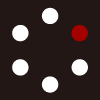
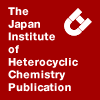
HETEROCYCLES
An International Journal for Reviews and Communications in Heterocyclic ChemistryWeb Edition ISSN: 1881-0942
Published online by The Japan Institute of Heterocyclic Chemistry
e-Journal
Full Text HTML
Received, 18th June, 2012, Accepted, 7th August, 2012, Published online, 28th August, 2012.
DOI: 10.3987/COM-12-S(N)38
■ STEREOCONTROLLED TOTAL SYNTHESIS OF (−)-FR901483
Shigeru Ieda, Akitaka Masuda, Mami Kariyama, Toshiyuki Wakimoto, Tomohiro Asakawa, Tohru Fukuyama,* and Toshiyuki Kan*
Synthetic Organic & Medicinal Chemistry, School of Pharmaceutical Science, University of Shizuoka, 52-1 Yada, Suruga, Shizuoka 422-8526, Japan
Abstract
The total synthesis of a potent immunosuppressant (–)-FR901483 is accomplished. The skeleton itself is constructed by the Ugi 4CC reaction, subsequent intramolecular Dieckmann condensation, and a diastereoselective intramolecular aldol reaction. However, the remarkable feature is the stereoselective incorporation of the p-methoxybenzyl and methylamino groups within the tricyclic core skeleton.INTRODUCTION
(–)-FR901483 (1) is a novel immunosuppressant isolated from the fermentation broth of Cladobotryum sp. No. 11231.1 Unlike Cyclosporin A and tacrolimus,1 which are powerful, but have strong side effects, 1 has a different mode of action and is expected to replace or reduce their dosages. Moreover, 1 is an attractive total synthesis target due to its promising biological activity and unique tricyclic structure. Although several total syntheses and synthetic studies of 1 have been reported to date, few are stereoselective.2,3,4 Previously, we reported the stereocontrolled synthesis of optically active key intermediate 3.3l Herein we describe a detailed stereocontrolled total synthesis of optically active 1. This synthesis may realize diverse analogues.
RESULTS AND DISCUSSION
Scheme 1 illustrates our retrosynthetic analysis of 1. Because the crucial step in our racemic synthesis is an intramolecular aldol reaction of keto-aldehyde 5a to provide tricycle intermediate 4a, we initially attempted an enantioselective intramolecular aldol reaction of 5a by means of a chiral organocatalyst.
Organocatalytic mediated aldol reactions have received much attention.5 Thus, we anticipated that 5a would provide optically active 4a and incorporate three stereocenters. Although we tested several catalysts for the enantioselective desymmetrization6 of 5a, the results were unsatisfactory. Table 1 shows typical reaction conditions.
Next we examined a diastereoselective aldol reaction of 5b to construct tricycle intermediate 4b, which could easily be converted into intermediate 2 relying on our racemic synthesis. We envisioned the facile construction of optically pure azaspiro intermediate 5b to be the crucial step. Although α-trisubstituted amines have been synthesized via numerous procedures, we opted to construct 7 using the Ugi 4CC reaction7 because the Ugi reaction can assemble complex molecules from simple components (Scheme 2).
Due to the limited examples of the Ugi 4CC reaction with a ketone system, we initially explored suitable reaction conditions using commercially available cyclohexanedione monoethylene acetal 9, acetic acid 10, p-methoxyphenyl isocyanide 12, and amine moieties 138 in MeOH. As indicated in Table 2, the R3 substituent in fragment 13 was essential, and the ethereal substituents in 12 were unsatisfactory, but an sp2 carbon in R3 accelerated the reaction rate. Consequently, we selected the vinyl group as an aldehyde equivalent.
Optically active amine 11 was prepared in seven steps from commercially available N-Boc-tyrosine methyl ester 15,9 while oxirane 19 was prepared in accordance with Kaneka’s patent.10 A classical reduction of oxirane via thiirane afforded desired compound 20, and recrystallization improved its enantiomeric excess up to 100% ee.
A mixture of cyclohexanedione monoethlyene acetal 9, acetic acid 10, optically active amine 11, p-methoxyphenyl isocyanide 12, in MeOH was allowed to stand at room temperature to afford 21. This reaction efficiently assembled all the carbon atoms necessary to construct tricyclic key intermediate 3 in a single step. Although hydrolysis of the C-terminal amide bond in the Ugi adduct can be troublesome,11 methanolysis of the p-methoxyphenyl amide in 21 proceeded smoothly with a concomitant acetal exchange to provide 22.
Presumably, this reaction proceeded via oxazolonium intermediate 24 through neighboring group participation. As shown in Scheme 5, the acetamide moiety should play a key role in this transformation; this speculation is supported by the fact that the amide of Passerini compound 25, which has an acetate instead of an acetamide moiety, is unaffected under the same methanolysis conditions. Additionally, trimethyl orthoformate should play a key role in dehydration and trapping of p-anisidine.
Recently, we developed a novel, odorless isonitrile, which is advantageous for cleavage of the amide bond in the Ugi adduct (Scheme 6). Additionally, the Ugi 4CC reaction with isonitrile 26 yielded 22 after treatment under acidic conditions. These results are reasonable because the mechanism appears to be similar to the methanolysis of 21.
The subsequent intramolecular Dieckmann condensation effectively constructed the spiro-lactam ring. Thus, treatment with LHMDS caused 22 to cyclize, yielding 28. The ensuing deoxygenation of the carbonyl group in 28 was performed via a three-step sequence to give desired lactam 31: the reduction of the ketone with NaBH4, dehydration with phosphoryl chloride, and a chemoselective one-electron reduction of the resultant unsaturated lactam. In addition, simultaneous hydrolysis of the dimethyl acetal occurred in the last step of the acidic work-up.
During the dehydration reaction with phosphoryl chloride, concomitant formation of methyl enol ethers occurred. After the mixture was reduced with magnesium metal in methanol without purification, hydrolysis of both the enol ether and dimethyl ketal in an acidic work up gave ketone 31. At this stage, the oxidative cleavage of the terminal olefin in 31 was performed by ozonolysis to afford 5b.
With desired optically active keto-aldehyde 5b in hand, we then focused on the diasteroselective intramolecular aldol reaction. Although Snider has reported a similar intramolecular cyclization of 5b, their reaction conditions could not be applied to our study because the cyclization reaction proceeded with a significant amount of racemization under basic conditions and the reproducibility was insufficient. Therefore, we selected acidic conditions at room temperature. Treatment of keto-aldehyde 5b with acetic acid and a catalytic amont of pyrrolidine12 afforded 4b via the desired cyclization where the two chiral centers were generated in one step. Although the stereochemistry of the hydroxy group in 4b was the opposite of the natural product,13 it was necessary to control the endo-reduction of the ketone in 4b.
It is plausible that thermodynamic control is responsible for the stereochemistry of diequatorial aldol product 4b. The ratio of the byproducts, which can analyze the selectivity of the intramolecular aldol reaction (Scheme 9), indicates that chelation with hydrogen bonding via a proton and steric hinderance of p-methoxybenzyl group contributed to the selectivity.
Similar to the route established for 4b, stereoselective reduction of 4b was carried out by NaBH(OAc)3,14,15 through coordination with the hydroxyl group to give 36. Selective protection of the exo-oriented hydroxy group of 36 as a TBS ether and a subsequent Swern oxidation of the remaining alcohol provided key intermediate 3, whose enantiomeric excess (96% ee) was determined by chiral HPLC.
