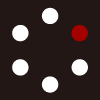
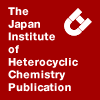
HETEROCYCLES
An International Journal for Reviews and Communications in Heterocyclic ChemistryWeb Edition ISSN: 1881-0942
Published online by The Japan Institute of Heterocyclic Chemistry
e-Journal
Full Text HTML
Received, 22nd June, 2012, Accepted, 23rd July, 2012, Published online, 2nd August, 2012.
DOI: 10.3987/COM-12-S(N)50
■ SYNTHESIS OF BIS-nAPHTHOPORPHYRINS
Hiroki Uoyama, Hiroko Yamada, Tetsuo Okujima, and Hidemitsu Uno*
Department of Chemistry and Biology, Graduate School or Science and Engineering, Ehime University, Bunkyo-cho 2-5, Matsuyama 790-8577, Japan
Abstract
Ethanonaphthoporphyrins with a halogen atom at the ζ position were prepared by the [3+1] porphyrin synthesis of halogen-substituted ethanobenz[f]isoindole with a tripyrrane derivative. The halogeno porphyrins were converted to the corresponding ethynyl and pinacolatoboronyl compounds by Sonogashira and borylation reactions, respectively. Suzuki, Sonogashira, and Glaser coupling reactions of these ethanonaphthoporphyrins gave bis-porphyrins connected with no atom, acetylene, and butadiyne, respectively. Retro Diels-Alder reaction of these bis-porphyrins brought about the conversion of bicyclo[2.2.2]octadiene to benzene moieties to give bis-naphthoporphyrins, quantitatively.INTRODUCTION
Porphyrin dimers, trimers and oligomers1 have continuously attracted attention as host molecules for carbon materials such as fullerenes and nanotubes,2 as near infrared dyes,3 and as artificial models for a light harvesting antenna porphyrin system.4 For these applications, not only the electronic properties of porphyrins but also the spacer between the porphyrins are important. When the porphyrin rings are directly connected, interaction of the porphyrin π-systems is small because these porphyrin rings tend to orient nearly perpendicularly due to steric repulsion regardless of the connecting positions.5 Therefore, electronic structures of the oligomers are not greatly different from those expected by summation of the monomeric porphyrins and only small perturbation to electronic states is observed. When spacer groups are employed to connect the porphyrin rings, the spacer properties become important. In the case of aryl spacers, the π-planes of spacers and porphyrin rings tend to be perpendicular and the interaction is small, too.6 When alkyl chains are used, the length is important for communication of the porphyrin chromophores.7 On the other hand, alkyne spacers are quite different and electronic states of the porphyrin rings are well mixed to form large delocalized chromophores.8 Introduction of the alkyne groups can be achieved by the reliable method, namely Sonogashira reaction.9 As the alkyne groups are easily converted to other functional groups, many successful examples for the modification of porphyrin chromophores were reported.10 For Sonogashira reaction, porphyrin halogenated at a desired position is required. Selective halogenation of porphyrin is rather difficult because easily available porphyrins such as tetraphenylporphyrin and octaethylporphyrin have high symmetry of D4h.11 An alternative method for preparation of halogenated porphryins from pyrroles bearing halogen atoms become important. When a pyrrole derivative bearing iodine atoms at β-positions was employed for the porphyrin synthesis, the aimed diiodoporphyrin was obtained only in a very low yield.12 During the porphryin synthesis under such acidic conditions, a halogen atom directly substituted on the pyrrole ring is sometimes lost by electrophilic attack of proton on the pyrrole ring.13 Therefore, the pyrroles with halogen atoms in distal positions were preferably employed for this purpose.14 The connecting moieties between pyrrole nucleus and halogen atoms are important. During the course of our continuous investigation for the preparation of π-expanded porphyrins, we prepared pyrroles connected with halogenated benzene through bicyclo[2.2.2]octadiene in order to combine the porphyrin chromophores. In this paper, we demonstrate the synthesis of halogenated ethanonaphthoporphyrins 1 and their conversion to naphthoporphyrins 8 and diporphyrins 2–7.
RESULTS AND DISCUSSION
Synthesis
The new halogenated pyrroles 14b and 14c were prepared according to the modified method reported in the literature (Scheme 1).15 The Diels-Alder reaction of benzynes generated from anthranilic acids 9 with 1,3-cyclohexadiene gave 1,4-dihydro-1,4-ethanonaphthalene derivatives,16 which were roughly purified by silica-gel column chromatogaphy and used without further purification. Treatment of the crude products with benzenesulfenyl chloride gave sulfides 10 in moderate yields (two steps). Sulfides 10 were oxidized with mCPBA to give β-chloro sulfones 11 in good yields. Elimination of hydrogen chloride from 11 with DBU afforded α,β-unsaturated sulfones 12 in good yields. Pyrrolecarboxylate esters 13 were obtained in good yields by the modified Barton-Zard pyrrole synthesis using sulfones 12 and ethyl isocyanoacetate with the aid of KO-t-Bu. Removal of the ester moiety from pyrrolecarboxylates 13 was achieved by heating 13 with KOH in ethylene glycol at 175 °C for 2 h. α-Free pyrroles 14 were obtained in good yields. The total yields of pyrroles 14a, 14b, and 14c from corresponding anthranilic acids 9 were 22, 24, and 17%, respectively.
Porphyrins 1a–c were obtained by using the inverse mode17 of [3+1] method (Scheme 2).18 Condensation of the pyrrole 14 and tripyrranedicarboaldehyde 1519 followed by oxidation with DDQ and treatment with Zn(OAc)2·2H2O gave porphyrins 1a, 1b, and 1c in respective yields of 38, 43, and 41%.
Preparation of bis-porphyrins 2–4 are shown in Scheme 3. Since the preparation of directly linked bis-porphyrin 2 by the Ullmann homo coupling reaction of either 1b or 1c with Cu failed, we employed the Suzuki-Miyaura coupling reaction. Pinacolatoboronyl derivative 1e was obtained by the reaction of porphyrins 1b–c and bis(pinacolato)diboron with PdCl2(PPh3)2 in 73 and 75% yields, respectively. The Suzuki-Miyaura cross coupling reaction between 1b–c and pinacolatoboronated porphyrin 1e led to formation of 2 in 61 and 57% yields, respectively. The Sonogashira coupling reaction between iodoporphyrin 1c and trimethylsilylacetylene gave acetylene-substituted porphyrin 1d in a 93% yield, while the similar reaction of bromo derivative 1b was unsuccessful. The trimethylsilyl group of 1d was removed with tetrabutylammonium fluoride to give 1f in an 88% yield. Acetylene-linked porphyrin dimer 3 was prepared by the Sonogashira coupling reaction between porphyrins 1c and 1f in a 76% yield. On the other hand, butadiyne-linked bis-porphyrin 4 was synthesized by the Eglinton modification of the Glaser coupling reaction of 1f in an 87% yield.
Preparative conversions of the BCOD-fused porphyrins and bisporphyrins 1–4 were performed at 290 °C under a reduced pressure (ca. 7 Pa) for 10 min according to our previous report,15 whereby the naphthoporphyrin derivatives 5–8 were obtained in quantitative yields. Bis-naphthoporphyrins 5–7 were insoluble in common solvents such as chloroform, dichloromethane, and toluene, but slightly soluble in pyridine and THF.
UV-vis Spectra of Properties and DFT calculations
UV-vis spectra of BCOD-fused porphyrins 1a, 2, 3, and 4 in CHCl3 are shown in Figure 2. BCOD-fused porphyrin monomer 1a showed the Soret band absorption at 402 nm and two Q band absorptions at 531 and 570 nm. In BCOD-fused porphyrin dimers 2, 3, and 4, the Soret and Q bands showed strong absorptions with no bathochromic shift compared to those of monomeric 1a and 1d because each porphyrin π-electronic system of 2, 3, and 4 was separated by the BCOD moiety.
UV-vis spectra of naphthoporphyrins 5–8 in pyridine are shown in Figure 2. Bathochromic shift of Soret- and Q-band absorptions was observed in naphthoporphyrins 5–8 compared to those of the corresponding BCOD-fused porphyrins 1–4. UV-vis spectra of bis-naphthoporphyrins 5–7 showed only small change compared to those of naphthoporphyrins 8. Soret band absorptions of bis-naphthoporphyrins 5–7 were quite similar and became broad with a slight bathochromic shift, while the Q band absorptions only looked like the sum of the Q band signals of naphthoporphyrin 8a.
