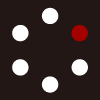
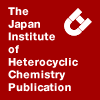
HETEROCYCLES
An International Journal for Reviews and Communications in Heterocyclic ChemistryWeb Edition ISSN: 1881-0942
Published online by The Japan Institute of Heterocyclic Chemistry
e-Journal
Full Text HTML
Received, 29th May, 2012, Accepted, 2nd August, 2012, Published online, 13th August, 2012.
DOI: 10.3987/COM-12-S(N)22
■ COMPUTATIONAL STUDY ON ACID CATALYZED RING-OPENING REACTION OF 2,4-DIETHOXY-6-TRIFLUOROMETHYL-3,4-DIHYDRO-2H-PYRAN WITH THIOLS
Norio Ota, Yasuhiro Kamitori,* Mizuki Hatakenaka, and Etsuji Okada*
Department of Chemical Science and Engineering, Graduate School of Engineering, Kobe University, 1-1 Rokkodai-cho, Nada-ku, Kobe 657-8501, Japan
Abstract
2,4-Diethoxy-6-trifluoromethyl-3,4-dihydro-2H-pyran was reacted with thiols in the presence of p-toluenesulfonic acid by heating followed by treatment with trifluoroacetic acid to afford the corresponding 4-trifluoroacetyl-1,3-butadienyl sulfides selectively. Our DFT calculation results suggest thermodynamically controlled attack of thiols at 6-position of pyrylium intermediate followed by electrocyclic ring-opening as a reasonable mechanism for this reaction.In recent years, a number of researches have been reported about the development of new synthetic methodologies for various kinds of fluorine-containing heterocycles. In addition, these researches have encouraged explorations of new bioactive skeletons which show noteworthy performance in life science fields such as medicinal and agrochemical discoveries.1-4 We have focused on such scope to develop convenient synthetic methods for two types of fluorinated 1,2-butadienyl sulfides, 4-trifluoroacetyl-1,3- butadienyl sulfides (1) and 4,4’-bis(trifluoroacetyl)-1,3-butadienyl sulfides (2),5,6 which work as versatile building blocks for novel fluorine-containing heterocycles because of its functionalized hexa-3,5- dien-2-one systems.7-9 The former dienes (1) were synthesized by the reaction of 2,4-diethoxy-6- trifluoromethyl-3,4-dihydro-2H-pyran (3), which could be prepared by hetero Diels-Alder reaction of 4-ethoxy-1,1,1-trifluorobut-3-en-2-one with ethyl vinyl ether,10 with thiols in the presence of p-toluenesulfonic acid on heating followed by treatment with trifluoroacetic acid (Scheme 1).5 In contrast to this acidic condition, the latter dienes (2) could be obtained by the reaction of 2-ethoxy-4-isobutoxy-5-trifluoroacetyl-6-trifluoromethyl-3,4-dihydro-2H-pyran (4) prepared from 1,1,1,5,5,5-hexafluoro-3-(isobutoxymethylidene)pentane-2,4-dione and ethyl vinyl ether11 with sodium thiolates.6
The mechanistic studies about these ring-opening reactions giving 1 and 2 especially give us important knowledges to develop efficient methods accessing similar type of fluorine-containing dienes as novel building blocks. We previously proposed a reasonable mechanism for the reaction of 4 with thiolates affording 2.12 However, a profile of mechanism from 3 to 1 has not been demonstrated yet, which undergoes the reaction under the different conditions.
Here we wish to report our computational study for the acid catalyzed reaction of dihydropyran (3) with thiols giving dienes (1) selectively. Probable pathways from 3 to 1 were examined on the basis of DFT calculations (RB3LYP/6-31G*) using methanethiol as a model of thiols to elucidate the mechanism of this reaction.
At the first step of the reaction of dihydropyran (3), 6-cation (5a) and 4-cation (6a) are assumed to be formed in the presence of p-toluenesulfonic acid, and so we can propose the two pathways, Path A and Path B, accessing dienes (1) from 3 as illustrated in Scheme 2. On Path A, a nucleophilic attack of thiols to 5a,b gives adducts 7 followed by de-ethanolization on 7 giving 2H-pyrans (8).13 Finally, 8 undergoes an electrocyclic ring-opening to afford 1. Path B, on the other hand, is initiated with the de-ethanolization on 4-cation (6a,b) giving pyrylium (9) followed by the regioselective attack of thiols at 6-position of 9 giving 8.
Based on our previous work as to the analogous reaction course, Path A has to be denied since 6-cation (5a,b) which should be the key intermediate of this path seems to be hard to form. Our knowledge has suggested about 21 kcal/mol of instability in 6-cation (5a,b) compared to 4-cation (6a,b).14 It means that this value accounts for the exclusive formation of 6a,b from 3 in the presence of acid catalyst.15 Accordingly, Path A in this reaction was excluded since it was suggested that 5a,b was absent.