A one-electron reduction converted ketone 3 into the exo-oriented alcohol because the reduction with lithium aluminum hydride exclusively gave the undesired endo-alcohol. Because the hydride should attack from the convex face of 3, a one-electron reduction with samarium (II) was investigated. Thus, treatment with samarium (II) diiodide (SmI2)16 in the presence of HMPA at –78 °C smoothly reduced ketone 3 to provide desired 38. In this SmI2 mediated reduction of 3, the addition of HMPA was essential for high selectivity17. Subsequent protection of 38 with the TBS ether gave 39. As shown in Scheme 11, an amine group was stereoselectively incorporated using a one-electron reduction as a key step. Upon treatment of 40 with sodium nitrite under acidic conditions in a biphasic medium, sequential nitrosylation and decarboxylation proceeded smoothly to provide oxime 41. The addition of a phase transfer catalyst improved the reproducibility in the reaction. The crucial reduction of 41 was accomplished by treatment with zinc in acetic acid to provide desired amine 42 as a single isomer. As shown in Figure 1, the approach of the proton from the convex face of more stable intermediate 43 could explain the selectivity.
Mono-N-methylation of primary amine 42 was achieved in a stepwise manner. After the conversion of 42 into formamide 45, treatment with lithium aluminum hydride allowed the simultaneous reduction of both the lactam and formamide, while concomitant deprotection of one of the TBS groups provided corresponding methylamine derivative 46, which was subsequently protected with a Cbz group to afford 47.
After the deprotection of the TBS ether, the phosphate ester was selectively incorporated via the phosphoramidite method18 to give 49.19 Finally, simultaneous cleavage of Cbz and the benzyl ester groups under hydrogenolysis conditions yielded (–)-FR901483 (1). The spectral data (relative optical rotation, 1H NMR, 13C NMR, IR, and HRMS) of 1 fully agreed with those of the natural product (Scheme 12).
In conclusion, a highly stereoselective total synthesis of (–)-FR901483 (1) was achieved via the Ugi 4CC reaction, subsequent intramolecular Dieckmann condensation, and a diastereoselective intramolecular aldol reaction. Our synthesis stereoselectively constructed the exo-oriented alcohol by a SmI2 mediated reduction. Finally, a one-electron reduction determined the amine stereochemistry at C(10). The versatility and flexibility of this total synthesis should contribute to future syntheses of FR901483 analogues.
EXPERIMENTAL
General. Nuclear magnetic resonance [13C NMR (68 MHz)] spectra were determined on a JEOL EX-270 instrument, [1H NMR (400 MHz) and 13C NMR (100 MHz)] spectra were determined on a JEOL-LA400 instrument, and [1H NMR (500 MHz) and 13C NMR (125 MHz)] spectra were determined on a JEOL ECA 500 instrument and JEOL α−500 instrument. Chemical shifts for 1H NMR are reported in parts per million (ppm) downfield from tetramethylsilane (δ) in deuterochloroform (CDCl3) or deuteromethanol (CD3OD) as an internal standard or relative to the signal at 7.26 (3.31) ppm for deuterochloroform (deuteromethanol), while coupling constants are in hertz (Hz). The following abbreviations are used for spin multiplicity: s = singlet, d = doublet, t = triplet, q = quartet, m = multiplet, and br = broad. Chemical shifts for 13C NMR are reported in ppm relative to the centerline of the triplet at 77.0 ppm for deuterochloroform or the centerline of a septet at 118.2 (49.0) ppm for deuteroacetonitrile (CD3CN) [deuteromethanol (CD3OD)]. Melting points (mp) were determined on a Yanaco Micro Melting Point Apparatus. Infrared spectra (IR), which are reported in wavenumbers (cm–1), were recorded on a JASCO FT/IR-410 Fourier Transform Infrared Spectrometer. Mass spectra (MS) were obtained on a JEOL JMS-GCmate MS-DIP20 with polyethylene glycol as the matrix or a JEOL MStation 700 using the Fast Atom Bombardment (FAB) method and 3-nitrobenzylalcohol as the matrix. Analytical thin layer chromatography (TLC) was performed on 0.25-mm thick Merck precoated analytical plates of silica gel 60 F254. Preparative TLC separations were conducted on 0.50-mm thick Merck precoated of silica gel 60 F254. Compounds were eluted from the adsorbent with 10% methanol (MeOH) in chloroform (CHCl3). Flash column chromatography separations were performed on KANTO CHEMICAL Silica Gel 60 (40-100 mesh). All non-aqueous reactions were carried out in oven-dried glass apparatuses under a slight positive pressure of argon. Prior to use, all solvents were dried over molecular sieves 3A or 4A. All other reagents were commercially available, and unless otherwise specified, were used without further purification.
tert-Butyl [(2S)-1-(4-methoxyphenyl)but-3-en-2-yl]carbamate (20).
Potassium thiocyanate (22.0 g, 226 mmol) was added to a stirred suspension of 19 (22.1 g, 75.3 mmol) in EtOH (400 mL) and H2O (300 mL) at room temperature. The mixture was heated to 60 °C and stirred for 24 h before cooling to room temperature. Then H2O (400 mL) was added. The mixture was extracted with AcOEt twice. The combined organic layer was washed with brine, dried over anhydrous MgSO4, and then passed through a pad of silica gel. The solvent was removed under reduced pressure. The residual mixture was used in the next step without further purification.
To a stirred solution of the crude product in toluene (50 mL), PPh3 (7.29 g, 27.8 mmol) was added at room temperature. The mixture was heated to 80 °C and stirred for 12 h before cooling to room temperature. The mixture was diluted with toluene (50 mL), and then H2O (50 mL) was added. The resulting precipitate was filtered with a sintered glass filter. After the organic layer was separated, the aqueous layer was extracted with AcOEt twice. The combined organic layer was washed with brine and dried over anhydrous MgSO4. The solvent was removed under reduced pressure. The residual mixture was purified by silica gel chromatography (toluene-AcOEt:n-hexane=1:20) to give 20 (10.9 g, 39.2 mmol) as a colorless crystal. The resulting crystal was recrystallized from toluene–n-heptane to afford purified 20 (9.81 g, 35.2 mmol, 47%, 100% ee20) as a colorless crystal. [α]D26.4 34.5 (c 1.01, CHCl3); IR (firm) 3363, 2981, 1685, 1612, 1511, 1441, 1244, 1167 cm-1; 1H-NMR (CDCl3, 400MHz) δ 1.41 (9H, s), 2.77 (2H, br. d, J = 6.4 Hz), 3.78 (3H, s), 4.37 (1H, br. s), 4.52 (1H, br. s), 5.06-5.11 (2H, m), 5.78 (1H, ddd, J = 5.3, 10.3, 15.9 Hz), 6.83 (2H, d, J = 8.5 Hz), 7.09 (2H, d, J = 8.5 Hz); 13C-NMR (CDCl3, 100MHz) δ 28.3, 40.4, 53.5, 55.1, 79.2, 113.6, 129.3, 130.4, 138.1, 144.6, 155.2, 158.1; HRMS (ESI) calculated for C16H23NNaO3 300.1576 [(M+Na)+], found 300.1563.
(2S)-1-(4-Methoxyphenyl)but-3-en-2-amine (11).
6N-HCl (13 mL) was added to a solution of 20 (1.30 g, 4.69 mmol) in MeOH (26 mL) at room temperature and stirred for 24 h. Then the reaction mixture was washed with toluene. The addition of 12% aqueous sodium hydroxide created basic conditions. The mixture was extracted with Et2O three times. The combined organic layer was washed with brine and dried over anhydrous MgSO4. After filtration, the solvent was removed under reduced pressure. Resulting colorless oil 11 (819 mg, 4.60 mmol, 98%) was used in the next step without further purification.
