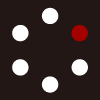
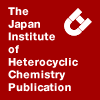
HETEROCYCLES
An International Journal for Reviews and Communications in Heterocyclic ChemistryWeb Edition ISSN: 1881-0942
Published online by The Japan Institute of Heterocyclic Chemistry
e-Journal
Full Text HTML
Received, 27th June, 2012, Accepted, 3rd August, 2012, Published online, 7th August, 2012.
DOI: 10.3987/COM-12-S(N)63
■ SYNTHESIS AND PROPERTIES OF AZULENE-SUBSTITUTED THIOPHENES, TERTHIOPHENES AND DITHIENOTHIOPHENES
Tetsuo Okujima,* Akiko Toda, Yoko Miyashita, Ayumi Nonoshita, Hiroko Yamada, Noboru Ono, and Hidemitsu Uno
Department of Chemistry and Biology, Graduate School or Science and Engineering, Ehime University, Bunkyo-cho 2-5, Matsuyama 790-8577, Japan
Abstract
Bis(azulenylethynyl)thiophenes, terthiophenes, and dithienothiophenes have been synthesized by the Pd-catalyzed cross-coupling of the corresponding diiodothiophenes with 1- and 6-ethynylazulenes under Sonogashira–Hagihara conditions.INTRODUCTION
Various π-conjugated systems based on oligomeric and/or fused aromatics have been studied as large-area, low-cost, and flexible materials such as organic light emitting diodes, organic photovoltaic cells, and organic field effect transistors (OFETs).1 Oligothiophene and thienothiophene derivatives are an important and promising class of organic semiconductors.2 On the other hand, azulene (C10H8), a structural isomer of naphthalene, has attracted the interest of many research groups due to its unusual properties as well as its beautiful blue color.3 Its experimental values of the dipole moment of azulene are reported to 1.0 D and 1.08 D.4 The negative end of the dipole moment vector is toward its five-member ring, which results in cation stabilization.5 Morley has reported that overall ring charges of the azulene ring was in agreement with a large polarized structure of azulene ring. In addition, the high electron density can be found at 1- and 3-positions in azulene ring, whereas the positive charge is localized at 4-, 6-, and 8-positions.6 Recently, azulene-thiophene oligomers, 1,3- and 4,7-di(2-thienyl)azulenes, have been reported as advanced materials for electronic, optelectronic, and electrochromic devices.7 These oligomers provided effective optical band gap control and on/off fluorescence switching. In 2012, Nakayama and co-workers have reported di(2-azulenyl)-2,2'-bithiophene and -thieno[3,2-b]thiophene which showed OFET characteristics with high carrier mobilities of up to 5.0 × 10−2 cm2/Vs.8 These systems exhibited an edge-to-face orientation in a well-defined herringbone packing in the crystalline state. Since 2-azulenyl group acts as neither electron-donating nor electron-withdrawing groups, the electronic contribution for bithiophene and thienothiophene should be smaller than the substitution at the other positions. We report herein the synthesis of di(1- and 6-azulenylethynyl)thiophenes, terthiophenes and dithienothiophenes by Sonogashira–Hagihara coupling reaction.
RESULTS AND DISCUSSION
Key building blocks have been prepared according to the literature procedures to achieve the synthesis of desired azulene-thiophene derivatives. 3,4-Di-n-butyl-2,5-diiodothiophene (1) was prepared by Kumada–Tamao–Corriu coupling of 3,4-dibromothiophene in the presence of Ni(dppp)Cl2 and the treatment with I2 and HgO.9 3',4'-Di-n-butyl-2,2':5',2"-terthiophene10 and dithieno[3,2-b:2',3'-d]thiophene11 were prepared according to the literature procedures. The subsequent reactions with NIS afforded the corresponding diiodoterthiophene 2 and diiododithienothiophene 3, respectively. Sonogashira–Hagihara coupling of diethyl 6-bromoazulene-1,3-dicarboxylate12 and TMSA followed by removal of trimethylsilyl group with TBAF gave 6-ethynylazulene 4.13
Synthesis of di(6-azulenylethynyl)thiophenes 6–8 are summarized in Scheme 1 and Figure 1. Sonogashira–Hagihara coupling reactions of 1 and 2 with 4 afforded di(6-azulenylethynyl)thiophene 6 and terthiophene 7 in 71% and 84% yields, respectively. Although Pd(0)-catalyzed coupling of 3 with 4 afforded the desired product, the pure product could not be isolated due to the low solubility in common organic solvent. Thus, preparation of di(6-azulenylethynyl)dithienothiophene with hexyloxycarbonyl substituents at 1,3-positions in azulene was carried out. Dihexyl 6-ethynylazulene-1,3-dicarboxylate (5) was prepared by transesterification of diethyl 6-bromoazulene-1,3-dicarboxylate with 1-hexanol, Sonogashira–Hagihara coupling with TMSA, and detrimethylsilylation with KF.13 The cross-coupling of 3 with 5 in the presence of Pd(0) catalyst gave the bisadduct 8 in 80% yield.