In Path B, the selective formation of pyrylium (9) from 4-cation (6a,b) has to proceed. Since this de-ethanolization is an endothermic process requiring the external energy no more than 11.3 kcal/mol,14 this step is assumed to proceed smoothly under the experimental conditions in Scheme 1. However, the direct attack of thiols to 6a giving sulfides (10) is also possible as depicted in Scheme 3.
This exothermic process giving 10 would significantly interfere with the formation of 9. The de-ethanolization on 10 affords 6-cation (11), while the inverse reaction from 10 reproduces 4-cation (6a). We computed optimized structures and their energies for 10 (R= Me) and 11 (R= Me) as shown in Figure 1.
Although the both elimination processes are endothermic, our calculations predicted that the de-ethanolization from 10 (R= Me) to 11 (R= Me) requires over 22 kcal/mol more energy than the process reproducing 6a from 10 (R= Me).16 Moreover, the necessary energy for the ionization of dihydropyran (3) to form 4-cation (6a) is slightly larger (ΔΔE= 0.1 kcal/mol) than the corresponding energy for the process from 10 to 6a. These results suggest the presence of equilibrium between 6a and 10 under the reaction conditions. Consequently, 6a would be completely converted to pyryliums (9). It also would be supported by the fact that dihydropyrans (10) was not detected at any stage of the reaction from 3 to dienes (1).5
As we reported previously,17 the frontier electron density (LUMO of 9) at C4 is considerably huge compared to C6 (Figure 2). These results predict that the kinetically controlled reaction of pyrylium (9) with thiols occurs selectively at C4 to give 4H-pyrans (12) but not 2H-pyrans (8). On the other hand, 2H-pyran (8, R= Me) is 2.2 kcal/mol more stable than 12 (R= Me). The Boltzmann equation gives an estimate of 42/1 for the ratio of the products (8/12). Therefore, the selective formation of 8 can be interpreted as the result of thermodynamically controlled attack of thiols at C6 of pyrylium (9).
In contrast to the reaction of 3 with thiols, it has been known that acid catalyzed reaction of 3 with aromatic compounds instead of thiols under similar conditions gave ca. 1 : 1 mixture of 4H-pyrans (14) and dienes (15) as depicted in Scheme 4.18
In this case, the energy value of 2H-pyran (13, Ar= Ph) which is the precursor of 15 (Ar= Ph) is very close to 14 (Ar= Ph) (Figure 2). The above energy differences between the corresponding 2H-pyrans and 4H-pyrans would control which course the reactions of 3 with thiols or aromatic compounds would adopt, respectively.
Finally, we estimated the activation energy for the ring-opening process from 2H-pyrans (8) resulted by the reaction of pyrylium (9) with methanethiol as a model of thiols. The optimized transition state structure (TS8)19 is illustrated in Figure 3. The energy difference between 8 and TS8 is estimated to be ca. 19 kcal/mol which is corresponding to the activation energy of this process. The ring-opening of 8 giving dienes (15) is exothermic process with release of 1.7 kcal/mol. In the presence of acid catalyst, these (Z,E)-dienes (15) readily isomerize to (E,E)-dienes (1) to contribute to the thermodynamical stabilization. The above results suggest that the irreversible ring-opening of the intermediates (8) will easily occur under the experimental conditions to afford 1 (Scheme 1).
In conclusion, the acid catalyzed ring-opening reaction of dihydro-2H-pyran (3) with thiols giving 4-trifluoroacetyl-1,3-butadienyl sulfides (1) has been reasonably explained by the pathway (Path B) which is comprised of initial formation of pyrylium (9) via 4-cation (6a,b), subsequent thermodynamically controlled attack of thiols at C6 of 9, and final electrocyclic ring-opening of 8.
However, the following experimental results could not be always explained by this mechanism. It was found that O-S exchanged dihydropyrans (7) were obtained mainly in some cases together with 1 when the reaction was quenched before the treatment with trifluoroacetic acid (Scheme 5).5
Additionally, upon treatment with trifluoroacetic acid, the mixture was completely converted into 1.5 Even if these results seem to indicate the presence of additional reaction paths, none of our DFT calculations suggested and rationalized the formation of 7 from 3. It means that the acid catalyzed reaction of 3 with thiols is very interesting reaction which can not be figured out completely by the present computational analysis. Even though we are continuing to make an effort to bring up the other hypothetical reaction course for 7 based on further accumulated experimental data, we unfortunately could not achieve it as of now. Further work is under progress on synthetic application of 1 to various fluorine-containing heterocycles having potential biological importance.