In order to figure out electronic properties of bis-naphthoporphyrins, we performed DFT calculation (B3LYP/6-31G(d)-LanL2DZ) of 5, 6, 7, and 8a. Figures 3 and 4 show energy levels and some frontier MO diagrams of naphthoporphyrin 8a and dimers 5-7. The eight molecular orbitals (HOMO−3 ∼ LUMO+3) of dimers 5–7 can be approximately described as linear combinations of four molecular orbitals (HOMO−1 ∼ LUMO+1) of constituent monomers.20 The HOMO−1, HOMO, LUMO, and LUMO+1 of monomer constitute the following pairs of dimer orbitals: HOMO−3/HOMO−2, HOMO−1/HOMO, LUMO/LUMO+1, and LUMO+2/LUMO+3. AO coefficients at ζ-positions of naphthoporphyrin are nearly zero in HOMO−1 and small in HOMO, LUMO, and LUMO+1. Therefore, those of HOMO−3 and HOMO−2 of dimers are also nearly zero, because HOMO−3 and HOMO−2 of dimers consist of linear combination of HOMO-1 orbitals of the monomer. Thus, the HOMO−2/HOMO−3 pair shows no energy splitting. On the other hand, as HOMO−1 ∼ LUMO+3 of dimers have small AO coefficients on the connecting ζ-positions, the HOMO/HOMO−1, LUMO/LUMO+1, and LUMO+2/LUMO+3 pairs of dimers exhibit small energy splitting. Consequently, very similar electronic spectra are obtained especially in the Q-band region.
In conclusion, we have achieved the synthesis of ethanonaphthoporphyrins with a bromine or iodine atom at the ζ-position and their conversion to the corresponding ethynyl and pinacolatoboronyl compounds by Sonogashira and borylation reactions. These monomeric porphyrin compounds were successfully dimerized by the Suzuki, Sonogashira, and Glaser coupling reactions. Fully conjugated naphthoporphyrin dimers connected directly and with acetylene and butadiyne at ζ-positions were obtained by the retro Diels-Alder reaction. These naphthoporphyrin dimers are revealed to show the similar UV-vis absorptions to the corresponding monomers especially in the Q-band region.
EXPERIMENTAL
General: Melting points were measured on a Yanagimoto micromelting point apparatus and are uncorrected. Otherwise noted, NMR spectra were obtained in CDCl3 with a JEOL AL-400 or EX-400 spectrometer at the ambient temperature by using tetramethylsilane as an internal standard for 1H and 13C. IR spectra were measured with a Horiba FT-720 infrared spectrophotometer. EI and FAB MS were measured with a JEOL JMS-700. MALDI-TOF MS were measured on a Voyager DE Pro instrument (Applied Biosystems). Elemental analyses were performed with a Yanaco MT-5 elemental analyzer. Preparative GPC was carried out on a JAI LC-9801 chromatograph equipped with JAI-1H (Φ20 x 600 mm) and JAI-2H (Φ20 x 600 mm) columns. UV-vis spectra were measured with a HITACHI U-2810 spectrophotometer. All solvents and chemicals were reagent grade quality, obtained commercially, and used without further purification except as noted. Dry CH2Cl2 and THF were purchased from Kanto Chemical Co. Triethylamine, pyridine, and DBU, were distilled from calcium hydride and then stored on appropriate Molecular Sieves. Solvents for chromatography were purified by distillation. For spectral measurements, spectral grades of pyridine and chloroform were purchased from Nacalai Tesque Co. Thin-layer (TLC) and column chromatography with silica gel was performed on Art. 5554 (Merck KGaA), Silica Gel 60N (Kanto Chemical Co.). Tripyrrane 15 was prepared according to the literature.19
General procedure for preparation of sulfide 10: To a solution of anthranilic acid 9 (25.0 mmol) in THF (40 mL) was added CF3CO2H (0.04 mL) at 0 °C. The mixture was stirred for 10 min and isopenyl nitrite (5.01 mL, 3.75 mmol) was slowly added at the same temperature. The mixture was warmed to room temperature and stirred for 1.5 h. The mixture was cooled to 0 °C and white solid was formed. The solid was filtered and then washed with cooled ClCH2CH2Cl. Caution: The diazonium salt must be wet with the solvent, or it explodes! The wet solid was suspended in ClCH2CH2Cl (10 mL) and added to a refluxed solution of 1,3-cyclohexadiene (4.77 mL, 50.0 mmol) in ClCH2CH2Cl (150 mL). After the addition, the mixture was stirred for 2 h under reflux. The mixture was cooled to room temperature and concentrated under a reduced pressure. The black residue was purified by short column chromatography on silica gel (CHCl3, Rf = 0.9) to give a colorless crude product. Benzenesulfenyl chloride was added to a stirred solution of the crude product in dry CH2Cl2 (100 mL) at -78 °C under N2 until the orange color of PhSCl no more disappeared. The mixture was warmed to room temperature and stirred for 30 min. The reaction mixture was quenched with water and extracted with CH2Cl2. The organic extract was washed successively with aqueous NaHCO3, water, and brine, dried over Na2SO4, and concentrated under a reduced pressure. The residue was chromatographed on silica gel to give 10.
2-Chloro-3-phenyltho-1,4-dihydro-1,4-ethanonaphthalene (10a): Yield, 42%; colorless oil, Rf = 0.45 (30% EtOAc/hexane); 1H NMR δ 7.43 (2H, m), 7.28 (5H, m), 7.23 (2H, m), 3.83 (1H, m), 3.59 (1H, m), 3.22 (1H, m), 3.10 (1H, m), 2.38 (1H, m), 1.91 (1H, m), 1.54 (1H, m), and 1.34 (1H, m); 13C NMR δ 139.84, 139.19, 135,27, 134.43, 131.98, 129.18, 128.92, 127.19, 127.06, 125.75, 124.38, 124.22, 64.89, 57.23, 42.89, 40.32, 26.15, and 18.49; IR (KBr) νmax/cm-1 1583, 1481, 1439, 1101, 742, and 690; MS (EI) m/z (rel. intensity) 300 (M+, 100), 191 (13), 128 (29), and 77 (9). Anal. Calcd for C18H17ClS+1/2H2O: C, 69.77; H, 5.86. Found: C, 70.14; H, 5.85%.
6-Bromo-2-chloro-3-phenyltho-1,4-dihydro-1,4-ethanonaphthalene and 6-bromo-3-chloro-2-phenyltho-1,4-dihydro-1,4-ethanonaphthalene (10b): Yield, 36%; colorless oil, Rf = 0.45 (30% CHCl3/hexane); 1H NMR (diastereomeric mixture) δ 7.38−7.45 (3H, m), 7.23−7.35 (5H, m), 7.05 (1H, m), 3.78 (1H, m), 3.55 (1H, m), 3.20 (1H, m), 3.06 (1H, m), 2.37 (1H, m), 1.91 (1H, m), 1.52 (1H, m), and 1.33 (1H, m); 13C NMR (typical signals) δ 141.87, 141.31, 138.71, 138.10, 133.90, 132.16, 132.05, 130.00, 129.92, 128.95, 128.93, 128.77, 127.44, 127.39, 127.37, 127.32, 125.93, 120.81, 120.51, 64.12, 64.11, 56.84, 56.81, 42.62, 42.36, 40.23, 39.83, 25.88, 25.81, and 18.22; IR (KBr) νmax/cm-1 1574, 1475, 1439, 1414, 1259, 1238, and 1024; MS (EI) m/z (rel. intensity) 380 [M+(79Br)+1, 100], 271 (49), 235 (31), 208 (62), and 128 (83). Anal. Calcd for C18H16BrClS: C, 56.93; H, 4.25. Found: C, 56.94; H, 4.20%.
2-Chloro-6-iodo-3-phenyltho-1,4-dihydro-1,4-ethanonaphthalene and 3-chloro-6-iodo-2-phenyltho-1,4-dihydro-1,4-ethanonaphthalene (10c): Yield, 27%; colorless oil; Rf = 0.25 (10% CHCl3/hexane); 1H NMR (diastereomeric mixture) δ 7.50−7.67 (2H, m), 7.42 (2H, m), 7.23−7.32 (3H, m), 6.93 (1H, m), 4.68 (one of isomers, 1H, m), 3.92 (one of isomers, 1H, m), 3.78 (one of isomers, 1H, m), 3.53 (one of isomers, 1H, m), 3.33 (one of isomers, 1H, m), 3.10 (1H, m), 3.04 (one of isomers, 1H, m), 2.35 (one of isomers, 1H, m), 2.14 (one of isomers, 1H, m), 1.87 (, 1H, m), 1.49 (, 1H, m), 1.30 (one of isomer, 1H, m), and 1.08 (one of isomers, 1H, m); 13C NMR (typical signals) δ 144.43, 143.22, 142.22, 141.60, 139.43, 138.84, 136.70, 136.05, 134.97, 134.07, 133.99, 133.84, 133.25, 132.16, 132.08, 131.06, 127.73, 127.43, 127.37, 127.12, 126.28, 124.22, 92.59, 91.94, 64.13, 58.62, 56.72, 55.96, 51.13, 42.86, 42.35, 39.85, 29.14, 25.86, 25.73, and, 24.33; IR (KBr) νmax/cm-1 1583, 1473, 1408, 1259, 1173, 955, 820, 742, and 690; MS (EI) m/z (rel. intensity) 426 (M +, 100), 317 (40), 281 (37), 254 (31), and 128 (65). Anal. Calcd for C18H16ClIS+1/8H2O: C, 50.40; H, 3.82. Found: C, 50.20; H, 3.86%.