To examine the electronic contribution of azulene as an electron donating group, thiophene, terthiophene, and dithienothiophene with 1-azulenylethynyl substituents were prepared. The Pd(0)-catalyzed coupling of 1,6-di-t-butyl-3-iodoazulene and methyl 3-iodoazulene-1-carboxylate with TMSA afforded the corresponding 1-ethynylazulenes 9 and 10, respectively.14,15 The cross-coupling reactions of 2 with 9 in the presence of Pd(PPh3)4 afforded bisadduct 11 in 93% yield together with butadiyne 15 (Scheme 2).14,16 Formation of 15 was attributable to the oxidative homo-coupling of an excess of 9. Bisadduct 12 was obtained by the similar cross-coupling under Sonogashira–Hagihara conditions as a sole product. On the other hand, bis(3-ethoxycarbonyl-1-azulenylethynyl)thiophene 13 and terthiophene 14 were obtained by the cross-coupling of 1 and 2 with 10 in the presence of PdCl2(PPh3)2 in 86% and 83% yields, respectively.
Iodoterthiophene 16 reacted with 4 under Sonogashira–Hagihara conditions to afford 17 in 85% yield. In order to prepare 2-(1-azulenylethynyl)-5"-(6-azulenylethynyl)terthiophene, iodination of 17 was carried out. When 17 was treated with NIS and AcOH in CH2Cl2, the desired product was not obtained. In 1H NMR of the product, two signals with the integral ratio of 1 : 0.8 were observed for H-2 at 8.83 and 8.86 ppm and for H-5,7 at 7.74 and 7.83 ppm of the azulene ring while a signal for α-H of the thiophene ring disappeared, which indicated that iodination occurred at α-position of thiophene and the product would be a mixture of two isomers with similar structure. New two singlet signals with the integral ratio of 1 : 0.8 were observed at 2.42 and 1.82 ppm corresponding to methyl group. Molecular ion peak appeared at m/z 966 together with fragment peaks at m/z 921 (M+−OEt) and 840 (M+−I) in the FAB MS, which indicated that molecular formula was C40H40I2O6S3. These results suggested that the product was a mixture of 2-iodoterthiophenes possessing 1-iodo-2-acetoxy or 2-iodo-1-acetoxy-2-azulen-6-ylvinyl groups at 5"-position shown in Scheme 3.
The absorption spectra of di(6-azulenylethynyl)thiophenes are shown in Figure 3(a). The intense absorptions of 6 and 7 were observed at 484 and 482 nm, respectively, in spite of the difference in the extension of the π-system. Dithienothiophene 8 showed a slight bathochromic shift by ca. 10 nm compared to 6 and 7. Their absorption edges reached around 600 nm. The absorption spectra of 11–14 are shown in Figure 3(b). They showed characteristic weak absorptions of the azulene system at 500–800 nm although the similar absorptions of 6–8 were not observed due to the intense absorptions at around 500 nm. The longest wavelength absorption maxima of 13 and 14 were observed at almost same wavelength. A slight bathochromic shift of terthiophene 11 compared to 14 was presumably attributable to the difference in electronic contribution between the electron-donating t-butyl groups of 11 and the electron-withdrawing methoxycarbonyl group of 14. The absorption edges of di(1-azuleneylethynyl)thiophenes 11–14 reached over 700 nm.
To estimate the electron distribution of di(azulenylethynyl)thiophenes, we have performed the B3LYP/6-31G(d) density functional theory (DFT) calculations of the molecular orbitals of 18, 19, and 20 as shown in Figure 4.17 The electron distribution of 18 was quite similar that of 20 for the four orbitals, LUMO+3, LUMO+2, LUMO+1, and LUMO. Their LUMO+3 and LUMO+2 were degenerated. While HOMO and HOMO−1 in 18 showed the similar distribution with degenerated states for HOMO−1 and HOMO−2 in 19, HOMO−2 and HOMO−3 in 18 showed the similar distribution for HOMO and HOMO−3 in 19, respectively. Their HOMO–LUMO energy gaps were estimated same values of 2.62 eV, which was larger than that of 19 (ΔE = 2.46 eV). On the other hand, the TD–DFT results of 18, 19, and 20 predicted the longest wavelength absorptions at 609 nm (f = 0.0098), 662 nm (f = 0.0045), and 550 nm (f = 0.0138), respectively, which were consistent with the absorption spectra. These bands mainly consist of the single-electron transition, HOMO to LUMO for 18 (75%) and 19 (85%), and HOMO−1 to LUMO for 20 (71%). This lowest energy transition in 19 showed the different distribution for those of 18 and 20.
In summary, we have demonstrated Pd-catalyzed ethynyl-liked oligomerization of azulene at the 1- and 6-positions with thiophene, terthiophene, and dithienothiophene at the α-positions. Bis(azulenylethynyl)thiophenes have been synthesized by Sonogashira–Hagihara coupling reaction of the corresponding diiodothiophenes with 1- and 6-ethynylazulenes. These compounds showed the small HOMO–LUMO energy gaps due to their effective π-conjugation. Their absorption edges reached in red to NIR region. These properties are expected to provide good performance organic photovolatic cell based on these bis(azulenylethynyl)thiophenes.