1H-NMR (CDCl3, 400MHz) δ 1.84 (2H, br, s), 2.57 (1H, dd, J = 7.8, 13.2 Hz), 2.78 (1H, dd, J = 6.2, 13.2 Hz), 3.56 (1H, dd, J = 6.2, 13.2 Hz), 3.79 (3H, s), 5.05 (1H, d, J = 10.4 Hz), 5.14 (1H, d, J = 17.0 Hz), 5.88 (1H, ddd, J = 6.2, 10.4, 17.0 Hz), 6.84 (2H, d, J = 8.6 Hz), 7.12 (2H, d, J = 8.6 Hz).
8-{Acetyl[(2R)-1-(4-methoxyphenyl)but-3-en-2-yl]amino}-N-(4-methoxyphenyl)-1,4-dioxaspiro[4.5]decane-8-carboxamide (21).
Acetic acid 10 (0.48 mL, 8.32 mmol) was added to a solution of ketone 9 (1.08 g, 6.92 mmol), amine 11 (1.47 g, 8.29 mmol), and isonitrile 12 (1.39 g, 10.4 mmol) in MeOH (30 mL) at room temperature. The solution stood at room temperature for 3.5 days. The mixture was concentrated under reduced pressure, and then the residual mixture was purified by silica gel chromatography (AcOEt:n-hexane=1:2 to 1:1) to give 21 (2.70 g, 53.6 mmol, 77% from ketone 9) as a thick, pale brown amorphous solid. [a]D20.5 –17.1 (c 0.46, CHCl3); 1H-NMR (CDCl3, 500 MHz) δ 1.64 - 1.67 (3H, m), 1.86 (1H, quint, J = 7.4 Hz), 2.06 - 2.16 (4H, m), 2.20 (3H, s), 2.54 (1H, br. s), 3.06 (1H, dd, J = 7.4, 13.1 Hz), 3.32 (1H, br. s), 3.67 (3H, s), 3.77 (3H, s), 3.92 (4H, s), 4.48 (1H, br. s), 5.24 (2H, dd, J = 11.4, 15.1 Hz), 6.15 - 6.29 (1H, m), 6.80 (2H, d, J = 8.9 Hz), 6.82 (2H, d, J = 8.9 Hz), 7.14 (4H, d, J = 8.9 Hz); 13C-NMR (CDCl3, 100MHz) δ 14.0, 20.9, 25.6, 31.4, 31.8, 39.5, 54.9, 55.3, 60.2, 64.0, 64.1, 66.3, 107.5, 113.7, 114.1, 116.8, 122.9, 130.1, 138.1, 156.4, 158.3, 171.0, 174.2; IR (firm) 3379, 2935, 2247, 1649, 1513, 1248, 1107, 1035 cm-1; HRMS (ESI) calcd for C29H36N2NaO6 531.2478 [(M+Na)+], found 531.2473.
Methyl 1-{acetyl[(2R)-1-(4-methoxyphenyl)but-3-en-2-yl]amino}-4,4-dimethoxycyclohexane carboxylate (22).
Racemic camphor sulfonic acid (1.20 g, 5.31 mmol) was added to a stirred solution of 21 (2.70 g, 5.31 mmol) in MeOH (20 mL) and trimethyl orthoformate (20 mL) at room temperature. After a stirring for 16 h, the solvent was removed under reduced pressure. Aqueous NaHCO3 was added to the residual mixture, and then the mixture was extracted with AcOEt twice. The combined organic layer was washed with brine and dried over anhydrous MgSO4. The solvent was removed under reduced pressure. The resulting mixture was purified by silica gel chromatography (AcOEt:n-hexane=1:2) to give 22 (1.90 g, 4.53 mmol, 85%) as a colorless crystal. mp 107 °C; [α]D25.6 3.28 (c 1.04, CHCl3); 1H-NMR (CDCl3, 400MHz) δ 1.31 (1H, m), 1.66 (1H, m), 2.00-2.20 (4H, m), 2.15 (3H, s), 3.00-3.30 (2H, m), 3.10 (3H, br. s), 3.71 (3H, s), 3.80 (3H, s), 4.41 (1H, br. s), 5.28 (1H, m), 6.02 (1H, m), 6.88 (2H, d, J = 8.36 Hz), 7.15 (2H, d, J = 8.36 Hz); 13C NMR (CDCl3, 100MHz) δ 25.0, 28.4, 28.6, 28.8, 28.8, 40.3, 47.2, 47.6, 51.6, 51.9, 57.5, 65.0, 98.6, 114.0, 113.9, 115.9, 129.9, 130.0, 130.4, 137.5, 158.2, 172.2, 173.7; IR (firm) 2950, 2832, 1739, 1641, 1514, 1249, 1107 cm-1; HRMS (ESI): calcd for C23H33NNaO6 442.2206 [(M+Na)+], found 442.2206.
8,8-Dimethoxy-1-[(2S)-1-(4-methoxyphenyl)but-3-en-2-yl]-1-azaspiro[4.5]decane-2,4-dione (28).
1.6 M Lithium hexamethyldisilazide in THF (2.81 mL, 4.50 mmol) was added to a solution of 22 (629 mg, 1.50 mmol) in THF (7 mL) at –78 °C under argon atmosphere, and was stirred for 2 h before being allowed to warm to room temperature. Then aqueous NH4Cl was added. The mixture was extracted with AcOEt three times. From the combined organic layer, the solvent was removed under reduced pressure. The residual mixture was purified by silica gel chromatography (AcOEt:n-hexane=1:2) to give 28 (552 mg, 1.43 mmol, 95%) as a yellow oil. [α]D26.4 6.67 (c 1.33, CHCl3); 1H-NMR (CDCl3, 400MHz) δ 0.62 (1H, br. d, J = 14.0 Hz), 1.23 (1H, m), 1.52 (1H, br. d, J = 13.3 Hz), 1.74-1.89 (3H, m), 3.03 (2H, s), 3.13 (3H, s), 3.16 (3H, s), 3.55-3.78 (2H, m), 3.78 (3H, s), 5.09-5.30 (2H, m), 6.34 (1H, ddd, J = 6.9, 10.3, 17.2 Hz), 6.82 (2H, d, J = 8.48 Hz), 7.11 (2H, d, J = 8.48 Hz); 13C-NMR (CDCl3, 100MHz) δ 26.6, 27.4, 27.5, 27.9, 37.2, 40.4, 47.2, 47.6, 54.9, 58.9, 69.1, 98.1, 114.0, 113.5, 116.0, 130.3, 130.3, 136.5, 158.1, 167.8, 208.3; IR (firm) 2949, 2832, 1477, 1758, 1691, 1513, 1307, 1248 cm-1; HRMS (ESI) calcd for C22H29NNaO5 410.1943 [(M+Na)+], found 410.1941.
1-[(2S)-1-(4-Methoxyphenyl)but-3-en-2-yl]-1-azaspiro[4.5]decane-2,8-dione (31).
Sodium borohydride (2.00 g, 52.9 mmol) was added to a stirred solution of 28 (4.10 g, 10.6 mmol) in MeOH (100 mL) at room temperature. After stirring for 24 h, aqueous NH4Cl was added, and the resulting mixture was extracted with AcOEt twice. The combined organic layer was washed with brine and dried over anhydrous MgSO4. The solution was removed under reduced pressure. The resulting crude product was used in the next step without further purification.
To a stirred solution of the crude product from the previous step, phosphoryl chloride (3.30 g, 21.6 mmol) in pyridine was added at room temperature. The mixture was heated at 60 °C, and stirred for 2 h. The mixture was allowed to cool to room temperature, aqueous NaHCO3 was added, and then the mixture was extracted with AcOEt twice. The combined organic layer was washed with brine and dried over anhydrous MgSO4. The solvent was removed under reduced pressure. The resulting crude product was used in the next step without further purification.