General procedure for oxidation of sulfide 10 to sulfone 11: To a solution of 10 (7.00 mmol) in dry CH2Cl2 (70 mL) was added mCPBA (16.8 mmol) at 0 °C. The mixture was then stirred for 2 h at room temperature. The mixture was quenched with saturated aqueous NaHCO3. The mixture was extracted with CH2Cl2. The organic extract was washed successively with water and brine, dried over Na2SO4, and concentrated under a reduced pressure. The residue was chromatographed on silica gel to give 11.
2-Chloro-3-phenylsulfonyl-1,4-dihydro-1,4-ethanonaphthalene (11a): Yield, 88%; colorless powder, mp 177−180 °C; Rf = 0.3 (30% EtOAc/hexane); 1H NMR (diastereomer mixture) δ 7.85 (2H, m), 7.67 (1H, m), 7.56 (2H, m), 7.29 (3H, m), 7.21 (1H, m), 4.25 (1H, m), 3.72 (1H, m), 3.56 (1H, m), 3.31 (1H, m), 2.34 (1H, m), 1.97 (1H, m), 1.56 (1H, m), and 1.42 (1H, m); 13C NMR (typical signals) δ 138.56, 138.01, 137.70, 133.77, 129.10, 128.61, 127.54, 127.27, 125.24, 124.16, 72.49, 56.85, 42.80, 34.64, 26.75, and 18.10; IR (KBr) νmax/cm-1 1444, 1302, 1146, 1086, 754, and 723; MS (EI) m/z (rel. intensity) 332 (M+, 20), 191, (100), 162 (8), 129 (93), and 77 (17). Anal. Calcd for C18H17ClO2S+1/2H2O: C, 63.24; H, 5.31. Found: C, 63.48; H, 5.12%.
6-Bromo-2-chloro-3-phenylfulfonyl-1,4-dihydro-1,4-ethanonaphthalene and 6-bromo-3-chloro-2-phenylsulfonyl-1,4-dihydro-1,4-ethanonaphthalene (11b): Yield, 94 %; colorless powder, mp 56−58 °C, Rf = 0.4 (30% EtOAc/hexane); 1H NMR (diastereomer mixture) δ 7.78−7.82 (2H, m), 7.51−7.67 (3H, m), 7.31−7.45 (2H, m), 7.14 (one isomer, 1H, m), 7.03 (another isomer, 1H, m), 4.18 (1H, m), 3.70 (another isomer, 1H, m), 3.63 (one isomer, 1H, m), 3.50 (1H, m), 3.25 (1H, m), 2.30 (1H m), 1.94 (1H, m), 1.53 (1H, m), 1.35 (1H, m); 13C NMR (typical signals) δ 140.59, 139.73, 137.91, 137.67, 137.50, 134.35, 134.06, 134.00, 130.67, 130.32, 130.16, 129.74, 129.25, 129.19, 128.73, 128.66, 128.45, 128.21, 127.37, 127.28, 127.02, 125.85, 121.30, 120.97, 72.36, 72.19, 56.44, 42.64, 42.38, 34.65, 34.18, 26.64, 26.54, 17.99, and 17.94; IR (KBr) νmax/cm-1 1477, 1444, 1411, 1295 and 1147; MS (EI) m/z (rel. intensity) 412 [M+(81Br), 92], 207, (51), 162 (71), and 128 (53). Anal. Calcd for C18H16BrClO2S+1/ 4CH2Cl2: C, 50.63; H, 3.84. Found: C, 50.35; H, 3.68%.
2-Chloro-6-iodo-3-phenylsulfonyl-1,4-dihydro-1,4-ethanonaphthalene and 3-chloro-6-iodo-2-phenylsulfonyl-1,4-dihydro-1,4-ethanonaphthalene (11c): Yield, 97%; colorless solid, mp 48−56 °C; Rf = 0.45 (CHCl3); 1H NMR (diastereomer mixture) δ 7.91−7.94 (1H, m), 7.76−7.81 (1H, m), 7.49−7.70 4H, m), 6.71 (another isomer, 1H, m), 6.90 (one isomer, 1H, m), 5.17 (another isomer, 1H, m), 4.16 (one isomer, 1H, m), 3.63 (both isomers, 1H, m), 3.48 (both isomers, 1H, m), 3.40 (another isomer, 1H, m), 3.22 (one isomer, 1H, m), 2.64 (another isomer, 1H, m), 2.27 (one isomer, 1H, m), 2.09 (another isomer, 1H, m), 1.92 (one isomer, 1H, m), 1.52 (another isomer, 1H, m), 1.27 (one isomer, 1H, m), 1.07 (another isomer, 1H, m), and 0.91 (one isomer, 1H, m); 13C NMR (typical signals) δ 143.00, 141.52, 140.84, 139.89, 138.15, 137.90, 137.62, 136.61, 136.25, 134.14, 134.06, 133.97, 133.10, 129.55, 129.23, 129.16, 128.72, 128.65, 127.76, 127.22, 126.04, 123.94, 92.76, 92.13, 72.26, 71.23, 56.43, 53.59, 48.63, 42.43, 40.69, 34.37, 29.14, 26.60, 24.10, and 17.94; IR (KBr) νmax/cm-1 1473, 1446, 1317, 1147, 1083, 957, and 723; MS (EI) m/z (rel. intensity) 458 (M +, 100), 422 (3), 394 (12), 333 (6), 317 (76), 281 (30), 254 (19), and 128 (83). Anal. Calcd for C18H16ClIO2S+1/8H2O: C, 46.90; H, 3.55. Found: C, 46.50; H, 3.55%.
General procedure for α,β-unsaturated sulfone 12: To a stirred solution of 11 (7.00 mmol) in dry CH2Cl2 (70 mL) was added DBU (7.7 mmol) at room temperature under N2. After 1 h, a 1.0-M aqueous HCl solution was added to the reaction mixture. The mixture was extracted with CH2Cl2. The organic extract was washed successively with saturated aqueous NaHCO3, water, and brine, dried over Na2SO4, and concentrated under a reduced pressure. The residue was chromatographed on silica gel to give 12.
2-Phenylsulfonyl-1,4-dihydro-1,4-ethanonaphthalene (12a): Yield, 90%, colorless oil, Rf = 0.2 (30% EtOAc/hexane); 1H NMR δ 7.79 (2H, m), 7.55 (1H, m), 7.46 (3H, m), 7.15 (1H, m), 7.07 (1H, m), 7.03 (1H, m), 6.99 (1H, m), 4.20 (2H, m), and 1.54 (4H, m); 13C NMR δ 146.98, 144.60, 144.48, 141.17, 139.47, 133.08, 128.98, 127.59, 125.80, 125.66, 123.05, 122.64, 41.40, 40.49, 26.16, and 25.54; IR (KBr) νmax/cm-1 1610, 1460, 1311, 1149, 1090, 746, and 690; MS (EI) m/z (rel. intensity) 296 (M+, 5), 268 (100), 127 (21), and 77 (9).
6-Bromo-2-phenylsulfonyl-1,4-dihydro-1,4-ethanonaphthalene and 7-bromo-2-phenylsulfonyl-1,4-dihydro-1,4-ethanonaphthalene (12b): Yield, 95%; colorless powder, mp 191−193 °C, Rf = 0.3 (30% EtOAc/hexane); 1H NMR (diastereomer mixture) δ 7.81 (2H, m), 7.47−7.61(4H, m), 7.10−7.32 (2H, m), 7.04 (one isomer, 1H, m), 6.82 (another isomer, 1H, m), 4.17 (2H, m), and 1.46−1.61 (4H, m); 13C NMR (typical signals) δ 147.06, 144.40, 142.87, 143.82, 143.60, 140.78, 140.43, 139.39, 133.45, 133.36, 129.23, 129.19, 128.88, 128.71, 127.79, 126.44, 125.98, 124.71, 124.23, 119.83, 119.46, 41.14, 40.95, 40.26, 40.07, 25.89, and 25.26; IR (KBr) νmax/cm-1 1606, 1471, 1411, 1300, 1242, 1155, and 1090; MS (EI) m/z (rel. intensity) 376 [M+(81Br), 39], 348 (100), 267 (8), 235 (35), 154 (51), and 126 (97). Anal. Calcd for C18H15BrO2S: C, 57.61; H, 4.03. Found: C, 57.31; H, 3.97%.