EXPERIMENTAL
General. Melting points were determined on a Yanaco micro melting point apparatus MP500D and are uncorrected. FAB mass spectra were measured on a JEOL JMS-700. IR spectra were measured on a Horiba FT-720 infrared spectrophotometer, and UV-vis spectra were measured on a JASCO V-570 spectrophotometer. 1H NMR spectra (13C NMR spectra) were recorded on a JEOL AL-400 at 400 MHz (100 MHz). Elemental analyses were performed at the Integrated Center for Sciences, Ehime University.
3,4-Di-n-butyl-2,5-bis[1,3-bis(ethoxycarbonyl)-6-azulenylethynyl]thiophene 6
To a degassed solution of 1 (88 mg, 0.20 mmol) and 4 (120 mg, 0.405 mmol) in Et3N (15 mL) were added CuI (7 mg, 0.04 mmol), PPh3 (8 mg, 0.03 mmol) and Pd(PPh3)4 (18 mg, 0.015 mmol) under an Ar atmosphere. The mixture was stirred at room temperature for 2 h. The precipitated crystals were collected by filtration and purified by column chromatography on silica gel with CHCl3 to give 8 (98 mg, 64%). The filtrate was diluted with CHCl3. The mixture was washed successively with water and brine, dried over Na2SO4, and concentrated under reduced pressure. The residue was purified by column chromatography on silica gel with CHCl3 to give 8 (11 mg, 7%).
Dark brown crystals; mp > 250 °C; MS (FAB) m/z 785 [M+H]+ and 740 [M+H−OEt]+; IR (KBr disk) νmax 2175 and 1689 cm−1; UV-vis (CH2Cl2) λmax, nm (log ε) 237 (4.78), 270 (4.51), 302 sh (4.52), 328 (4.65), 360 (4.53), 376 (4.53), 464 sh (4.85), and 484 (4.88); 1H NMR (400 MHz, CDCl3) δ 9.63 (d, 4H, J = 11.0 Hz, Az-4,8), 8.75 (s, 2H, Az-2), 7.81 (d, 4H, J = 11.0 Hz, Az-5,7), 4.43 (q, 8H, J = 7.1 Hz, CO2Et), 2.79 (t, 4H, J = 7.6 Hz, n-Bu), 1.67 (m, 4H, n-Bu), 1.50 (m, 4H, n-Bu), 1.46 (t, 12H, J = 7.1 Hz, CO2Et), and 1.03 (t, 6H, J = 7.3 Hz, n-Bu); 13C NMR (100 MHz, CDCl3) δ 164.69 (C=O), 149.27, 143.46 (Az-2), 143.05 (Az-3a,8a), 137.38(Az-4, 8), 135.80, 132.32 (Az-5,7), 120.45, 117.08 (Az-1,3), 100.78(-C≡C-), 88.90 (-C≡C-), 60.17 (CO2Et), 32.55 (n-Bu2), 28.62 (n-Bu1), 22.87 (n-Bu3), 14.62 (CO2Et), and 14.09 (n-Bu4); Anal. Calcd for C48H48O8S: C, 73.45; H, 6.16. Found: C, 73.16; H, 6.23.
3',4'-Di-n-butyl-5,5"-bis[1,3-bis(ethoxycarbonyl)-6-azulenylethynyl]-2,2':5',2"-terthiphene 7
To a degassed solution of 2 (89 mg, 0.15 mmol) and 4 (91 mg, 0.31 mmol) in Et3N (12 mL) were added CuI (5 mg, 0.03 mmol), PPh3 (9 mg, 0.03 mmol) and Pd(PPh3)4 (17 mg, 0.015 mmol) under an Ar atmosphere. The mixture was stirred at room temperature for 17 h. The precipitated crystals were collected by filtration and purified by column chromatography on silica gel with CHCl3 to give 9 (91 mg, 66%). The filtrate was diluted with CHCl3. The mixture was washed successively with water and brine, dried over Na2SO4, and concentrated under reduced pressure. The residue was purified by column chromatography on silica gel with CHCl3 to give 9 (26 mg, 18%).
dark brown powder; mp 277.6–278.0 °C; MS (MALDI-TOF) m/z 948 M+ and 740 [M−OEt]+; IR (KBr disk) νmax 2177 and 1689 cm−1; UV-vis (CH2Cl2) λmax, nm (log ε) 238 (4.85), 269 (4.56), 334 (4.77), 353 sh (4.73), 375 sh (4.68) and 482(4.83); 1H NMR (400 MHz, CDCl3) δ 9.65 (d, 4H, J = 11.0 Hz, Az-4,8), 8.77 (s, 2H, Az-2), 7.87 (d, 4H, J = 11.0 Hz, Az-5,7), 7.38 (d, 2H, J = 3.9 Hz, H-3,3"), 7.13 (d, 2H, J = 3.9 Hz, H-4,4"), 4.44 (q, 8H, J = 7.2 Hz, CO2Et), 2.77 (t, 4H, J = 7.9 Hz, n-Bu), 1.64–1.46 (m, 8H, n-Bu), 1.46 (t, 12H, J = 7.2 Hz, CO2Et), and 1.01 (t, 6H, n-Bu); Anal. Calcd for C44H34O8S: C, 70.86; H, 5.52. Found: C, 70.54; H, 5.43.