Mg metal (4.20 g, 173 mmol) was added to a stirred solution of the crude product in MeOH (100 mL) at room temperature. Until hydrogen gas was generated, the suspension was heated with a heat gun. After stirring for 5 h and confirming the metal tips disappeared, 3 N HCl was added at 0 °C. After stirring at room temperature for 2 h, the mixture was extracted with AcOEt twice. The combined organic layer was washed with brine and dried over anhydrous MgSO4. The solvent was removed under reduced pressure. The resulting mixture was purified by silica gel chromatography (AcOEt:n-hexane=1:1 to 2:1) to give 31 (2.27 g, 6.91 mmol, 66%) as a colorless oil. [α]D25.6 4.26 (c 0.99, CHCl3); 1H-NMR (CDCl3, 400 MHz) δ 0.76 (1H, dq, J = 3.2, 13.5 Hz), 1.47 (1H, dt, J = 4.8, 13.8 Hz), 1.72-1.78 (2H, m), 1.93-1.98 (2H, m), 2.19-2.12 (2H, m), 2.24-2.44 (4H, m), 2.81 (2H, s), 2.90 (1H, m), 3.55-3.63 (1H, m), 3.78 (3H, s), 5.03-5.11 (1H, m), 6.34 (1H, ddd, J = 7.2, 10.3, 17.4 Hz), 6.81 (2H, d, J = 11.6 Hz), 7.07 (2H, d, J = 11.6 Hz); 13C-NMR (CDCl3, 100 MHz) δ 28.3, 29.5, 33.3, 34.2, 37.1, 37.5, 51.1, 55.1, 58.9, 63.0, 113.5, 115.7, 130.4, 130.8, 137.0, 158.1, 174.3, 208.9; IR (firm) 2936, 1716, 1679, 1666, 1513, 1410, 1246 cm-1; HRMS (ESI) calcd for C20H25NNaO3 350.1732 [(M+Na)+], found 350.1745.
(2S)-2-(2,8-Dioxo-1-azaspiro[4.5]dec-1-yl)-3-(4-methoxyphenyl)propanal (5b).
Ozone gas was passed through a stirred solution of 31 (2.26 g, 6.90 mmol) in MeOH (40 mL) at –78 °C. After starting material 31 was no longer detected by TLC, argon gas was passed through the solution for a few minutes before Me2S (858 mg, 13.8 mmol) was added. The mixture was allowed to warm to room temperature, and then the solvent was removed under reduced pressure. Brine was added to the residual mixture, which was extracted with AcOEt twice. The solvent was removed under reduced pressure. The resulting crude product 5b (2.11 g, 6.41 mmol, 93%) was used in the next step without further purification. [α]D24.8 –48.9 (c 1.16, CHCl3); 1H-NMR (CDCl3, 400 MHz) δ 0.71 (1H, m), 1.27 (1H, dt, J = 5.0, 18.8 Hz), 1.81 (2H, dt, J = 5.4, 13.3 Hz), 2.13 (1H, m), 2.26 (1H, dd, J = 6.0, 14.7 Hz), 2.35-2.60 (5H, m), 3.30-3.50 (3H, m), 4.97 (3H, s), 6.83 (2H, d, J = 8.7 Hz), 7.08 (2H, d, J = 8.7 Hz), 9.65 (1H, s); 13C-NMR (CDCl3, 100 MHz) δ 28.7, 32.4, 33.7, 34.4, 34.5, 37.4, 37.5, 55.3, 62.1, 62.8, 114.0, 128.2, 129.0, 129.5, 130.6, 158.5, 175.1, 198.7, 208.3; IR (firm) 3382, 2934, 1477, 1666, 1511, 1248 cm-1; HRMS (ESI) calcd for C19H23NNaO4 352.1525 [(M+Na)+], found 352.1528.
(5S,6R,7R,10aR)-6-Hydroxy-5-(4-methoxybenzyl)tetrahydro-1H-7,10a-methanopyrrolo[1,2-a]azocine-3,8(2H,5H)-dione (4b).
Pyrrolidine (149 mg, 2.09 mmol) and acetic acid (503 mg, 8.38 mmol) were added to a stirred solution of 5b (2.30 g, 6.98 mmol) in AcOEt at 0 °C. The mixture was warmed to 20 °C, and stirred for 24 h. The solvent was removed under reduced pressure, and the residual mixture was purified by silica gel chromatography (AcOEt:n-hexane=2:1) to give 4b (1.38 g, 4.20 mmol, 60%) as a colorless crystal. mp 156.3 °C; [α]D23.7 –93.2 (c 1.18, CHCl3); 1H NMR (CDCl3, 400 MHz) δ 1.52 (1H, br. d, J = 13.5 Hz), 1.72-1.87 (6H, m), 2.05 (1H, dd, J = 3.6, 13.2 Hz), 2.18 (1H, m), 2.48-2.55 (4H, m), 2.81 (1H, s), 3.56 (1H, dd, J = 4.4, 14.0 Hz), 3.78 (3H, s), 3.71-3.80 (1H, m), 3.95 (1H, dd, J = 6.2, 8.5 Hz), 6.82 (2H, d, J = 8.5 Hz), 7.18 (2H, d, J = 8.5 Hz); 13C NMR (CDCl3, 100 MHz) δ 31.2, 32.2, 33.4, 35.2, 35.8, 38.1, 51.1, 55.1, 59.5, 59.6, 70.4, 113.7, 129.4, 131.0, 158.1, 175.5, 211.1; IR (firm) 3382, 2934, 1666, 1511, 1248 cm-1; HRMS (ESI) calcd for C19H24NNaO4 352.1525 [(M+Na)+], found 352.1538.
(5S,6R,7S,10aR)-6,8-Dihydroxy-5-(4-methoxybenzyl)octahydro-3H-7,10a-methanopyrrolo[1,2-a]azocin-3-one (36).
Sodium triacetoxyborohydride (45.9 mg, 217 μmol) was added to a stirred solution of 4b (23.8 mg, 72.3 μmol) in MeCN (2 mL) and acetic acid (1 mL) at 0 °C. After stirring for 1 h, brine was added. The mixture was extracted with AcOEt twice. The combined organic layer was washed with brine and dried over anhydrous MgSO4. The solvent was removed under reduced pressure. The residual mixture was purified by silica gel thin layer chromatography (AcOEt:MeOH=10:1) to give 36 (14.9 mg, 45.0 µmol, 62%) as a colorless crystal. mp 197.9 °C; [α]D22.7 75.3 (c 0.75, MeOH); 1H-NMR (CDCl3, 400 MHz) δ 1.02 (1H, br. d, J = 13.0 Hz), 1.57 (1H, m), 1.73-1.80 (8H, m), 2.16 (1H, dd, J = 4.1, 13.0 Hz), 2.24 (1H, br. s), 2.36 (1H, dd, J = 9.4, 17.4 Hz), 2.51 (1H, m), 3.10 (1H, m), 3.78 (3H, s), 3.75-3.90 (1H, m), 3.86-4.00 (2H, m), 4.26 (1H, br. s), 6.84 (2H, d, J = 8.5 Hz), 7.18 (2H, d, J = 8.5 Hz); 13C-NMR (CDCl3, 100 MHz) δ 28.6, 31.2, 31.3, 31.6, 33.4, 36.6, 41.2, 44.6, 55.2, 59.9, 60.4, 60.7, 64.1, 72.0, 114.1, 129.5, 130.9, 158.3, 175.4; IR (firm) 3372, 2932, 1658, 1511, 1406, 1250 cm-1; HRMS (ESI) calcd for C19H26NNaO4 354.1681 [(M+Na)+], found 354.1670.
