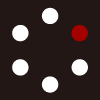
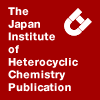
HETEROCYCLES
An International Journal for Reviews and Communications in Heterocyclic ChemistryWeb Edition ISSN: 1881-0942
Published online by The Japan Institute of Heterocyclic Chemistry
e-Journal
Full Text HTML
Received, 10th August, 2012, Accepted, 25th September, 2012, Published online, 5th October, 2012.
DOI: 10.3987/COM-12-S(N)107
■ ISOLATION AND FIRST X-RAY STRUCTURES OF NICKEL COMPLEXES OF 1,2,5-THIADIAZOLE-3,4-DITHIOLATE (TDAS) IN PROTONATED FORMS
Kouzou Matsumoto,* Maho Nishizawa, Yasukazu Hirao, Hiroyuki Kurata, and Takashi Kubo*
Department of Chemistry, Graduate School of Science, Osaka University, Toyonaka, Osaka 560-8531, Japan
Abstract
Protonated Ni(tdas)2 complexes, tdas = 1,2,5-thiadiazole-3,4-dithiolate, are isolated and characterized by X-ray crystallography. pH-dependent absorption measurements reveal high proton donor ability of Ni(Htdas)2.Metal bis(dithiolene) complexes have attracted intense interest as electro-conductive and magnetic materials.1 Within the family of dithiolene ligands 1,3-dithiole-2-thione-4,5-dithiolato (dmit), maleonitrile-2,3-dithiolato (mnt), and 5,6-dihydro-1,4-dithiin-2,3-dithiolate (dddt) are widely used. The 1,2,5-thiadiazole-3,4-dithiolate (tdas) ligand has been also intensively studied as M(tdas)2 complexes (M = Ni, Pd, Pt, Fe, Co, Cu, Au) in terms of potential application of functional materials 2,3 since the first isolation as a nickel complex.2a The tdas ligand possesses an electron-deficient heteroaromatic ring, and consequently, stable redox states of M(tdas)2 are dianion and monoanion states, while a neutral state appears to be difficult to generate according to the cyclic voltammogram that shows the irreversible anodic wave during [Ni(tdas)2]− → [Ni(tdas)2]0 oxidation process.2j Indeed the neutral complex of M(tdas)2 has not been isolated yet. Therefore, the use of M(tdas)2 has been limited to anion counterparts of charge transfer complexes or salts. On the other hand, the tdas ligand should exist in a protonated form as shown in Scheme 1, which could lead to a neutral form of the M(tdas)2 systems. This not only expands the possibility of M(tdas)2 for electro-conductive and magnetic materials, but also leads to a new function such as ferroelectricity 4 because a protonated M(tdas)2 is expected to behave as a good proton donor. Herein we report the first isolation and characterization of protonated Ni(tdas)2 complexes.
In order to obtain protonated tdas metal complexes, we thoroughly investigated the protonation of [Ni(tdas)2]2–.2b After many trials, we could find the appropriate reaction conditions that a di-protonated complex Ni(Htdas)2 and a mono-protonated complex [Ni(Htdas)(tdas)]– can be selectively obtained by changing the counter ions of [Ni(tdas)2]2–. For the di-protonated complex Ni(Htdas)2, trifluoroacetic acid (TFA) was added to a solution of (Et4N)2[Ni(tdas)2] in acetonitrile.5 Ni(Htdas)2 was obtained as a deep purple solid. Elemental analysis and IR measurement of the solid fully supported the exclusive formation of di-protonated species. Single crystals of Ni(Htdas)2 suitable for X-ray crystallographic analysis were obtained by the addition of concentrated nitric acid to an aqueous solution of (Et4N)2[Ni(tdas)2]. Other recrystallization conditions (TFA or hydrochloric acid in acetonitrile, water, or N,N-dimethylformamide) gave only a microcrystalline solid or decomposed products. The mono-protonated complex (n-Bu4N)[Ni(Htdas)(tdas)] was obtained as red prisms by the addition of TFA into an acetonitrile solution of (n-Bu4N)2[Ni(tdas)2].6 The selective formation of (n-Bu4N)[Ni(Htdas)(tdas)] is due to the lower solubility of (n-Bu4N)[Ni(Htdas)(tdas)] than Ni(Htdas)2 in acetonitrile. Elemental analysis and IR measurement of the red prisms supported the selective formation of mono-protonated species.
The X-ray structure of Ni(Htdas)2 is shown in Figure 1.7 Ni(Htdas)2 forms a N–H···O hydrogen bond (2.721 Å) with a water molecule (Figure 1a). Contrary to the planar structure of [Ni(tdas)2]2–,2b,2d,2e,2f,2h,2i,2k-n Ni(Htdas)2 takes a “Z-shaped” structure (Figure 1b). The dihedral angle between the mean plane of Htdas and the central NiS4 plane is approximately 12.0°. There are some differences in bond lengths between Ni(Htdas)2 and [Ni(tdas)2]2–. The bond lengths of S2–C2 (1.698 (7) Å) and N2–C2 (1.333(8) Å) of Ni(Htdas)2 are shorter and longer than the corresponding bonds of [Ni(tdas)2]2–,8 due to their double and single bond character (see, Scheme 1), respectively. In crystal, Ni(Htdas)2 molecules stack along the a-axis and the stack columns are connected by hydrogen bonds with water molecules and by short S···S contacts between neighboring molecules (S2···S3; 3.175 Å, S1···S3; 3.341 Å).