2,6-Bis[1,3-bis(hexyloxycarbonyl)-6-azulenylethynyl]dithieno[3,2-b:2',3'-d]thiophene 8
To a degassed solution of 3 (54 mg, 0.12 mmol) and 7 (128 mg, 0.313 mmol) in Et3N (10 mL) were added CuI (7 mg, 0.04 mmol), PPh3 (7 mg, 0.03 mmol) and Pd(PPh3)4 (17 mg, 0.015 mmol) under an Ar atmosphere. The mixture was stirred at room temperature for 16 h. The precipitated crystals were collected by filtration and purified by column chromatography on silica gel with 20% hexane/CHCl3 to give 10 (98 mg, 80%).
Dark brown crystals; mp 244.5–246.0 °C; MS (FAB) m/z 1009 [M+H]+; IR (KBr disk) νmax 2181 and 1685 cm−1; UV-vis (CH2Cl2) λmax, nm (log ε) 239 (4.83), 271 (4.60), 312 (4.77), 346 sh (4.66), 360 sh (4.66), 375 sh (4.61), and 493 (4.98); 1H NMR (400 MHz, CDCl3) δ 9.60 (d, 4H, J = 11.2 Hz, Az-4,8), 8.71 (s, 2H, Az-2), 7.80 (d, 4H, J = 11.2 Hz, Az-5,7), 7.53 (s, 2H, H-3,5), 4.36 (t, 8H, J = 6.8 Hz, CO2C6H13), 1.82 (tt, 8H, J = 6.8 and 7.4 Hz, CO2C6H13), 1.49 (m, 8H, CO2C6H13), 1.43–1.35 (m, 16H, CO2C6H13), and 0.93 (t, 12H, J = 7.1 Hz, CO2C6H13); 13C NMR (100 MHz, CDCl3) δ 164.72, 143.52, 143.03, 142.87, 137.28, 135.19, 132.68, 132.26, 126.95, 123.54, 117.15, 99.04, 88.95, 64.43, 31.60, 28.92, 25.89, 22.65, and 14.11; Anal. Calcd for C60H64O8S3: C, 71.40; H, 6.39. Found: C, 71.25; H, 6.38
3',4'-Di-n-butyl-5,5"-bis(3,6-di-t-butyl-1-azulenylethynyl)-2,2':5',2"-terthiphene 11
To a degassed solution of 2 (92 mg, 0.15 mmol) and 9 (118 mg, 0.446 mmol) in Et3N (10 mL) were added CuI (6 mg, 0.03 mmol), PPh3 (7 mg, 0.03 mmol) and Pd(PPh3)4 (18 mg, 0.015 mmol) under an Ar atmosphere. The mixture was stirred at room temperature for 17 h. After addition of aqueous NH4Cl, the organic layer was washed successively with water and brine, dried over Na2SO4, and concentrated under reduced pressure. The residue was purified by column chromatography on alumina with CHCl3 and hexane to CHCl3 to give 11 (123 mg, 93%) and 1,4-bis(3,6-di-t-butyl-azulenyl)buta-1,3-diyne (22 mg).
Dark brown solids; mp 140 °C (decomp); MS (FAB) m/z 885 [M+H]+; IR (KBr disk) νmax 2175 cm−1; UV-vis (CH2Cl2) λmax, nm (log ε) 240 (4.65), 272 sh (4.62), 302 (4.72), 314 (4.72), 441 (4.75), 614 (3.09), and 680 sh (2.84); 1H NMR (400 MHz, CDCl3) δ 8.57 (d, 2H, J = 10.7 Hz, Az-4), 8.49 (d, 2H, J = 10.5 Hz, Az-8), 7.85 (s, 2H, Az-2), 7.37 (dd, 2H, J = 10.5 and 1.7 Hz, Az-7), 7.33 (dd, 2H, J = 10.7 and 1.7 Hz, Az-5), 7.21 (d, 2H, J = 3.8 Hz, H-3,3" or H-4,4"), 7.06 (d, 2H, J = 3.8 Hz, H-3,3" or H-4,4"), 2.76 (t, 4H, J = 8.2 Hz, n-Bu), 1.65-1.40 (m, 8H, n-Bu), 1.58 (s, 18H, Az-3-t-Bu), 1.46 (s, 18H, Az-6-t-Bu), and 0.99 (t, 6H, J = 7.2 Hz, n-Bu); 13C NMR (100 MHz, CDCl3, 50 °C) δ 162.47, 141.44, 140.47, 139.22, 136.70 (Az-2), 135.70, 135.50 (Az-4), 135.22 (Az-8), 130.98 (C-3,3" or C-4,4"), 130.03, 125.70 (C-3,3" or C-4,4"), 124.60, 121.89 (Az-7), 121.04 (Az-5), 107.62, 91.73 (-C≡C-), 86.81 (-C≡C-), 38.58 (t-Bu), 33.39 (t-Bu), 32.93 (n-Bu2), 32.06 (Az-3-t-Bu), 31.88 (Az-6-t-Bu), 28.09 (n-Bu1), 23.11 (n-Bu3), and 13.93 (n-Bu4); Anal. Calcd for C60H68S3: C, 81.39; H,7.74. Found: C, 80.95; H, 7.84.