(5S,6R,7R,10aR)-8-{[tert-Butyl(dimethyl)silyl]oxy}-6-hydroxy-5-(4-methoxybenzyl)octahydro-3H-7,10a-methanopyrrolo[1,2-a]azocin-3-one (37).
Triethylamine (103 mg, 1.02 μmol) and TBS triflate (134 mg, 508 μmol) were added to a solution of 36 (112 mg, 339 μmol) in CH2Cl2 at –20 °C, which was stirred for 24 h. Aqueous NH4Cl was added. The mixture was allowed to warm to room temperature, and was extracted with AcOEt twice. The combined organic layer was washed with brine and dried over anhydrous MgSO4. The solvent was removed under reduced pressure. The residual mixture was purified by silica gel thin layer chromatography (AcOEt:n-hexane=1:2) to give 37 (96.7 mg, 216 µmol, 64%) as a colorless oil. [α]D20.4 32.7 (c 1.02, CHCl3); IR (firm) 2930, 1660, 1551, 1251, 1031 cm-1; 1H-NMR (CDCl3, 400 MHz) δ -0.03 (3H, s), -0.02 (3H, s), 0.84 (9H, s), 1.10 (1H, d, J = 12.8 Hz), 1.51-1.70 (7H, m), 1.76 (1H, dd, J = 8.2, 11.9 Hz), 2.13 (1H, br. s), 2.18 (1H, dd, J = 4.1, 12.8 Hz), 2.33 (1H, dd, J = 9.6, 17.4 Hz), 2.49 (1H, m), 2.80 (1H, m), 3.75 (3H, s), 3.70-3.85 (1H, m), 4.13 (1H, d, J = 3.2 Hz), 4.16 (1H, br. s), 6.83 (2H, d, J = 7.6 Hz), 7.18 (2H, d, J = 7.6 Hz); 13C-NMR (CDCl3, 100 MHz): δ -5.1, -4.9, 18.0, 25.7, 29.1, 31.2, 31.6, 31.7, 33.5, 37.6, 41.7, 55.1, 60.3, 60.8, 64.6, 72.8, 114.2, 129.7, 130.8, 158.4, 175.4; IR (firm) 2930, 1660, 1551, 1251, 1031 cm-1; HRMS (ESI) calcd for C25H39NNaO4Si 468.2546 [(M+Na)+], found 468.2532.
(5S,7S,10aR)-8-{[tert-Butyl(dimethyl)silyl]oxy}-5-(4-methoxybenzyl)tetrahydro-1H-7,10a-methanopyrrolo[1,2-a]azocine-3,6(2H,5H)-dione (3).
Oxalyl chloride (281 mg, 2.21 mmol) was added to a solution of DMSO (230 mg, 2.94 mmol) at –78 °C, which was stirred for 20 min. Then a solution of 37 (98.5 mg, 221 μmol) and triethylamine (816 mg, 8.07 mmol) was added. The mixture was warmed to 0 °C and stirred for an additional 10 min before aqueous NH4Cl was added. The mixture was allowed to warm to room temperature, and was extracted with AcOEt twice. The combined organic layer was washed with brine and dried over anhydrous MgSO4. The solvent was removed under reduced pressure. The residual mixture was purified by silica gel thin layer chromatography (CH2Cl2:MeOH=10:1) to give 3 (91.6 mg, 206 µmol, 93%, 96.4% ee) as a colorless oil. [α]D23.6 32.6 (c 0.91, CHCl3); 1H-NMR (CDCl3, 400 MHz) δ 0.00 (3H, s), 0.00 (3H, s), 0.12 (1H, br. d, J = 8.5 Hz), 0.81 (9H, s), 1.27 (1H, m), 1.51 (2H, m), 1.69-1.86 (5H, m), 2.18 (1H, m), 2.24 (1H, br. s), 2.40 (1H, dd, J = 9.2, 17.4 Hz), 2.60 (1H, ddd, J = 8.2, 12.4, 17.0 Hz), 3.08 (1H, dd, J = 2.8, 13.7 Hz), 3.75 (3H, s), 3.80-3.75 (1H, m), 3.96 (1H, m), 4.52 (1H, br. s), 6.76 (2H, d, J = 8.5 Hz), 6.86 (2H, d, J = 8.5 Hz); 13C-NMR (CDCl3, 100 MHz) δ -5.1, -5.1, 17.9, 25.6, 26.9, 28.9, 30.9, 31.5, 34.1, 34.1, 35.2, 51.7, 55.2, 59.4, 64.2, 68.0, 113.8, 128.7, 131.0, 158.7, 174.5, 210.1; IR (firm) 2934, 1687, 1512, 1396, 1251, 1028 cm-1; HRMS (ESI) calcd for C25H37NNaO4Si 466.2390 [(M+Na)+], found 466.2401.
(5S,6S,7R,10aR)-8-{[tert-Butyl(dimethyl)silyl]oxy}-6-hydroxy-5-(4-methoxybenzyl)octahydro-3H-7,10a-methanopyrrolo[1,2-a]azocin-3-one (38).
Diiodomethane (0.57 mL, 7.1 mmol) was added to a suspension of Sm0 (1.28 g, 8.52 mmol) in THF (71 mL) at –78 °C. After stirring at room temperature for 16 h, a degassed mixture of MeOH (0.58 mL, 14.2 mmol), HMPA (6.2 mL, 35.5 mmol), and THF (30 mL) was added. Then key intermediate 3 (630 mg, 1.42 mmol) in degassed THF (9 mL) was added dropwise at –78 °C, and the reaction was stirred overnight. Saturated aqueous sodium bicarbonate was added at room temperature, and the mixture was extracted with AcOEt (three times). The combined organic layer was washed with brine, and then dried over MgSO4. The solvent was removed under reduced pressure. The resulting residue was purified by silica gel chromatography (AcOEt:n-hexane=4:1-1:1) to afford crude alcohol 38 (553 mg) containing inseparable HMPA, which was used in the next reaction without further purification.
(5S,6S,7S,10aR)-6,8-bis{[tert-Butyl(dimethyl)silyl]oxy}-5-(4-methoxybenzyl)octahydro-3H-7,10a-methanopyrrolo[1,2-a]azocin-3-one (39).
2,6-Lutidine (0.4 mL, 3.7 mmol) followed by TBSOTf (0.6 mL, 2.5 mmol) were added to a stirred solution of the crude product of 38 (554 mg, 1.2 mmol) in CH2Cl2 (10 mL) at room temperature. After stirring at room temperature for 5 h, saturated aqueous ammonium chloride was added and the mixture was extracted with CH2Cl2 (three times). The combined organic layer was dried over MgSO4. The solvent was removed under reduced pressure. The resulting residue was purified by silica gel chromatography (AcOEt:n-hexane=5:95) to afford pure product 39 (360 mg, 0.664 mmol, 54% for 2 steps) as a pale yellow oil. [α]D22.0 18.3 (c 1.03, CHCl3); 1H-NMR (CDCl3, 500 MHz) δ 0.00 (3H, s), 0.01 (3H, s), 0.02 (1H, s), 0.02 (1H, s), 0.87 (9H, s), 0.92 (9H, s), 1.59-1.83 (7H, m), 1.86-1.94 (1H, m), 2.28-2.42 (2H, m), 3.11 (1H, dd, J = 7.9, 14.7 Hz), 3.62-3.65 (1H, m), 3.75-3.78 (1H, m), 3.79 (3H, s), 3.85 (1H, dd, J = 5.1, 14.2 Hz), 4.04-4.09 (1H, m), 6.82 (2H, d, J = 8.5 Hz), 7.21 (2H, d, J = 8.5 Hz); 13C-NMR (CDCl3, 68 MHz): δ –4.9, –4.8, –4.7, –4.1, 18.0, 18.3, 25.7, 26.0, 27.3, 29.5, 31.1, 32.1, 33.7, 34.0, 45.4, 55.2, 58.6, 60.6, 67.5, 69.4, 113.6, 130.2, 132.6, 157.7, 176.1; IR (firm) 2953, 2930, 2857, 1693, 1513, 1250, 1077, 1053 cm-1; HRMS (FAB) calcd for C31H53NO4Si2 559.3513 (M+), found 559.3528.