6-Iodo-2-phenylsulfonyl-1,4-dihydro-1,4-ethanonaphthalene and 7-iodo-2-phenylsulfonyl-1,4-dihydro-1,4-ethanonaphthalene (12c): Yield, 88%, colorless solid, mp 179−185 °C; Rf = 0.25 (CHCl3); 1H NMR (diastereomer mixture) δ 7.80 (1H, m), 7.60 (1H, m), 7.34−7.53 (4H, m), 7.30 (1H, m), 6.92 (one isomer: 1H, d, J = 7.8 Hz), 6.70 (another isomer: 1H, d, J = 7.8 Hz), 4.15 (2H, m), and 1.44−1.55 (4H, m); 13C NMR (typical signals) δ 147.44, 147.08, 144.40, 144.12, 143.88, 143.86, 141.47, 141.21, 139.42, 134.95, 134.78, 133.47, 133.37, 132.18, 131.73, 129.24, 129.20, 127.82, 127.80, 125.09, 124.61, 91.05, 90.81, 41.03, 40.97, 40.16, 40.09, 25.88, 25.83, 25.29, and 25.21; IR (KBr) νmax/cm-1 3061, 1468, 1444, 1404, 1230, 1228, 1146, and 1088; MS (EI) m/z (rel. intensity) 422 (M +, 46), 394 (100), 281 (22), 153 (21), and 126 (37). Anal. Calcd for C18H15IO2S: C, 51.20; H, 3.58. Found: C, 50.88; H, 3.66%.
General procedure for Barton-Zard reaction of 12: To a stirred solution of 12 (5.00 mol) and ethyl isocyanoacetate (0.66 mL, 6.0 mmol) in dry THF (50 mL) was added a 1.0-M solution of potassium tert-butoxide in THF (6.0 mmol) at 0 °C under N2. The mixture was stirred for 8 h at room temperature. The reaction was quenched by adding a 1.0-M aqueous HCl solution. The mixture was extracted with EtOAc. The organic extract was washed successively with aqueous NaHCO3, water and brine, dried over Na2SO4, and concentrated under a reduced pressure. The residue was chromatographed on silica gel to give 13.
Ethyl 4,9-dihydro-4,9-ethano-2H-benzo[f]isoindole-1-carboxylate (13a): Yield, 85%; colorless powder, mp 142−144 °C, Rf = 0.3 (30% EtOAc/hexane); 1H NMR δ 8.81 (1H, br s), 7.25 (1H, m), 7.15 (1H, m), 7.03 (2H, m), 6.56 (1H, d, J = 2.4 Hz), 4.77 (1H, m), 4.31 (2H, q, J = 7.1 Hz), 4.22 (1H, m), 1.69 (4H, m), and 1.35 (3H, q, J = 7.1 Hz); 13C NMR δ 161.57, 144.84, 144.08, 135.50, 130.32, 125.27, 125.20, 123.44, 122.86, 114.49, 113.79, 59.86, 37.47, 37.30, 27.87, 27.24, and 14.52; IR (KBr) νmax/cm-1 3327, 1678, 1423, 1317, 1151, 1088, and 1043; MS (EI) m/z (rel. intensity) 296 (M+, 5), 268 (100), 127 (21), and 77 (9). Anal. Calcd for C17H17NO2+1/8H2O: C, 75.74; H, 6.45; N, 5.20. Found: C, 75.67; H, 6.44; N, 5.14%.
Ethyl 6-bromo-4,9-dihydro-4,9-ethano-2H-benzo[f]isoindole-1-carboxylate and ethyl 7-bromo-4,9-dihydro-4,9-ethano-2H-benzo[f]isoindole-1-carboxylate (13b): Yield, 88%, colorless powder, mp 55−60 °C, Rf = 0.45 (30% EtOAc/hexane); 1H NMR (diastereomer mixture) δ 8.49 (1H, br s), 7.71 (1H, s), 7.21 (1H, m), 7.07 (1H, m), 6.66 (1H, s), 4.74 (1H, s), 4.34 (2H, q, J = 7.1 Hz), 4.26 (1H, s), 1.64−1.72 (4H, m), and 1.40 (3H, t, J = 7.1 Hz); 13C NMR (typical signals) δ 161.25, 147.14, 146.46, 143.96, 143.23, 130.0, 129.64, 128.11, 1,28.05, 126.70, 126.17, 125.12, 124.54, 118.80, 118.72, 114.80, 114.73, 113.92, 113.86, 60.02, 37.28, 37.27, 37.22, 37.21, 27.62, 27.61, 27.01, 27.00, and 14.54; IR (KBr) νmax/cm-1 3313, 1684, 1315, 114.2, and 1041; MS (FAB) m/z 348 [M+(81Br)+1]. Anal. Calcd for C17H16BrNO2+1/ 4CHCl3: C, 56.38; H, 4.53; N, 3.81. Found: C, 56.58; H, 4.46; N, 3.91%.
Ethyl 6-iodo-4,9-dihydro-4,9-ethano-2H-benzo[f]isoindole-1-carboxylate and ethyl 7-iodo-4,9-dihydro-4,9-ethano-2H-benzo[f]isoindole-1-carboxylate (13c): Yield, 90%; colorless oil, Rf = 0.3 (CHCl3); 1H NMR (diastereomer mixture) δ 8.42 (1H, br s), 7.57 (1H, m), 7.32 (1H, m), 6.94−7.01 (1H, m), 6.65 (1H, m), 4.75 (1H, m), 4.33 (1H, m), 4.23 (1H, m), 1.70 (4H, m), and 1.40 (3H, m); 13C NMR (typical signals) δ 161.38, 161.35, 146.52, 144.46, 143.71, 133.97, 133.90, 132.15, 131.66, 129.60, 129.29, 125.32, 124.77, 123.31, 122.75, 114.50, 114.46, 113.96, 90.22, 90.11, 59.91, 59.87, 37.31, 37.23, 36.84, 36.78, 27.45, 26.87, 26.83, and 14.46; IR (KBr) νmax/cm-1 3296, 1684, 1423, 1315, 1142, 1097, 1039; MS (FAB) m/z 394 (M++1).
General procedure for removal of ester moiety: To a solution of 13 (4.00 mmol) in ethylene glycol (40 mL) was added potassium hydroxide (2.0 g) under N2. After being heated at 175 °C for 2 h, the mixture was cooled to room temperature and water was added. The mixture was extracted with ethyl acetate. The organic extract was washed with saturated aqueous NaHCO3 and brine, dried over Na2SO4, and concentrated under a reduced pressure. The residue was chromatographed on silica gel to give 14.
4,9-Dihydro-4,9-ethano-2H-benz[f]isoindole (14a): Yield, 79%; colorless powder, mp 182−184 °C, Rf = 0.35 (30% EtOAc/hexane); 1H NMR δ 7.53 (1H, br s), 7.20 (2H, m), 7.05 (2H, m), 6.53 (2H, d, J = 2.0 Hz), 4.26 (2H, s), and 1.73 (4H, m); 13C NMR δ 145.59, 128.53, 125.19, 123.05, 108.73, 37.46, and 28.43; IR (KBr) νmax /cm-1 3369, 1468, 1396, 1282, 1038, and 750; MS (EI) m/z (rel. intensity) 295 (M+, 33) and 167 (100).
6-Bromo-4,9-dihydro-4,9-ethano-2H-benz[f]isoindole (14b): Yield, 84%; colorless powder, mp 87−89 °C, Rf = 0.5 (30% EtOAc/hexane); 1H NMR δ 7.58 (1H, br s), 7.35 (1H, d, J = 2.0 Hz), 7.18 (1H, dd, J = 7.8 and 2.0 Hz), 7.07 (1H, d, J = 7.8 Hz), 4.24 (1H, s), 4.23 (1H, s), and 1.68−1.76 (4H, m); 13C NMR δ 147.77, 144.56, 127.90, 127.62, 126.29, 124.66, 118.58, 108.99, 108.92, 37.29, 36.96, 28.12, 28.07, and one carbon signal is not found; IR (KBr) νmax /cm-1 3351, 1454, 1408, 1306, 1157, and 1047; MS (FAB) m/z 276 [M+(81Br)+1]. Anal. Calcd for C14H12BrN+1/8CH2Cl2: C, 59.70; H, 4.28; N, 4.84. Found: C, 59.51; H, 4.43, N, 4.80%.
6-Iodo-4,9-dihydro-4,9-ethano-2H-benz[f]isoindole (14c): Yield, 82%; colorless powder, Rf = 0.45 (60% CHCl3/hexane); mp 167−170 °C; 1H NMR δ 7.57 (1H, br s), 7.54 (1H, s), 7.38 (1H, dd, J = 7.8 and 1.5 Hz), 6.96 (1H, d, J = 7.8 Hz), 6.52 (2H, d, J = 1.5 Hz), 4.22 (2H, d, J = 5.4 Hz), and 1.68−1.76 (4H, m); 13C NMR δ 148.12, 145.32, 134.07, 132.03, 127.91, 127.64, 125.11, 108.99, 90.12, 37.15, 37.07, 28.09, and two carbon signals are not found; IR (KBr) νmax/cm-1 3353, 1400, 1305, 1170, 1171, and 1047; MS (FAB) m/z 322 (M++1). Anal. Calcd for C14H12IN+1/8CH2Cl2: C, 51.13; H, 3.72; N, 4.22. Found: C, 51.50; H, 3.69; N, 4.29%.