2,6-Bis(3,6-di-t-butyl-1-azulenylethynyl)dithieno[3,2-b:2',3'-d]thiophene 12
To a degassed solution of 3 (67 mg, 0.15 mmol) and 9 (110 mg, 0.417 mmol) in Et3N (11 mL) were added CuI (7 mg, 0.04 mmol), PPh3 (11 mg, 0.042 mmol) and Pd(PPh3)4 (27 mg, 0.038 mmol) under an Ar atmosphere. The mixture was stirred at room temperature for 17 h. After addition of aqueous NH4Cl, the organic layer was washed successively with water and brine, dried over Na2SO4, and concentrated under reduced pressure. The residue was purified by column chromatography on alumina with 10% CHCl3/hexane, 20% CHCl3/hexane and 30% CHCl3/hexane followed by recrystallization from CHCl3/hexane to give 12 (54 mg, 49%).
Dark brown crystals; mp >250 °C (decomp); MS (FAB) m/z 721 [M+H]+; IR (KBr disk) νmax 2177 cm−1; UV-vis (CH2Cl2) λmax, nm (log ε) 237 (4.63), 270 sh (4.55), 300 (4.77), 314 sh (4.69), 354 (4.44), 407 sh (4.57), 436 sh (4.76), 460 (4.84), 483 sh (4.71), 570 sh (3.19), 604 sh (3.19), 673 sh (2.96); 1H NMR (400 MHz, CDCl3) δ 8.58 (d, 2H, J = 10.7 Hz, Az-4), 8.49 (d, 2H, J = 10.5 Hz, Az-8), 7.86 (s, 2H, Az-2), 7.43 (s, 2H, H-4, 5), 7.40 (dd, 2H, J = 10.5 and 2.0 Hz, Az-7), 7.35 (dd, 2H, J = 10.7 and 2.0 Hz, Az-5), 1.58 (s, 18H, 3'-t-Bu) and 1.47 (s, 18H, 6'-t-Bu); 13C NMR (100 MHz, CDCl3) δ 162.60 (Az-6), 141.54 (Az-8a), 141.32 (dithienothiophene), 139.25 (Az-3), 136.59 (Az-2), 135.78 (Az-3a), 135.60 (Az-4), 135.22 (Az-8), 130.71 (dithienothiophene), 125.32 (dithienothiophene), 123.68 (C-3, 5), 122.12 (Az-7), 121.28 (Az-5), 106.99 (Az-1), 92.49 (-C≡C-), 87.45 (-C≡C-), 38.61 (6'-t-Bu), 33.35 (3'-t-Bu), 31.97 (3'-t-Bu) and 31.84 (6'-t-Bu); Anal. Calcd for C48H48S3·1/2H2O: C,78.96; H, 6.76. Found: C, 78.56; H, 6.91.
3,4-Di-n-butyl-2,5-bis(3-methoxycarbonyl-1-azulenylethynyl)thiophene 13
To a degassed solution of 1 (86 mg, 0.19 mmol) and 10 (110 mg, 0.525 mmol) in Et3N (10 mL) were added CuI (8 mg, 0.04 mmol) and PdCl2(PPh3)2 (13 mg, 0.019 mmol) under an Ar atmosphere. The mixture was stirred at room temperature for 19 h. The reaction mixture was filtered through an alumina pad and concentrated under reduced pressure. The residue was purified by column chromatography on silica gel with 25% CHCl3/hexane followed by recrystallization from CHCl3/hexane to give 13 (102 mg, 86%).