(5S,6S,7S,10aS)-6,8-bis{[tert-Butyl(dimethyl)silyl]oxy}-5-(4-methoxybenzyl)-3-oxooctahydro-1H-
7,10a-methanopyrrolo[1,2-a]azocine-2-carboxylic acid (40).
n-BuLi (2.0 M in hexane)(4.8 mL, 12.9 mmol) was added to a solution of diisopropylamine (2.3 mL, 16.1 mmol) in THF (15 mL) at –78 °C. The resulting solution was stirred at 0 °C for 20 min before cooling to –78 °C. Then a solution of 39 (600 mg, 1.07 mmol) in THF (5 mL) was added dropwise. After stirring for 1 h at –78 °C, crushed dry-ice blocks were added until dry-ice solids were observed. After stirring at –78 °C for 20 min, saturated aqueous ammonium chloride was added, and the mixture was extracted with AcOEt (six times). The combined organic layer was dried over Na2SO4. The solvent was removed under reduced pressure. The resulting residue was washed with hexane and filtered over a pad of Celite. The filtrate was concentrated under reduced pressure to give the crude product of 40 (672 mg), which was used in the next reaction without further purification.
(2Z,5S,6S,7S,10aS)-6,8-Bis{[tert-butyl(dimethyl)silyl]oxy}-2-(hydroxyimino)-5-(4-methoxybenzyl)octahydro-3H-7,10a-methanopyrrolo[1,2-a]azocin-3-one (41).
NaNO2 (1.2 g, 17.0 mmol) was added to a stirred solution of the crude product of 40 (100 mg) and tetra-n-butylammonium bromide in 5:1 Et2O:H2O (2.4 mL) at 0 °C, and then conc. HCl (0.25 mL, 3.00 mmol) was added cautiously in four portions over 9 h. Saturated aqueous ammonium chloride was added. The mixture was stirred for an additional 1 h before extracting with AcOEt (four times). The combined organic layer was dried over MgSO4. The solvent was removed under reduced pressure. The resulting residue was purified by silica gel chromatography (AcOEt:n-hexane=7:1 to 4:1) to afford pure oxime 41 (64 mg, 115 µmol, 66% for 2 steps) as a pale orange solid. [α]D24.0 16.8 (c 1.00, CHCl3); 1H-NMR (CDCl3, 400 MHz) δ 0.01-0.04 (12H, m), 0.86 (9H, s), 0.92 (9H, s), 1.60-2.08 (7H, m), 2.56 (2H, q, J = 18.0 Hz), 3.12 (1H, dd, J = 9.2, 15.6 Hz), 3.65 (1H, s), 3.78 (3H, s), 4.11-4.18 (2H, m), 6.82 (2H, d, J = 8.2 Hz), 7.20 (2H, d, J = 8.2 Hz), 9.42 (1H, br. s) 13C-NMR (CDCl3, 100 MHz): δ –5.0, –4.9, –4.8, –4.1, 18.0, 18.3, 25.7, 26.0, 28.8, 30.2, 32.7, 33.5, 37.1, 45.2, 55.2, 57.9, 60.1, 66.7, 68.9, 113.7, 130.0, 131.9, 152.8, 157.8, 163.9; IR (firm) 3269, 2953, 2930, 2857, 1708, 1660, 1513, 1251 cm–1 ; HRMS (FAB) calcd for C31H52N2O5Si2 588.3415 (M+), found 588.3410.
(2S,5S,6S,7S,10aS)-2-Amino-6,8-bis{[tert-butyl(dimethyl)silyl]oxy}-5-(4-methoxybenzyl)octahydro-3H-7,10a-methanopyrrolo[1,2-a]azocin-3-one (42).
Activated zinc dust (1.30 g, 19.4 mmol) and NH4Cl (520 mg, 9.7 mmol) were added to a stirred solution of oxime 41 (285 mg, 0.510 mmol) in AcOH (12 mL) at room temperature. After stirring at 50 °C overnight, the mixture was filtered over a pad of Celite, and the filtrate was concentrated under reduced pressure. To the resulting residue, saturated aqueous sodium bicarbonate was added, and the mixture was extracted with CH2Cl2 (three times). The combined organic layer was dried over Na2SO4. The solvent was removed under reduced pressure to give the crude product of 42 (297 mg), which was used in the next reaction without further purification. 1H-NMR (CDCl3, 400 MHz) δ –0.02-0.06 (12H, m), 0.85 (9H, s), 0.93 (9H, s), 1.61-2.07 (10H, m), 2.21 (1H, m), 3.13 (1H, dd, J = 7.3, 14.6 Hz), 3.46 (1H, dd, J = 8.7, 10.5 Hz), 3.51 (1H, s), 3.70 (1H, br), 3.79 (3H, s), 3.87 (1H, t, J = 6.9 Hz), 3.98 (2H, dd, J = 6.4, 14.7 Hz), 6.83 (2H, d, J = 8.2 Hz), 7.19 (2H, d, J = 8.2 Hz); 13C-NMR (CDCl3, 100 MHz) δ –5.0, –4.9, –4.8, –4.0, 18.0, 18.1, 25.7, 25.8, 26.4, 30.2, 32.6, 33.5, 42.7, 45.4, 52.6, 55.2, 57.2, 59.3, 67.2, 69.5, 113.7, 129.7, 132.4, 157.8, 176.7.
N-[(2S,5S,6S,7S,10aS)-6,8-Bis{[tert-butyl(dimethyl)silyl]oxy}-5-(4-methoxybenzyl)-3-oxooctahydro-1H-7,10a-methanopyrrolo[1,2-a]azocin-2-yl]formamide (45).
A mixture (3.8 mL) of HCOOH (6.0 mL, 157 mmol) and Ac2O (3.8 mL, 40 mmol) were added to a stirred solution of amine 42 (297 mg) in CH2Cl2 (15 mL) at 0 °C dropwise, and the solution was stirred for 10 min at 0 °C. Then the reaction mixture was warmed to room temperature, and a small amount of toluene was added. The solvent was removed under reduced pressure to give the crude product of 45 (323 mg). This unstable product was used immediately in the next reaction without further purification.
(2S,5S,6S,7R,10aS)-8-{[tert-Butyl(dimethyl)silyl]oxy}-5-(4-methoxybenzyl)-2-(methylamino)octahydro-1H-7,10a-methanopyrrolo[1,2-a]azocin-6-ol (46).
Lithium aluminum hydride (405 mg, 11 mmol) was added to a stirred solution of the crude product of 45 (323 mg) in THF (15 mL) at 0 °C. After stirring at 50 °C for 16 h, water (0.4 mL), 15% aqueous sodium hydroxide (0.4 mL), water (1.2 mL), and a sufficient amount of Et2O were added successively at room temperature. After stirring for 30 min, the mixture was filtered over a pad of Celite. The filtrate was concentrated under reduced pressure to give crude methylamine 46 (259 mg). This polar product was used in the next reaction without further purification.
