31.8, 35.2, 59.0, 60.6, 64.9, 82.0, 115.7, 118.0, 122.1, 127.2, 130.5, 132.1, 161.0, 164.7, 172.8. HRMS (FAB+) (M+ + H), found: m/z 382.1864. Calcd for C19H28NO7: 382.1866.
12. R. A. Sheldon and J. K. Kochi, 'Metal-Catalyzed Oxidations of Organic Compounds,' Academic Press, New York, 1981, Chap. 10; A. H. Haines, 'Methods for the Oxidation of Organic Compounds,' Academic Press, London, 1985, Chap. 2.5.