Dark green powder; mp 210.8 °C; MS (FAB) m/z 613 [M+H]+; IR (KBr disk) νmax 2183 and 1697 cm−1; UV-vis (CH2Cl2) λmax, nm (log ε) 241 (4.75), 292 (4.73), 312 sh (4.64), 367 (4.57), 378 sh (4.56), 418 (4.52), 458 sh (4.40) and 570 (3.14); 1H NMR (400 MHz, CDCl3) δ 9.60 (d, 2H, J = 9.8 Hz, Az-4), 8.71 (d, 2H, J = 9.8 Hz, Az-8), 8.47 (s, 2H, Az-2), 7.85 (dd, 2H, J = 9.8 and 9.8 Hz, Az-6), 7.58 (dd, 2H, J = 9.8 and 9.8 Hz, Az-5), 7.52 (dd, 2H, J = 9.8 and 9.8 Hz, Az-7), 3.97 (s, 6H, CO2Me), 2.81 (t, 4H, J = 7.8 Hz, n-Bu), 1.71 (m, 4H, n-Bu), 1.51 (tq, 4H, J = 7.3 and 7.3 Hz, n-Bu) and 1.01 (t, 3H, J = 7.3 Hz, n-Bu); 13C NMR (100 MHz, CDCl3) δ 165.09 (C=O), 145.97, 144.55 (Az-3a or 8a), 142.34 (Az-2), 141.14 (Az-3a or 8a), 140.31 (Az-6), 138.49 (Az-4), 137.79 (Az-8), 129.07 (Az-5), 127.55 (Az-7), 119.46, 116.45, 110.29, 91.12 (-C≡C-), 87.32 (-C≡C-), 51.34 (Me), 32.58 (n-Bu2), 28.66 (n-Bu1), 22.94 (n-Bu3) and 14.11 (n-Bu4); Anal. Calcd for C40H36O4S: C, 78.40; H, 5.92. Found: C, 78.20; H, 5.83.
3',4'-Di-n-butyl-5,5"-bis(3-methoxycarbonyl-1-azulenylethynyl)-2,2':5',2"-terthiophene 14
To a degassed solution of 2 (86 mg, 0.14 mmol), CuI (6 mg, 0.03 mmol) and PdCl2(PPh3)2 (12 mg, 0.017 mmol) in Et3N (5 mL) was added a solution of 10 (103 mg, 0.488 mmol) in Et3N (3 mL) under an Ar atmosphere. The mixture was stirred at room temperature overnight. The reaction mixture was filtered through an alumina pad and concentrated under reduced pressure. The residue was purified by column chromatography on silica gel with CHCl3 to give crude 14 and 1,4-bis(3-methoxycarbonyl-1- azulenyl)buta-1,3-diyne (33 mg). After recrystallization from CHCl3/hexane to give 14 (78 mg, 72%), the filtrate was purified by column chromatography on alumina with CHCl3 followed by recrystallization from CHCl3/hexane to give 14 (12 mg, 11%).
Dark brown powder; mp 140.3 °C (decomp); MS (FAB) m/z 777 [M+H]+; IR (KBr disk) νmax 2187 and 1699 cm−1; UV-vis (CH2Cl2) λmax, nm (log ε) 241 (4.81), 280 sh (4.75), 298 (4.80), 312 sh (4.75), 420 (4.72) and 563 (3.16); 1H NMR (400 MHz, CDCl3) δ 9.61 (d, 2H, J = 9.8 Hz, Az-4), 8.73 (d, 2H, J = 9.8 Hz, Az-8), 8.49 (s, 2H, Az-2), 7.86 (dd, 2H, J = 9.8 and 9.8 Hz, Az-6), 7.60 (dd, 2H, J = 9.8 and 9.8 Hz, Az-5), 7.55 (dd, 2H, J = 9.8 and 9.8 Hz, Az-7), 7.27 (d, 2H, J = 3.7 Hz, H-3 or 4), 7.08 (d, 2H, J = 3.7 Hz, H-3 or 4), 3.96 (s, 6H, CO2Me), 2.76 (t, 4H, J = 7.7 Hz, n-Bu1), 1.59 (m, 4H, n-Bu2), 1.49 (tq, 4H, J = 7.2 and 7.2 Hz, n-Bu3) and 1.00 (t, 6H, J = 7.2 Hz, n-Bu); 13C NMR (100 MHz, CDCl3) δ 165.09 (C=O), 144.68, 142.53 (Az-2), 141.20, 140.62, 140.35 (Az-6), 138.54 (Az-4), 137.94 (Az-8), 137.27 (C-2,2" or C-5,5"), 131.83 (C-3,3" or C-4,4"), 129.82 (C-3',4'), 129.15 (Az-5), 127.72 (Az-7), 125.64 (C-3,3" or C-4,4"), 123.39 (C-2,2" or C-5,5"), 116.46, 109.85 (Az-1), 89.65 (-C≡C-), 86.90 (-C≡C-), 51.34 (Me), 32.87 (n-Bu2), 28.04 (n-Bu1), 23.11 (n-Bu3) and 13.97 (n-Bu4); Anal. Calcd for C48H40O4S3·H2O: C, 72.51; H,5.32. Found: C, 72.75; H, 5.11.
3',4'-Di-n-butyl-3-(1,3-bis(ethoxycarbonyl)-6-azulenylethynyl)-2,2':5',2"-terthiophene 18
To a degassed solution of 17 (74 mg, 0.15 mmol) and 4 (52 mg, 0.18 mmol) in Et3N (10 mL) were added CuI (6 mg, 0.03 mmol), PPh3 (11 mg, 0.042 mmol) and Pd(PPh3)4 (18 mg, 0.015 mmol) under an Ar atmosphere. The mixture was stirred at room temperature overnight. After addition of aqueous NH4Cl, the organic layer was washed successively with water and brine, dried over Na2SO4, and concentrated under reduced pressure. The residue was purified by column chromatography on silica gel with CHCl3 to give 18 (136 mg, 85%).