General procedure for inverse [3+1] porphyrin synthesis: To a solution of pyrrole 14 (1.00 mmol) and tripyrrane 15 (478 mg, 1.00 mmol) in dry CH2Cl2 (100 mL) was added TFA (5.0 mL) at room temperature under N2 in the dark. After being stirred for 20 h at the same temperature, the mixture was neutralized with triethylamine and treated with DDQ (227 mg, 1.00 mmol) for 4 h. To the mixture was added Zn(OAc)2·2H2O (329 mg, 1.50 mmol) and stirred for 6 h. Water was added to the reaction mixture. The mixture was extract with CH2Cl2. The organic extract was washed with water and brine, dried over Na2SO4, and concentrated under a reduced pressure. The residue was purified by column chromatographed on silica gel and recrystallized from CHCl3/MeOH to give 1.
8,17-Dibutyl-12,13-diethyl-7,18-dimethyl-21,26-dihydro-21,26-ethanonaphtho[2,3-b]porphyrinato-zinc (1a): Yield, 38%; red powder, mp 232 °C (decomp), Rf = 0.35 (10% EtOAc/hexane); UV-vis (CHCl3) λmax/nm (log10 ε): 402 (5.49), 531 (4.21), 569 (4.28); 1H NMR δ 9.94 (2H, s), 8.48 (2H, s), 7.92 (2H, m), 7.33 (2H, m), 6.15 (2H, s), 3.38 (6H, s), 3.32 (4H, t, J = 7.6 Hz), 2.46 (2H, d, J = 6.8 Hz), 2.29 (2H, d, J = 6.8 Hz), 1.85 (4H, m), 1.47 (4H, m), 1.35 (6H, t, J = 7.6 Hz), 0.96 (6H, t, J = 7.6 Hz); 13C NMR δ 148.02, 147.27, 146.47, 146.40, 145.51, 141.33, 140.65, 140.33, 135.20, 125.44, 123.88, 97.32, 95.57, 40.55, 35.27, 29.37, 25.61, 23.00, 19.15, 18.38, 14.29, 11.72; MS (FAB) m/z 697 (M++1). Anal. Calcd for C44H48N4Zn: C, 75.68; H, 6.93; N, 8.02. Found: C, 75.61; H, 7.19; N, 8.00%.
23-Bromo-8,17-dibutyl-12,13-diethyl-7,18-dimethyl-21,26-dihydro-21,26-ethanonaphtho[2,3-b]porphyrinato zinc (1b): Yield, 43%; red solid, mp 238 °C (decomp), Rf = 0.2 (15% EtOAc/hexane); UV-vis (CHCl3) λmax/nm (log10 ε): 402 (5.55), 531 (4.26), and 570 (4.29); 1H NMR δ 10.12 (1H, s), 10.10 (1H, s), 9.40 (2H, s), 8.21 (1H, s), 7.61 (2H, s), 6.08 (1H, s), 6.07 (1H, s), 3.30−3.59 (14H, m), 2.59 (2H, d, J = 8.3 Hz), 2.33 (2H, d, J = 8.3 Hz), 2.02 (2H, m), 1.62 (4H, m), 1.54 (6H, t, J = 7.3 Hz), and 1.05 (6H, t, J = 7.3 Hz); 13C NMR (typical signals) δ 148.75, 147.72, 147.53, 147.51, 147.26, 147.00, 146.10, 145.52, 146.10, 141.11, 140.73, 135.64, 135.59, 128.17, 127.11, 125.37, 118.90, 97.46, 95.96, 40.22, 39.95, 35.20, 28.89, 25.70, 22.91, 19.21, 18.28, 14.15, 11.68, and 11.65; MS (FAB) m/z 823 (M++1).
8,17-Dibutyl-12,13-diethyl-23-iodo-7,18-dimethyl-21,26-dihydro-21,26-ethanonaphtho[2,3-b]porphyrinato zinc (1c): Yield, 41%; red solid, mp 233 °C (decomp), Rf = 0.2 (15% EtOAc/hexane); UV-vis (CHCl3) λmax/nm (log10 ε): 402 (5.55), 531 (4.26), and 570 (4.29); 1H NMR δ 10.12 (1H, s), 10.10 (1H, s), 9.40 (2H, s), 8.21 (1H, s), 7.61 (2H, s), 6.08 (1H, s), 6.07 (1H, s), 3.30−3.59 (14H, m), 2.59 (2H, d, J = 8.3 Hz), 2.33 (2H, d, J = 8.3 Hz), 2.02 (2H, m), 1.62 (4H, m), 1.54 (6H, t, J = 7.3 Hz), and 1.05 (6H, t, J = 7.3 Hz); 13C NMR (typical signals) δ 149.27, 147.51, 147.20, 147.08, 146.42, 145.47, 140.95, 140.61, 140.37, 135.24, 135.21, 134.36, 132.96, 125.93, 97.24, 95.44, 90.40, 40.13, 40.10, 35.16, 28.96, 28.92, 25.47, 22.91, 19.02, 18.26, 14.21, 11.64, and 11.59. MS (FAB) m/z 823 (M++1). Anal. Calcd for C44H47IN4Zn C, 64.12; H, 5.75; N, 6.80. Found: C, 64.05; H, 5.80; N, 6.71%.
8,17-Dibutyl-12,13-diethyl-7,18-dimethyl-23-trimethylsilylethynyl-21,26-dihydro-21,26-ethanonaphtha[2,3-b]porphyrinato zinc (1d): Three-times freeze-pump-thaw cycles were performed for a solution of porphyrin 1c (196 mg, 0.238 mmol), CuI (I) (4.5 mg, 0.024 mmol), triphenylphosphine (6.2 mg, 0.024 mmol), dichlorobis(tripheylphosphine)palladium(II) (16.8 mg, 0.0239 mmol), trimethysilylacetylene (0.34 mL, 2.4 mmol), and diisopropylamine (3.0 mL) in dry THF (30 mL) to remove dissolved O2 and then the solution was filled by N2. The mixture was refluxed for 12 h. The mixture was cooled to room temperature and water was added. The mixture was extracted with CHCl3. The organic extract was washed with water and brine, dried over Na2SO4, and concentrated under a reduced pressure. The residue was purified by column chromatography on silica gel and recrystallized from CHCl3/MeOH to give 1d (177 mg, 0.223 mmol, 94%) as a red solid, mp 166−172 °C; Rf = 0.3 (30% EtOAc/hexane), UV-vis (CHCl3) λmax/nm (log10 ε) 402 (5.53), 531 (4.21), and 570 (4.23); 1H NMR δ 10.10 (2H, s), 9.39 (1H, s), 9.33 (1H, s), 7.99 (1H, s), 7.78 (1H, d, J = 7.8 Hz), 7.43 (1H, d, J = 7.3 Hz), 6.10 (2H, d, J = 5.9 Hz), 3.65−3.78 (8H, m), 3.57 (3H, s), 3.55 (3H, s), 2.40 (2H, d, J = 8.3 Hz), 2.27 (2H, d, J = 9.3 Hz), 2.09 (4H, m), 1.62−1.69 (14H, m), 1.05 (6H, q, J = 7.3 Hz), and 0.26 (9H, s); 13C NMR (typical signals) δ147.76, 147.73, 147.68, 147.59, 147.39, 147.33, 146.94, 146.56, 146.53, 146.20, 141.54, 141.31, 140.95, 140.90, 135.87, 135.81, 129.27, 127.28, 123.59, 119.91, 105.87, 97.57, 96.36, 96.30, 92.79, 40.19, 40.04, 35.22, 35.19, 28.86, 28.82, 25.85, 25.79, 22.90, 22.86, 19.36, 18.30, 14.10, 14.08, and 11.62; MS (FAB) m/z 793 (M++1). Anal. Calcd for C49H56N4SiZn: C, 74.08; H, 7.10; N, 7.05. Found: C, 73.68; H, 7.15; N, 6.88%.
8,17-Dibutyl-12,13-diethyl-7,18-dimethyl-23-(4,4,5,5-tetramethyl-1,3,2-dioxaborolan-2-yl)-21,26-dihydro-21,26-ethanonaphtho[2,3-b]porphyrinato zinc (1e): A solution of halogenated porphyrins 1b or 1c (0.200 mmol), KOAc (196 mg, 2.00 mmol), PdCl2(PPh3)2 (14 mg, 0.02 mmol) and bis(pinacolato)diboron (254 mg, 1.00 mmol) in DMSO (5.0 mL) was filled with N2 by freeze-pump-thaw cycles three times. The mixture was heated at 100 °C for 15 h. The mixture was cooled to room temperature and water was added. The mixture was extracted with CHCl3. The organic extract was washed with water and brine, dried over Na2SO4, and concentrated under a reduced pressure. The residue was purified by column chromatography on silica gel and recrystallized from CHCl3/MeOH to give 1e (73% and 75% form 1b and 1c, respectively) as a red powder, mp >250 °C; Rf = 0.15 (10% EtOAc/hexane); UV-vis (CHCl3) λmax/nm (log10 ε): 402 (5.52), 531 (4.20), and 559 (4.27); 1H NMR δ 10.01 (1H, s), 9.93 (1H, s), 8.70 (1H, s), 8.48 (1H, s), 8.41 (1H, s), 7.99 (1H, d, J = 7.3 Hz), 7.87 (1H, d, J = 7.3 Hz), 6.17 (2H, m), 3.53 (3H, s), 3.42 (3H, s), 3.17−3.40 (8H, m), 2.45 (2H, m), 2.30 (2H, m), 1.96 (2H, m), 1.86 (2H, m), 1.55 (2H, m), 1.44 (2H, m), 1.37 (6H, s), 1.36 (12H, s), 0.99 (3H, t, J = 7.3 Hz), and 0.93 (3H, t, J = 7.3 Hz); 13C NMR δ 150.04, 148.23, 147.43, 147.36, 147.19, 146.63, 146.38, 145.84, 145.60, 145.49, 141.27, 141.26, 140.69, 140.54, 140.36, 135.38, 135.18, 132.54, 129.87, 123.51, 97.38, 97.28, 95.70, 95.52, 83.71, 40.69, 40.34, 35.32, 35.19, 29.14, 29.13, 25.82, 25.56, 25.04, 24.95, 23.02, 22.91, 19.16, 19.10, 18.34, 18.30, 14.29, 14.23, 11.78, and 11.71; MS (FAB) m/z 823 (M++1). Anal. Calcd for C50H59BN4O2Zn+CH2Cl2: C, 67.37; H, 6.76; N, 6.16. Found: C, 67.60; H, 6.83; N, 6.50%.