Benzyl [(2S,5S,6S,7R,10aS)-8-{[tert-butyl(dimethyl)silyl]oxy}-6-hydroxy-5-(4-methoxybenzyl)octahydro-1H-7,10a-methanopyrrolo[1,2-a]azocin-2-yl]methylcarbamate (47).
Na2CO3 (488 mg, 4.6 mmol) followed by CbzCl (0.32 mL, 2.3 mmol) were added to a stirred solution of crude methylamine 46 (259 mg) in 3:1 CH2Cl2:H2O (16 mL) at room temperature. After stirring for 3 h, saturated aqueous ammonium chloride was added, and the mixture was extracted with CH2Cl2. The combined organic layer was dried over Na2SO4. The solvent was removed under reduced pressure. The resulting residue was purified by silica gel chromatography (AcOEt:n-hexane=1:9 to 1:1) to afford pure alcohol 47 (174 mg, 0.293 mmol, 61% for 4 steps) as a pale yellow oil. [α]D24.0 7.65 (c 1.00, CHCl3); 1H-NMR (CDCl3, 500 MHz): δ -0.02 (3H, s), -0.01 (3H, s), 0.86 (9H, s), 1.47 (1H, dd, J = 6.2, 13.6 Hz), 1.52 – 2.12 (9H, m), 2.71 – 2.89 (6H, m), 3.19 – 3.29 (1H, m), 3.35 – 3.57 (2H, m), 3.72 (1H, br. s), 3.79 (3H, s), 4.83 (1H, br. s), 5.12 (2H, s), 6.84 (2H, d, J = 8.5 Hz), 7.21 (2H, d, J = 8.5 Hz), 7.28 - 7.39 (5H, m,); 13C-NMR (CDCl3, 68 MHz): δ -4.8, -4.7, 18.1, 25.9, 29.1, 29.8, 31.1, 35.9, 42.9, 45.9, 50.1, 52.1, 55.3, 59.0, 59.3, 67.2, 67.4, 67.7, 113.9, 128.0, 128.0, 128.6, 130.3, 131.0, 137.0, 156.5, 158.0; IR (firm) 3448, 2952, 2931, 1698, 1512, 1329, 1249, 1161, 1041 cm–1; HRMS (FAB) calcd for C34H51N2O5Si 595.3562 [(M+H)+], found 595.3531.
Benzyl [(2S,5S,6S,7S,10aS)-6,8-dihydroxy-5-(4-methoxybenzyl)octahydro-1H-7,10a-methanopyrrolo[1,2-a]azocin-2-yl]methylcarbamate (48).
Aqueous HF (48%, 1.6 mL) was added to a stirred solution of alcohol 47 (174 mg, 0.293 mmol) in MeCN (9 mL) at 0 °C. After stirring at 0 °C for 5 h, the reaction mixture was diluted with CH2Cl2 and saturated aqueous sodium bicarbonate was added. The mixture was extracted with CHCl3 (six times), and the combined organic layer was dried over Na2SO4. The solvent was removed under reduced pressure to give the crude product of 48 (150 mg), which was used in the next reaction without further purification.
Benzyl[(2S,5S,6S,7R,10aS)-8-{[bis(benzyloxy)phosphoryl]oxy}-6-hydroxy-5-(4-methoxybenzyl)octahydro-1H-7,10a-methanopyrrolo[1,2-a]azocin-2-yl]methylcarbamate (49).
1-H-tetrazole (21 mg, 0.30 mmol) was added to a stirred solution of diol 48 (50 mg) in CH2Cl2 (4 mL) at 0 °C, and the mixture was stirred for 20 min. Then dibenzyl(N,N-diisopropyl)phosphoramidite (90%)(ca. 58 µL, 0.20 mmol) was added slowly until the starting material was no longer detected by TLC analysis. Then the reaction mixture was cooled to –78 °C, and TBHP (5.5 M in decane) (40 µL) was added. Prior to the addition of saturated aqueous Na2SO3, the mixture was stirred for 1 h at –78 °C. Then the mixture was extracted with CH2Cl2, and the combined organic layer was dried over Na2SO4. The solvent was removed under reduced pressure. The resulting residue was purified by silica gel chromatography (AcOEt:n-hexane=2:3 to 5:1) and silica gel thin layer chromatography (AcOEt:n-hexane=3:1) to afford pure dibenzyl phosphate 49 (25 mg, 34 µmol, 35% for 2 steps) as a colorless oil. [α]D23.0 6.69 (c 1.70, CHCl3); IR (firm) 3408, 2935, 1695, 1512, 1454, 1248, 1009 cm–1; 1H-NMR (CD3OD, 500 MHz) δ 1.22 – 1.52 (2H, m), 1.61 - 2.11 (6H, m), 2.26 (1H, br. s), 2.70 (3H, s), 2.75 – 2.98 (3H, m), 3.26 (1H, br. s), 3.38 (1H, br. s), 3.54 – 3.72 (1H, m), 3.74 (3H, s), 4.31 (1H, br. s), 4.72 (1H, br. s), 4.96 – 5.04 (4H, m), 5.08 (2H, s), 6.84 (2H, d, J = 8.6 Hz), 7.21 (2H, d, J = 8.6 Hz), 7.27 - 7.40 (m, 15H); 13C-NMR (CD3OD, 68 MHz) δ 23.0, 29.3, 29.6, 31.3, 36.3, 44.1, 44.8, 51.7, 53.4, 55.7, 59.5, 68.0, 68.3, 70.8 (d, J = 6.0 Hz), 77.1, 114.7, 128.8, 129.1, 129.2, 129.6, 129.8, 131.2, 132.4, 137.2 (d, J = 6.0 Hz), 137.2 (d, J = 6.0 Hz), 138.2, 158.0, 159.6; HRMS (FAB) calcd for C42H50N2O8P 741.3299 [(M+H)+] found 741.3298.
(–)-FR901483 (1).
Aqueous 1 N HCl (18 μL) was added to a solution of dibenzyl phosphate 49 (34 mg, 46 μmol) in MeOH (3 mL), and the solvent was removed under reduced pressure giving 1·HCl. Pd/C (5%, wet) (30 mg) and MeOH (4 mL) were added. The mixture was stirred at room temperature under 1 atm of hydrogen gas for 6 h and filtered. The residue was washed with MeOH. The combined filtrate was concentrated under reduced pressure to give FR901483 (1) (15 mg, 35.2 mmol, 77%) as a white solid. [α]D23.0 -10.3 (c 0.68, CHCl3); 1H-NMR (CD3OD, 500 MHz) δ 1.84 - 1.92 (1H, m), 2.01 - 2.28 (6H, m), 2.43 (1H, br. s), 2.51 - 2.59 (1H, m), 2.68 (3H, s), 2.97 - 3.06 (1H, m), 3.21 - 3.28 (1H, m), 3.57 (1H, br. s), 3.73 - 3.84 (2H, m), 3.78 (3H, s), 4.09 - 4.24 (2H, m), 4.42 (1H, dd, J = 9.7, 13.2 Hz), 6.88 (2H, d, J = 8.0 Hz), 7.32 (2H, d, J = 8.0 Hz); 13C-NMR (CD3OD, 68 MHz) δ 22.5, 28.1, 28.5, 32.3, 34.1, 42.0, 43.0, 51.8, 55.0, 55.7, 61.5, 64.3, 67.8, 70.6 (d, J = 6.0 Hz), 115.2, 128.9, 131.7, 160.3; IR (firm) 3336, 2933, 1612, 1514, 1458, 1248, 1180, 1009 cm–1; HRMS (FAB) calculated for C20H32N2O6P 427.1992 [(M+H)+] found 427.1996.