Reddish brown crystals; mp 144.4–146.2 °C; MS (FAB) m/z 654 [M]+ and 609 [M−OEt]+; IR (KBr disk) νmax 2171 and 1684 cm−1; UV-vis (CH2Cl2) λmax, nm (log ε) 238 (4.59), 272 (4.35), 297 sh (4.29), 339 (4.53), 356 (4.52), 376 sh (4.48), and 467 (4.56); 1H NMR (400 MHz, CDCl3) δ 9.64 (d, 2H, J = 11.0 Hz, Az-4,8), 8.76 (s, 1H, Az-2), 7.86 (d, 2H, J = 11.0 Hz, Az-5,7), 7.37 (d, 1H, J = 3.8 Hz, H-3 or H-4), 7.33 (dd, 1H, J = 1.2 and 5.1 Hz, H-5"), 7.16 (dd, 1H, J = 1.2 and 3.7 Hz, H-3"), 7.10 (d, 1H, J = 3.8 Hz, H-3 or H-4), 7.07 (dd, 1H, J = 3.7 and 5.1 Hz, H-4"), 4.43 (q, 4H, J = 7.1 Hz, CO2Et), 2.76 (t, 2H, J = 8.2 Hz, n-Bu), 2.71 (t, 2H, J = 8.2 Hz, n-Bu), 1.63–1.39 (m, 8H, n-Bu), 1.46 (t, 6H, J = 7.1 Hz, CO2Et), 0.99 (t, 3H, J = 7.1 Hz, n-Bu) and 0.96 (t, 3H, J = 7.3 Hz, n-Bu); 13C NMR (100 MHz, CDCl3) δ 164.76 (C=O), 143.32 (Az-2), 143.05 (Az-6 or Az-3a,8a), 141.07, 140.44 (C-2 or C-5), 140.29 (C-3' or C-4'), 137.38 (Az-4,8), 136.08 (Az-6 or Az-3a,8a), 135.69, 134.38 (C-3 or C-4), 132.46 (Az-5,7), 130.98 (C-3' or C-4'), 128.91, 127.35 (C-4"), 126.08 (C-3"), 125.68 (C-3 or C-4), 125.60 (C-5"), 120.98 (C-2 or C-5), 116.94 (Az-1,3), 98.13 (-C≡C-), 89.41 (-C≡C-), 60.14 (Et), 32.91 (n-Bu2), 32.81 (n-Bu2), 28.12 (n-Bu1), 27.82 (n-Bu1), 23.11 (n-Bu3), 23.03 (n-Bu3), 14.63 (Et), 13.97 (n-Bu4) and 13.90 (n-Bu4); Anal. Calcd for C38H38O4S3: C, 69.69; H, 5.85. Found: C, 69.40; H, 5.79.
ACKNOWLEDGEMENTS
This work was partially supported by Grants-in-Aid for the Scientific Researches on Innovative Areas (Nos. 21108517, π−Space to HU) from MEXT, and B (No. 23350020 to HU), and Young Scientists B (No 24750041 to TO) from JSPS.
References
1. C. Reese, M. Roberts, M.-m. Ling, and Z. Bao, Mater. Today, 2004, 7, 20; CrossRef Y. Sun, Y. Liu, and D. Zhu, J. Mater. Chem., 2005, 15, 53; CrossRef A. R. Murphy and J. M. Fréchet, Chem. Rev., 2007, 107, 1066; CrossRef K. Takimiya, Y. Kunugi, and T. Otsubo, Chem. Lett., 2007, 36, 578; CrossRef A, Facchetti, Mater. Today, 2007, 10, 28; CrossRef M. T. Lloyd, J. E. Anthony, and G. G. Malliaras, Mater. Today, 2007, 10, 34. CrossRef
2. S. Shimamura, I. Osaka, E. Miyazaki, and K. Takimiya, Heterocycles, 2011, 83, 1187; CrossRef E. Miyazaki, T. Okanishi, Y. Suzuki, N. Ishine, H. Mori, K. Takimiya, and Y. Harima, Bull. Chem. Soc. Jpn., 2011, 84, 459; CrossRef T. Nishinaga, T. Miyata, M. Tateno, M. Koizumi, M. Takase, M. Iyoda, N. Kobayashi, and Y. Kunugi, J. Mater. Chem., 2011, 21, 14959; CrossRef M. Ashizawa, R. Kato, Y. Takanishi, and H. Takezoe, Chem. Lett., 2007, 36, 708; CrossRef Y. Sun, Y. Ma, Y. Liu, Y. Lin, Z. Wang, Y. Wang, C. Di, K. Xiao, X. Chen, W. Qiu, B. Zhang, G. Yu, W. Hu, and D. Zhu, Adv. Funct. Mater., 2006, 16, 426; CrossRef J. A. Merlo, C. R. Newman, C. P. Gerlach, T. W. Kelley, D. V. Muyres, S. E. Fritz, M. F. Toney, and C. D. Frisbie, J. Am. Chem. Soc., 2005, 127, 3997; CrossRef T. M. Pappenfus, M. W. Burand, D. E. Janzen, and K. R. Mann, Org. Lett., 2003, 5, 1535. CrossRef
3. K.-P. Zeller, 'Houben-Weyl: Methoden der Organischen Chemie' Vol. 5, ed. by H. Kropf, Georg Thieme, Stuttgart, 1985, pp. 127-418.