8,17-Dibutyl-12,13-diethyl-23-ethynyl-7,18-dimethyl-21,26-dihydro-21,26-ethanonaphtho[2,3-b]porphyrinato zinc (1f): To a stirred solution of porphyrin 1d (177 mg, 0.223 mmol) in THF (23 mL) was added 1.0-M tetrabutylammonium fluoride in THF (2.3 mL, 2.3 mmol) at 0 °C. The mixture was stirred at the same temperature for 2 h. Water was added to the reaction. The mixture was extract with EtOAc. The organic extract was washed with water and brine, dried over Na2SO4, and concentrated under a reduced pressure. The residue was purified by recrystallization from CHCl3/MeOH to give 1f (141 mg, 0.195 mmol, 87%) as a red solid, mp 225 °C (decomp.); UV-vis (CHCl3) λmax/nm (log10 ε): 402 (5.51), 531 (4.20), and 570 (4.27); 1H NMR δ 9.91 (1H, s), 9.87 (1H, s), 8.53 (1H, s), 8.49 (1H, s), 7.78 (1H, d, J = 6.4 Hz), 7.87 (1H, m), 7.51 (1H, m), 6.14 (2H, s), 3.41 (3H, s), 3.35 (3H, s), 3.25−3.34 (8H, m), 3.08 (1H, s), 2.48 (2H, m), 2.32 (2H, m), 1.87 (4H, m), 1.50 (4H, m), 1.37 (6H, m), and 0.97 (6H, m); 13C NMR (typical signals) δ 147.55, 147.53, 147.43, 147.30, 147.27, 147.21, 146.63, 146.61, 146.54, 145.74, 141.01, 140.98, 140.84, 140.82, 140.46, 140.39, 135.32, 135.30, 135.28, 129.62, 127.50, 123.82, 119.01, 97.23, 95.59, 84.40, 762.17, 57.51, 57.45, 40.31, 40.16, 35.23, 35.09, 29.96, 25.51, 25.44, 23.10, 22.85, 19.03, 18.17, 14.09, 13.30, 11.54, and 11.45; MS (MALDI-TOF) m/z 720.2 (M+).
Suzuki-Miyaura reaction giving 2: A solution of halogenated porphyrin 1b or 1c (0.0400 mmol) pinacolatoboronated porphyrin 1d (0.0400 mmol), K2CO3 (27.6 mg, 0.0800 mmol), and PdCl2(PPh3)2 (2.8 mg, 0.0040 mmol) in DMSO (0.5 mL) was degassed by three-times freeze-pump-thaw cycles and then filled with N2. The mixture was heated at 100 °C for 14 h. The mixture was cooled to room temperature and water was added. The mixture was extracted with CHCl3. The organic extract was washed with water and brine, dried over Na2SO4, and concentrated under a reduced pressure. The residue was purified by column chromatography on silica gel and recrystallized from CHCl3/MeOH to give bi(21,23-zinco-8,17-dibutyl-12,13-diethyl-7,18-dimethyl-21,26-dihydro-21,26-ethanonaphtho[2,3-b]porphyrin-23-yl) (2; 88% and 83% form 1b and 1c, respectively) as a red powder, mp >300 °C; Rf = 0.95 (CHCl3); UV-vis (CHCl3) λmax/nm (log10 ε): 402 (5.73), 531 (4.47), and 569 (4.55); 1H NMR (pyridine-d5) δ 10.95 (2H, s), 10.85 (2H, s), 10.52 (4H, s), 8.12 (2H, m), 7.87 (2H, s), 6.50 (2H, m), 6.41 (2H, s), 4.19 (16H, m), 3.85 (6H, s), 3.78 (6H, s), 2.37 (12H, m), 2.22 (4H, m), 1.96 (6H, m), 1.77 (8H, m), and 1.09 (12H, m); 13C NMR (pyridine-d5; typical signals) δ 149.25, 149.21, 149.12, 148.46, 147.54, 147. 51, 145.73, 145.68, 143.03, 142.99, 142.97, 142.88, 141.99, 141.96, 139.40, 139.33.137.06, 124.55, 124.48, 124.44, 98.79, 98.74, 97.49, 97.48, 40.96, 40.40, 35.91, 29.75, 29.72, 26.62, 23.30, 20.24, 19.06, 14.45, 12.08, 12.05, and 12.00; MS (MALDI-TOF) m/z 1394.8 (M++H).
Sonogashira reaction giving 3: Three-times freeze-pump-thaw cycles were applied for a solution of 1f (78.1 mg, 0.108 mmol), 1c (89.0 mg, 0.108 mmol), CuI (I) (2.1 mg, 0.011 mmol), triphenylphosphine (2.9 mg, 0.011 mmol), dichlorobis(tripheylphosphino)palladium(II) (7.0 mg, 0.010 mmol), and diisopropylamine (0.5 mL) in dry THF (5.0 mL) to remove dissolved O2 and then the mixture was filled with N2. The mixture was refluxed for 14 h. After the mixture was cooled to room temperature and water was added. The mixture was extracted with EtOAc. The organic extract was washed with water and brine, dried over Na2SO4, and concentrated under a reduced pressure. The residue was purified by column chromatography on silica gel and recrystallized from CHCl3/MeOH to give 1,2-di(21,23-zinco-8,17-dibutyl-12,13-diethyl-7,18-dimethyl-21,26-dihydro-21,26-ethanonaphtho[2,3-b]porphyrin-23-yl)ethyne (3; 67.4 mg, 0,0424 mmol, 88%) as a red powder, mp >300 °C; Rf = 0.95 (CHCl3); UV-vis (CHCl3) λmax/nm (log10 ε): 403 (5.73), 532 (4.47), and 570 (4.53); 1H NMR (THF-d8) δ 10.29 (2H, s), 10.27 (2H, s), 10.08 (4H, s), 7.88 (2H, s), 7.68 (2H, d, J = 7.6 Hz), 7.32 (2H, d, J = 7.6 Hz), 6.12 (4H, s), 4.09 (16H, m), 3.67 (6H, s), 3.66 (6H, s), 2.41 (4H, m), 2.29 (8H, m), 2.13 (4H, m), 1.88 (6H, t, J = 7.6 Hz), 1.72 (8H, m), and 1.06 (12H, t, J = 7.3 Hz); 13C NMR (THF-d8) δ 150.81, 150.77, 150.75, 150.72, 150.70, 150.32, 150.10, 149.42, 144.46, 144.34, 144.33, 143.41, 138.53, 138.47, 132.34, 129.84, 126.23, 121.05, 118.51, 100.06, 100.00, 98.92, 84.14, 75.37, 42.83, 42.59, 38.14, 31.38, 28.59, 25.43, 25.42, 25.41, 22.12, 22.10, 20.78, 20.76, 16.21, 13.49, and 13.48; MS (MALDI-TOF) m/z 1419.8 (M+).