ACKNOWLEDGEMENTS
The authors thank Mr. Koji Nagao (Astellas Pharma Inc.) for the sample of natural product 1. This work was financially supported by CREST, JST, and a Grant-in-Aid from the Ministry of Education, Science, Sports, Culture and Technology of Japan.
References
1. K. Sakamoto, E. Tsujii, F. Abe, T. Nakanishi, M. Yamashita, N. Shigematsu, S. Izumi, and M. Okuhara, J. Antibiot., 1996, 49, 37. CrossRef
2. For a review of biomimetic syntheses of this family compounds, see: H. Takayama, J. Synth. Org. Chem. Jpn., 2002, 60, 350. CrossRef
3. For synthetic studies, see: (a) T. Fujimoto, H. Kitaoka, S. Ieda, T. Kan, and T. Fukuyama, Abstract of papers, 79th Symposium on Organic Synthesis, Japan; The Society of Synthetic Organic Chemistry, Japan: Tokyo, June 2001, 17; (b) H. Suzuki, N. Yamazaki, and C. Kibayashi, Tetrahedron Lett., 2001, 42, 3013; CrossRef (c) K. M. Brummond and J. L. Lu, Org. Lett., 2001, 3, 1347; CrossRef (d) D. J. Wardrop and W. M. Zhang, Org. Lett., 2001, 3, 2001; CrossRef (e) G. Puigbo, F. Diaba, and J. Bonjoch, Tetrahedron, 2003, 59, 2657; CrossRef (f) J. Bonjoch, F. Diaba, G. Puigbo, E. Peidro, and D. Sole, Tetrahedron Lett., 2003, 44, 8387; CrossRef (g) J. E. Kropf, I. C. Meigh, M. W. P. Bebbington, and S. M. Weinreb, J. Org. Chem., 2006, 71, 2046; CrossRef (h) S. Kaden and H.-U. Reissig, Org. Lett., 2006, 8, 4763; CrossRef (i) D. B. Gotchev and D. L. Comins, J. Org. Chem., 2006, 71, 9393; CrossRef (j) F. Diaba, E. Ricou, D. Sole, E. Teixido, N, Valls, and J. Bonjoch, ARKIVOC, 2007, iv, 320; (k) A. Asari, P. Angelov, J. M. Auty, and C. J. Hayes, Tetrahedron Lett., 2007, 48, 2631; CrossRef (l) S. T. Simila and S. F. Martin, J. Org. Chem., 2007, 72, 5342; CrossRef (m) H. Seike and E. J. Sorensen, Synlett, 2008, 695; CrossRef (n) S. Ieda, T. Kan, and T. Fukuyama, Tetrahedron Lett., 2010, 51, 4027. CrossRef
4. For total syntheses, see: (a) B. B. Snider and H. Lin, J. Am. Chem. Soc., 1999, 121, 7778; CrossRef (b) G. Scheffler, H. Seike, and E. J. Sorensen, Angew. Chem. Int. Ed., 2000, 39, 4593; CrossRef (c) M. Ousmer, N. A. Braun, C. Bavoux, M. Perrin, and M. A. Ciufolini, J. Am. Chem. Soc., 2001, 123, 7543; CrossRef (d) J. Maeng and R. L. Funk, Org. Lett., 2001, 3, 1125; CrossRef (e) T. Kan, T. Fujimoto, S. Ieda, Y. Asoh, H. Kitaoka, and T. Fukuyama, Org. Lett., 2004, 6, 2729; CrossRef (f) K. M. Brummond and S. Hong, J. Org. Chem., 2005, 70, 907; CrossRef (g) C. A. Carson and M. A. Kerr, Org. Lett., 2009, 11, 777; CrossRef (h) S. Ieda, Y. Asoh, T. Fujimoto, H. Kitaoka, T. Kan, and T. Fukuyama, Heterocycles, 2009, 79, 721. CrossRef
5. For a review of organo-catalyst mediated aldol reactions, see: (a) S. Saito and H. Yamamoto, Acc. Chem. Res., 2004, 37, 570; CrossRef Some examples in intramolecular aldol reactions, see: (b) Y. Hayashi, H. Sekizawa, and J. Yamaguchi, J. Org. Chem., 2007, 72, 6493; CrossRef (c) N. Itagaki, M. Kimura, T. Sugawara, and Y. Iwabuchi, Org. Lett., 2005, 7, 4185. CrossRef
6. For a review of enantioselective desymmetrization, see: M. C. Willis, J. Chem. Soc., Perkin Trans. 1, 1999, 1765. CrossRef
7. For reviews of multicomponent reactions with isonitriles, see: (a) C. Hulme and V. Gore, Curr. Med. Chem., 2003, 10, 51; CrossRef (b) A. Dömling and I. Ugi, Angew. Chem. Int. Ed., 2000, 39, 3168; CrossRef (c) G. Gokel, G. Lüdke, and I. Ugi, 'Isonitrile Chemistry' ed. by I. Ugi, Academic Press, Inc., New York, 1971, 145; (d) 'Multicomponent Reactions' ed. by J. Zhu, H. Bienaymé, Wiley-VCH Verlag GmbH & Co. KGaA, Weinheim 2005.
8. G. W. Gokel, R. P. Widera, and W. P. Weber, Org. Synth., Coll. Vol. VI, 1988, 232.
9. The conversion of 15 to 22 by DIBAL reduction and the subsequent Wittig olefination resulted in racemic 11. Instead of a Wittig olefination, a Nozaki-Takai olefination (Zn, CH2I2, AlMe3 in THF) was effectively conducted without racemization. However, the Nozaki-Takai olefination was unsuited for large-scale preparation due to its uncontrollable exothermic behavior.
10. US patent 5929284, 1997.
11. (a) K. Rikimaru, A. Yanagisawa, T. Kan, and T. Fukuyama, Heterocycles, 2007, 73, 403; CrossRef (b) K. Rikimaru, A. Yanagisawa, T. Kan, and T. Fukuyama, Synlett, 2004, 41. CrossRef
12. T. A. Spencer, K. K. Schmiegel, and K. L. Williamson, J. Am. Chem. Soc., 1963, 85, 3785. This well-known classic condition was rediscovered in Solensen’s work,3m although it exclusively gave the “undesired” stereoisomer in their synthetic studies. CrossRef
13. Similar stereochemistry of the aldol product was observed with 4a. Both the hydroxy groups of 3a and 3b were equatorially oriented and syn to the carbonyl groups.
14. D. A. Evans, K. T. Chapman, and E. M. Carreira, J. Am. Chem. Soc., 1988, 110, 3560. CrossRef
15. Synthetic protocol based on NaBH(OAc)3 reduction, and selective deprotection of the TBS ether has already been reported in ref 4d. However, the transformation of 5 to 18 was developed independently in our laboratory, see ref 3a.
16. For a recent review of SmI , see: (a) G. A. Molander and C. R. Harris, Chem. Rev., 1996, 96, 307; CrossRef (b) G. A. Molander, Chem. Rev., 1992, 92, 29. CrossRef
17. Enhancement of the reduction potency of SmI2 by the addition of HMPA has been reported, see: J. Inanaga and M. Yamaguchi, J. Synth. Org. Chem. Jpn., 1989, 47, 200. CrossRef
18. For a review of the phosphoramidite method, see: R. P. Iyer, Tetrahedron, 1992, 48, 2223. CrossRef
19. For a similar regioselective phosphitylation, see ref 4a.
20. Eluted with 1% EtOH-n-hexane at flow rate of 1.0 mL/min on Daicel Chemical Ind., Ltd. CHIRALCEL OJ column (0.46 cm × 25 cm). Retention time: (–)- = 9.7 min, (+)- = 10.5 min.