4. A. Julg, J. Chim. Phys., 1955, 52, 377; G. W. Wheland and D. E. Mann, J. Chem. Phys., 1949, 17, 264; CrossRef A. G. Anderson, Jr. and B. M. Steckler, J. Am. Chem. Soc., 1956, 81, 4941. CrossRef
5. J. O. Morley, J. Am. Chem. Soc., 1988, 110, 7660. CrossRef
6. S. Ito, N. Morita, and T. Asao, Bull. Chem. Soc. Jpn., 1995, 68, 1409; CrossRef S. Ito, S. Kikuchi, N. Morita, and T. Asao, J. Org. Chem., 1999, 64, 5815. CrossRef
7. E. Amir, R. J. Amir, L. M. Campos, and C. J. Hawker, J. Am. Chem. Soc., 2011, 133, 10046. CrossRef
8. Y. Yamaguchi, Y. Maruya, H. Katagiri, K. Nakayama, and Y. Ohba, Org. Lett., 2012, 14, 2316. CrossRef
9. C. Ringenbach, A. De Nicola, and R. Ziessel, J. Org. Chem., 2003, 68, 4708. CrossRef
10. C. H. Araki, H. Endo, G. Masuda, and T. Ogawa, Chem. Eur. J., 2004, 10, 3331; CrossRef J. Krömer and P. Bäuerle, Tetrahedron, 2001, 57, 3785; CrossRef K. Tamao, S. Kodama, I. Nakajima, and M. Kumada, Tetrahedron, 1982, 38, 3347; CrossRef C. Wang, M. E. Benz, E. LeGoff, J. L. Schindler, J. Allbritton-Thomas, C. R. Kannewurf, and M. G. Kanatzidis, Chem. Mater., 1994, 6, 401. CrossRef
11. J. Frey, S. Proemmel, M. A. Armitage, and A. B. Holmes, Org. Synth., 2006, 83, 209; F. Allared, J. Hellberg, and T. Remonen, Tetrahedron Lett., 2002, 43, 4553; CrossRef F. De Jong and M. J. Janssen, J. Org. Chem., 1971, 36, 1645. CrossRef
12. R. N. McDonald, J. M. Richmond, J. R. Curtis, H. E. Petty, and T. L. Hoskins, J. Org. Chem., 1976, 41, 1811. CrossRef
13. S. Ito, H. Inabe, N. Morita, K. Ohta, T. Kitamura, and K. Imafuku, J. Am. Chem. Soc., 2003, 125, 1669; CrossRef S. Ito, H. Inabe, T. Okujima, N. Morita, M. Watanabe, N. Harada, and K. Imafuku, J. Org. Chem., 2001, 66, 7090; CrossRef S. Ito, H. Inabe, T. Okujima, N. Morita, M. Watanabe, N. Harada, and K. Imafuku, Tetrahedron Lett., 2001, 42, 1085; CrossRef S. Ito, H. Inabe, T. Okujima, N. Morita, M. Watanabe, and K. Imafuku, Tetrahedron Lett., 2000, 41, 8343. CrossRef
14. K. H. H. Fabian, A. H. M. Elwahy, and K. Hafner, Eur. J. Org. Chem., 2006, 791; CrossRef K. H. H. Fabian, A. H. M. Elwahy, and K. Hafner, Tetrahedron Lett., 2000, 41, 2855. CrossRef
15. T. Shoji, S. Ito, K. Toyota, M. Yasunami, and N. Morita, Chem. Eur. J., 2008, 14, 8398; CrossRef N. Morita, S. Moriyama, T. Shoji, M. Nakashima, M. Watanabe, S. Kikuchi, S. Ito, and K. Fujimori, Heterocycles, 2004, 64, 305. CrossRef
16. M. B. Nielsen, S. L. Broman, M. Å. Petersen, A. S. Andersson, T. S. Jensen, K. Kilså, and A. Kadziola, Pure. Appl. Chem., 2010, 82, 843; CrossRef M. A. Petersen, K. Kilså, A. Kadziola, and M. B. Nielsen, Eur. J. Org. Chem., 2007, 1415; CrossRef A. H. M. Elwahy, Tetrahedron Lett., 2002, 43, 711. CrossRef
17. The B3LYP/6-31G(d) density functional theory calculations were performed by Gaussian R 03W.