Eglinton modification of the Glaser coupling reaction giving 4: Porphyrin 1f (66.1 mg, 0.0915 mmol) and Cu(OAc)2 (33 mg, 177 mmol) were dissolved in 5 mL of pyridine and the mixture was stirred at room temperature for 12 h. Water was added and the resulting mixture was extract with THF. The organic extract was washed with water and brine, dried over Na2SO4, and concentrated under a reduced pressure. The residue was purified by recrystallization from CHCl3/MeOH to give 1,4-di(21,23- zinco-8,17-dibutyl-12,13-diethyl-7,18-dimethyl-21,26-dihydro-21,26-ethanonaphtho[2,3-b]porphyrin-23-yl)-1,3-butadiyne (4; 61.6 mg, 0.0427 mmol, 93%) as a red powder, mp >300 °C; Rf = 0.95 (CHCl3); UV-vis (CHCl3) λmax/nm (log10 ε) 403 (5.83), 532 (4.52), and 570 (4.59); 1H NMR (THF-d8) δ 10.28 (2H, s), 10.27 (2H, s), 10.08 (4H, s), 7.88 (2H, s), 7.70 (2H, d, J = 7.8 Hz), 7.35 (2H, d, J = 7.8 Hz), 6.13 (4H, s), 4.10 (16H, m), 3.66 (12H, s), 2.40 (4H, m), 2.27 (8H, m), 2.11 (4H, m), 1.89 (6H, m), 1.70 (12H, t, J = 7.6 Hz), and 1.06 (12H, t, J = 7.4 Hz); 13C NMR (THF-d8) δ 147.85, 147.81, 147.73, 147.70, 147.56, 147.46, 147.08, 146.29, 146.28, 146.18, 141.48, 141.46, 141.45, 141.43, 140.44, 140.41, 135.52, 135.48, 128.07, 126.18, 123.02, 120.04, 97.05, 97.03, 95.95, 95.93, 88.39, 39.80, 39.78, 39.73, 35.17, 28.56, 28.54, 28.52, 25.63, 22.47, 22.45, 19.16, 17.81, 13.24, 10.53, and 10.52; MS (MALDI-TOF) m/z 1442.7 (M++H).
General procedure for the preparative retro-Diels-Alder reaction: A sample tube containing appropriate bicyclo[2.2.2]octadiene-fused porphyrin (ca. 10 mg) was placed in a glass flask. The flask was evacuated by means of a rotary vacuum pump and then placed in a preheated tube oven at 290 °C. After 1 h, the flask was taken out and then flushed with N2. The obtained sample was subject to all analysis. The conversion was quantitative.
8,17-Dibutyl-12,13-diethyl-7,18-dimethylnaphtho[2,3-b]porphyrinato zinc (8a): Green powder, mp >300 °C; UV-vis (pyridine) λmax/nm (log10 ε): 337 (4.30), 437 (5.48), 572 (4.30), 593 (4.44), and 610 (4.60). 1H NMR (pyridine-d5; typical signals) δ 10.90 (2H, s), 10.45 (2H, s), 7.85 (2H, m), 4.16 (8H, m), 3.73 (6H, s), 2.36 (4H, s), 1.95 (6H, m), 1.76 (4H, m), and 1.09 (6H, t, J = 8.6 Hz); 13C NMR (pyridine-d5; typical signals) δ 147.49, 146.98, 146.78, 142.51, 142.01, 133.50, 129.77, 126.27, 120.16, 99.43, 94.34, 35.92, 26.60, 23.34, 20.18, 19.13, 14.47, and 11.96; MS (FAB) m/z 669 (M++1). Anal. Calcd for C42H44N4Zn: C, 75.27; H, 6.62; N, 8.36. Found: C, 75.47; H, 6.91; N, 8.32%.
23-Bromo-8,17-dibutyl-12,13-diethyl-7,18-dimethylnaphtho[2,3-b]porphyrinato zinc (8b): Brown solid, mp 228-232 °C; UV-vis (pyridine) λmax/nm (log10 ε) 341 (4.53), 439 (5.45), 572 (4.31), 595 (4.48), and 609 (4.60); 1H NMR (pyridine-d5) δ 10.82 (2H, s), 10.42 (2H, s), 10.21 (1H, s), 10.17 (1H, s), 8.86 (1H, s), 7.76 (1H, d, J = 8.8 Hz), 7.89 (1H, d, J = 8.8 Hz), 4.13 (8H, m), 3.69 (3H, s), 3.68 (3H, s), 2.33 (4H, m), 1.91 (6H, t, J = 7.3 Hz), 1.74 (4H, m), and 1.07 (6H, t, J = 7.3 Hz); 13C NMR (pridine-d5; typical signals) δ 150.43, 150.38, 147.68, 147.66, 147.18, 147.16, 146.24, 146.09, 142.57, 142.16, 139.67, 139.24, 134.22, 131.53, 131.50, 129.22, 123.99, 120.29, 119.91, 119.20, 99.50, 99.45, 94.53, 94.49, 35.91, 26.60, 23.35, 20.17, 19.11, 14.47, 11.95, and 11.92; MS (FAB) m/z 749 [M+(81Br)+1]. Anal. Calcd for C42H43BrN4Zn: C, 67.43; H, 5.79; N, 7.48. Found: C, 67.29; H, 5.82; N, 7.48%.
8,17-Dibutyl-12,13-diethyl-23-iodo-7,18-dimethylnaphtho[2,3-b]porphyrinato zinc (8c): Mp >250 °C; UV-vis (pyridine) λmax/nm (log10 ε) 341 (4.59), 416 (5.05), 439 (5.47), 572 (4.35), 594 (4.53), and 610 (4.60); 1H NMR (pyridine-d5) δ 10.86 (2H, s), 10.46 (2H, s), 10.23 (1H, s), 10.15 (1H, s), 9.14 (1H, s), 8.40 (1H, d, J = 8.8 Hz), 8.11 (1H, d, J = 8.8 Hz), 4.14−4.19 (8H, m), 3.73 (6H, s), 2.39 (4H, m), 1.96 (6H, m), 1.76 (4H, t, J = 7.6 Hz), and 1.11 (6H, t, J = 7.3 Hz); 13C NMR (pyridine-d5) δ 147.67, 147.16, 146.56, 146.19, 142.56, 142.15, 139.39, 139.24, 138.36, 134.78, 134.37, 131.72, 131.26, 120.26, 119.02, 99.46, 94.50, 92.27, 35.91, 26.60, 23.34, 20.17, 19.11, 14.47, 12.95, and 11.92; MS (FAB) m/z 795 (M++1). Anal. Calcd for C42H43IN4Zn: C, 63.36; H, 5.44; N, 7.07. Found: C, 63.59; H, 5.44; N, 7.01%.
8,17-Dibutyl-12,13-diethyl-23-trimethylsilylethynyl-7,18-dimethylnaphtho[2,3-b]porphyrinato zinc (8d): Dark blue solid; mp >250 °C; UV-vis (pyridine) λmax/nm (log10 ε): 347 (4.59), 442 (5.42), 574 (4.35), 597 (4.58), and 611 (4.64); 1H NMR δ 9.27 (2H, s), 8.41 (4H, m), 8.41 (1H, m), 8.05 (1H, d, J = 8.8 H), 7.75 (1H, d, J = 8.8 Hz), 3.86 (4H, t, J = 7.6 Hz), 3.57 (4H, m), 2.86 (3H, s), 2.84 (3H, s), 2.05 (4H, m), 1.86 (6H, m), 1.67, (4H, m), 1.10 (6H, t, J = 7.3 Hz), and 0.53 (9H, s); 13C NMR δ 14.73, 147.70, 145.45, 145.44, 143.52, 143.48, 140.95, 140.91, 140.73, 136.57, 136.34, 133.62, 133.58, 133.31, 131.13, 128.73, 127.70, 119.58, 117.62, 117.52, 106.49, 98.24, 94.86, 91.98, 91.81, 35.27, 25.74, 25.71, 23.09, 19.62, 18.66, 14.22, 10.81, 10.75, and 0.34; MS (FAB) m/z 765 (M++1). Anal. Calcd for C49H56N4SiZn: C, 73.65; H, 6.84; N, 7.31. Found: C, 73.98; H, 6.89; N, 7.27%.
Bi(8,17-dibutyl-12,13-diethyl-7,18-dimethyl-21,23-zinconaphtho[2,3-b]porphyrin-23-yl) (5): Green powder, mp >300 °C; UV-vis (pyridne) λmax/nm (log10 ε): 355 (4.80), 427 (5.29), 448 (5.52), 558 (4.41), 575 (4.65), 599 (4.97), and 611 (4.88); 1H NMR (pyridine-d5; typical signals) δ 10.95 (2H, s), 10.91 (2H, s), 10.91 (2H, s), 10.48 (2H, s), 10.45 (4H, s), 10.38 (2H, s), 9.41 (2H, s); 8.90 (2H, d, J = 8.6 Hz), 3.86 (6H, s), 3.83 (6H, s), 2.47 (8H, m), 2.03 (12H, t, J = 7.6 Hz), 1.88 (8H, m), and 1.20 (12H, t, J = 7.3 Hz); MS (MALDI-TOF) m/z 1336.7 (M+).
Di(8,17-dibutyl-12,13-diethyl-7,18-dimethyl-21,23-zinconaphtho[2,3-b]porphyrin-23-yl)ethyne (6): Green powder, mp >300 °C; UV-vis (pyridne) λmax/nm (log10 ε) 356 (4.80), 426 (sh, 5.29), 446 (5.47), 559 (4.43), 575 (4.61), 600 (4.89), and 611 (4.88); 1H NMR (pyridine-d5) δ 10.87 (2H, s), 10.85 (2H, s), 10.43 (4H, s), 10.32 (2H, s), 10.26 (2H, s), 9.19 (2H, s), 8.77 (2H, d, J = 8.4 Hz), 8.24 (2H, d, J = 8.4 Hz), 4.23 (16H, m), 3.82 (6H, s), 3.77 (6H, s), 2.48 (8H, m), 2.04 (12H, t, J = 7.6 Hz), 1.88 (8H, m), and 1.21 (12H, t, J = 7.3 Hz); MS (MALDI-TOF) m/z 1359.8 (M++1).