COMPUTATIONAL METHODS
All calculations employed in this paper were accomplished using the computer programs packages PC SPARTAN 02 and PC SPARTAN 04.20 All calculations for geometrical optimizations were performed with the 6-31G* basis set at B3LYP21 level. The starting geometries employed for all optimizations were resulted from molecular mechanics using SYBYL22 force field and subsequent semi-empirical PM323 optimizations. The calculations for transition state geometries and their energies were also taken with the 6-31G* basis set at B3LYP level.
References
1. R. Filler and Y. Kobayashi, ‘Biomedicinal Aspects of Fluorine Chemistry,’ Kodansha & Elsevier Biomedical, Tokyo, 1982.
2. R. Filler, ‘Organofluorine Chemicals and Their Industrial Applications,’ Ellis Horwood, London, 1979.
3. J. T. Welch, Tetrahedron, 1987, 43, 3123. CrossRef
4. R. Filler, Y. Kobayashi, and L. M. Yagupolskii, ‘Organofluorine Compounds in Medicinal Chemistry and Biomedical Applications,’ Elsevier, Amsterdam, 1993.
5. M. Hojo, R. Masuda, and E. Okada, Chem. Lett., 1990, 113. CrossRef
6. M. Hojo, R. Masuda, and E. Okada, Synthesis, 1991, 46. CrossRef
7. (a) T. Kurihara, K. Nasu, Y. Mizuhara, and K. Hayashi, Chem. Pharm. Bull., 1982, 30, 2742; CrossRef (b) F. Palacios, J. Vicario, and D. Aparicio, Eur. J. Org. Chem., 2006, 2843; CrossRef (c) W. Wendelin, H. W. Schramm, and A. Blasi-Rabassa, Monatsh. Chem., 1985, 116, 385; Chem. Abstr., 1985, 103, 178216; CrossRef (d) W. Bethaeuser, M. Regitz, and W. Theis, Tetrahedron Lett., 1981, 22, 2535. CrossRef
8. (a) L. E. Kiss, H. S. Ferreira, and D. A. Learmonth, Org. Lett., 2008, 10, 1835; CrossRef (b) J.-C. Wypych, T. M. Nguyen, M. Benechie, and C. Marazano, J. Org. Chem., 2008, 73, 1169; CrossRef (c) L. E. Kiss and D. A. Learmonth, Patent, 2009, WO 2009054742, A2; Chem. Abstr., 2009, 150, 472566; (d) C. Jutz, R. M. Wagner, A. Kraatz, and H. G. Loebering, Liebigs Ann., 1975, 874; Chem. Abstr., 1975, 83, 114166.
9. (a) E. Okada, R. Masuda, and M. Hojo, Heterocycles, 1992, 34, 1927; CrossRef (b) A. K. Shanazarov, V. A. Kuzovkin, V. V. Chistyakov, and V. G. Granik, Khimiya Geterotsiklicheskikh Soedinenii, 1991, 86; Chem. Abstr., 1991, 115, 114416.
10. M. Hojo, R. Masuda, and E. Okada, Synthesis, 1989, 215. CrossRef
11. M. Hojo, R. Masuda, and E. Okada, Synthesis, 1990, 347. CrossRef
12. N. Ota, Y. Kamitori, Y. Tamai, G. Feng, M. Hatakenaka, and E. Okada, Heterocycles, 2011, 83, 2509. CrossRef
13. The additional pathway giving 2H-pyrans (8) from 6-cation (5a,b) via pyrylium (9) may be also possible (Scheme 2).
14. N. Ota, Y. Kamitori, R. Shirai, M. Hatakenaka, and E. Okada, Appl. Sci., 2012, 2, 129. CrossRef
15. On the basis of Boltzmann equation, the energy deference, ca. 21 kcal/mol is corresponding to the products ratio of 6a,b/5a,b over 1 x 1015.
16. Previously calculated energy values were used for 3, 6a, 6b, and 9 (see ref. 14 and 17). The energies of ethanol and methanethiol were calculated as -155.03430 au and -438.69835 au, respectively.
17. N. Ota, Y. Kamitori, E. Nishiguchi, M. Ishii, and E. Okada, Heterocycles, 2011, 82, 1337. CrossRef
18. N. Ota, E. Okada, D. Shibata, S. Adachi, and S. Saikawa, Heterocycles, 2010, 80, 515. CrossRef
19. Our calculations for vibrational frequencies of TS8 showed only one imaginary frequency at -417.6 cm-1 having the vibrational mode corresponding to the bond formation and cleavage between C2 and O1.
20. Wavefunction, Inc. Irvine, CA, USA.
21. A. D. Becke, J. Chem. Phys., 1993, 98, 5648. CrossRef
22. M. Clark, R. D. Cramer, III, and N. Van Opdenbosch, J. Computational Chem., 1989, 10, 982. CrossRef
23. J. J. P. Stewart, J. Computational Chem., 1989, 10, 209. CrossRef