Di(8,17-dibutyl-12,13-diethyl-7,18-dimethyl-21,23-zinconaphtho[2,3-b]porphyrin-23-yl)-1,3-butadiyne (7): Green powder, mp >300 °C; UV-vis (pyridne) λmax/nm (log10 ε) 356 (4.88), 425 (5.32), 547 (4.19), 560 (4.48), 575 (4.67), 601 (4.96), and 612 (4.94); 1H NMR (pyridine-d5; typical signals) δ 10.85 (2H, s), 10.84 (2H, s), 10.44 (4H, s), 10.27 (2H, s), 10.23 (2H, s), 9.11 (2H, s), 8.06 (2H, d, J = 8.8 Hz), 4.22 (16H, m), 3.81 (12H, s), 2.48 (8H, m), 2.04 (12H, t, J = 7.8 Hz), 1.89 (8H, m), and 1.21 (12H, t, J = 7.3 Hz); MS (MALDI-TOF) m/z 1383.6 (M++1).
ACKNOWLEDGEMENTS
This work is partially supported by Grant-in-Aids for the Scientific Research (23350020, 23655044, and 23108714 (π-space)) from the Japanese Ministry of Education, Culture, Sports, Science and Technology. Some calculations are done at IMS under the cooperative research program.
References
1. D. Kim, ed. “Multiporphyrin Arrays, Fundamentals and Applications.” Pan Stanford Publishing Pte. Ltd. 2012. CrossRef
2. K. Tashiro and T. Aida, Chem. Soc. Rev., 2007, 36, 189; CrossRef N. Komatsu and F. Wang, Materials, 2010, 3, 3818; CrossRef T. Yamaguchi, N. Ishii, K. Tashiro, and T. Aida, J. Am. Chem. Soc., 2003, 125, 13934; CrossRef S. Campidelli, C. Sooambar, E. Lozano Diz, C. Ehli, D. M. Guldi, and M. Prato, J. Am. Chem. Soc., 2006, 128, 12544; CrossRef F. Cheng, S. Zhang, A. Adronov, L. Echegoyen, and F. Diederich, Chem. Eur. J., 2006, 12, 6062; CrossRef H. Nobukuni, Y. Shimazaki, F. Tani, and Y. Naruta, Angew. Chem. Int. Ed., 2007, 119, 9133; CrossRef X. Peng, N. Komatsu, S. Bhattacharya, T. Shimawaki, S. Aonuma, T. Kimura, and A. Osuka, Nature Nanotech., 2007, 2, 361; CrossRef H. Uno, M. Furukawa, A. Fujimoto, H. Uoyama, H. Watanabe, T. Okujima, H. Yamada, S. Mori, M. Kuramoto, T. Iwamura, N. Hatae, F. Tani, and N. Komatsu, J. Porphyrins Phthalocyanines, 2011, 15, 951. CrossRef
3. M.-C. Yoon, Z. S. Yoon, S. Cho, D. Kim, A. Takagi, T. Matsumoto, T. Kawai, T. Hori, X. Peng, N. Aratani, and A. Osuka, J. Phys. Chem. A, 2007, 111, 9233; CrossRef K. J. McEwan, P. A. Fleitz, J. E. Rogers, J. E. Slagle, D. G. McLean, H. Akdas, M. Katterle, I. M. Blake, and H. L. Anderson, Adv. Mater., 2004, 16, 1933; CrossRef M. Balaz, H. A. Collins, E. Dahlstedt, and H. L. Anderson, Org. Biomol. Chem., 2009, 7, 874. CrossRef
4. H. Uoyama, K. S. Kim, K. Kuroki, J.-Y. Shin, T. Nagata, T. Okujima, H. Yamada, N. Ono, D. Kim, and H. Uno, Chem. Eur. J., 2010, 16, 4063; CrossRef J. A. A. W. Elemans, R. van Hameren, R. J. M. Nolte, and A. E. Rowan, Adv. Mater., 2006, 18, 1251; CrossRef T. Hori, N. Aratani, A. Takagi, T. Matsumoto, T. Kawai, M.-C. Yoon, Z. S. Yoon, S. Cho, D. Kim, and A. Osuka, Chem. Eur. J., 2006, 12, 1319; CrossRef V. S. Lin, S. G. DiMagno, and M. J. Therien, Science, 1994, 264, 1105. CrossRef
5. H. Hata, H. Shinokubo, and A. Osuka, J. Am. Chem. Soc., 2005, 127, 8264; CrossRef K. Funatsu, A. Kimura, T. Imamura, A. Ichimura, and Y. Sasaki, Inorg. Chem., 1997, 36, 1625; CrossRef H. Uno, Y. Kitawaki, and N. Ono, Chem. Commun., 2002, 116. CrossRef
6. D. Holten, D. F. Bocian, and J. S. Lindsey, Acc. Chem. Res., 2002, 35, 57. CrossRef
7. R. Selensky, D. Holten, M. W. Windsor, J. B. Paine III, D. Dolphin, M. Gouterman, and J. C. Thomas, Chem. Phys., 1981, 60, 33. CrossRef
8. T. E. O. Screen, I. M. Blake, L. H. Rees, W. Clegg, S. J. Borwick, and H. L. Anderson, J. Chem. Soc., Perkin Trans. 1, 2002, 320; CrossRef M. K. Kuimova, M. Balaz, H. L. Anderson, and P. R. Ogilby, J. Am. Chem. Soc., 2009, 131 , 7948. CrossRef
9. (a) Y. Nakamura, N. Aratani, and A. Osuka, Chem. Soc. Rev., 2007, 36, 831; CrossRef (b) C.-W. Lee, H.-P. Lu, C.-M. Lan, Y.-L. Huang, Y.-R. Liang, W.-N. Yen, Y.-C. Liu, Y.-S. Lin., E. W.-G. Diau, and C.-Y. Yeh, Chem. Eur. J., 2009, 15, 1403. CrossRef
10. L. J. K. Boerner, M. Nath, M. Pink, and J. M. Zaleski, Chem. Eur. J., 2011, 17, 9311; CrossRef C. Maeda, T. Yoneda, N. Aratani, M.-C. Yoon, J. M. Lim, D. Kim, N. Yoshioka, and A. Osuka, Angew. Chem. Int. Ed., 2011, 50, 5691. CrossRef
11. S. Ito, L. T. Phong, T. Komatsu, N. Igarashi, S. Otsubo, Y. Sakai, A. Ohno, S. Aramaki, Y. Tanaka, H. Uno, T. Oba, and K. Hiratani, Eur. J. Org. Chem., 2009, 5373. CrossRef
12. P. K. Panda, S. Padhy, K.-S. Ha, and C.-H. Lee, Bull. Korean Chem. Soc., 2004, 25, 1421. CrossRef
13. D. Kuzuhara, H. Yamada, T. Okujima, and H. Uno, unpublished result.
14. X.-Z. Jiang, C. Cai, J.-T. Liu, and H. Uno, Org. Biomol. Chem., 2012, 10, 3110; CrossRef C. Cai, H. Uoyama, M. Nakamura, and H. Uno, Heterocycles, 2012, 84, 829. CrossRef
15. S. Ito, N. Ochi, H. Uno, T. Murashima, and N. Ono, Chem. Commun., 2000, 893. CrossRef
16. H. E. Simmons, J. Am. Chem. Soc., 1963, 85, 1657; CrossRef A. M. Braun, J. Org. Chem., 1970, 35, 1208. CrossRef
17. H. Uno, K. Nakamoto, K. Kuroki, A. Fujimoto, and N. Ono, Chem. Eur. J., 2007, 13, 5773; CrossRef S. Ito, K. Nakamoto, H. Uno, T. Murashima, and N. Ono, Chem. Commun., 2001, 2696. CrossRef
18. A. Boudif and M. Momenteau, J. Chem. Soc. Chem. Commun., 1994, 2069; CrossRef A. Boudif and M. Momenteau, J. Chem. Soc. Perkin Trans. 1, 1996, 1235; CrossRef J. L. Sessler, J. W. Genge, A. Urbach, and P. Sanson, Synlett, 1996, 187; CrossRef T. D. Lash, P. Chandrasekar, A. T. Osuma, S. T. Chaney, and J. D. Spence, J. Org. Chem., 1998, 63, 8455. CrossRef
19. J. L. Sessler, M. R. Johnson, and V. Lynch, J. Org. Chem., 1987, 52, 4394; CrossRef S. V. Shevchuk, J. M. Davis, and J. L. Sessler, Tetrahedron Lett., 2001, 42, 2447. CrossRef
20. M. Gouterman, J. Mol. Spectroscopy, 1961, 6, 138. CrossRef