Figure 2 shows the X-ray structure of (n-Bu4N)[Ni(Htdas)(tdas)].9 There are two crystallographically independent (n-Bu4N)[Ni(Htdas)(tdas)] molecules in the unit cell. Although the high R1-value (8.8%) prohibits detailed discussion on molecular geometries, both [Ni(Htdas)(tdas)]– takes almost similar structure with high planarity (Figure 2b). [Ni(Htdas)(tdas)]– forms a one-dimensional (1D) chain running along the [1 -1 0] direction (Figure 2c) through the N–H···N hydrogen bonds (N2···N6; 2.733 Å and N4···N8; 2.745 Å) between neighboring molecules. Short S···S contacts (S2···S9; 3.335 Å and S5···S12; 3.379 Å) were observed in the 1D chain, while there was no appreciable short S···S contact between the 1D chains. The sheet of [Ni(Htdas)(tdas)]– and that of tetrabutylammonium ion stack alternately along the c axis (Figure 3a).
As mentioned above, Ni(Htdas)2 is expected to behave as a good proton donor. In order to estimate the pKa values of Ni(Htdas)2 (Scheme 2), pH dependent absorption spectra of (n-Bu4N)2[Ni(tdas)2] were measured (Figure 3b).10 However, the pKa values of Ni(Htdas)2 could not be determined because the absorption bands of [Ni(tdas)2]2– did not completely disappear even when the pH of the solution was nearly zero. This result indicates that the Ni(Htdas)2 possesses the pKa values of less than zero and accordingly prominent proton donor ability.
In summary, we isolated nickel complexes with tdas ligands in protonated forms. Ni(Htdas)2 and [Ni(Htdas)(tdas)]– possess high proton donor ability due to the electron deficiency of the thiadiazole ring. Investigation on functional properties of Ni(Htdas)2 such as electro-conductivity and dielectricity are now in progress in our group.
ACKNOWLEDGEMENTS
This work was supported by a grant from the Iketani Science and Technology Foundation (0201096-A).
References
1. N. Robertson and L. Cronin, Coord. Chem. Rev., 2002, 227, 93. CrossRef
2. (a) I. Hawkins and A. E. Underhill, J. Chem. Soc., Chem. Commun., 1990, 1593; CrossRef (b) O. A. Dyachenko, S. V. Konovalikhin, A. I. Kotov, G. V. Shilov, E. B. Yagubskii, C. Faulmann, and P. Cassoux, J. Chem. Soc., Chem. Commun., 1993, 508; CrossRef (c) S. Schenk, I. Hawkins, S. B. Wilkes, A. E. Underhill, A. Kobayashi, and H. Kobayashi, J. Chem. Soc., Chem. Commun., 1993, 1648; CrossRef (d) H. Yamochi, N. Sogoshi, Y. Simizu, G. Saito, and K. Matsumoto, J. Mater. Chem., 2001, 11, 2216; CrossRef (e) T. Okuno, K. Kuwamoto, W. Fujita, K. Awaga, and W. Nakanishi, Polyhedron, 2003, 22, 2311; CrossRef (f) C. Ni, D. Dang, Z. Ni, Y. Li, J. Xie, Q. Meng, and Y. Yao, J. Coord. Chem., 2004, 57, 1529; CrossRef (g) S. Curreli, P. Deplano, M. L. Mercuri, L. Pilia, A. Serpe, J. A. Schlueter, M. A. Whited, U. Geiser, E. Coronado, C. J. Gómez-García, and E. Canadell, Inorg. Chem., 2004, 43, 2049; CrossRef (h) S. S. Staniland, W. Fujita, Y. Umezono, K. Awaga, S. Crawford, S. Parsons, and N. Robertson, Mol. Cryst. Liq. Cryst., 2006, 452, 123; CrossRef (i) M.-G. Liu and C.-L. Ni, Acta Crystallogr., 2006, E62, m3039; CrossRef (j) G. Bruno, M. Almeida, D. Simão, M. L. Mercuri, L. Pilia, A. Serpe, and P. Deplano, Dalton Trans., 2009, 495; CrossRef (k) H. Zuo, J. Tian, X. Chen, Q. Huang, J.-R. Zhou, X.-P. Liu, C.-L. Ni, and X.-L. Hu, J. Chem. Crystallogr., 2009, 39, 698; CrossRef (l) Z.-M. Wang, F.-Q. Wang, and Z.-Y. Liu, Acta Crystallogr., 2011, E67, m1143; CrossRef (m) J.-F. Liu, J. Tian, W.-Q. Chen, L.-B. Liang, L.-L. Yu, L.-M. Yang, J.-R. Zhou, and C.-L. Ni, J. Chem. Crystallogr., 2012, 42,450; CrossRef (n) Z.-H. Zeng and S.-B. Yang, Acta Crystallogr., 2012, E68, m239. CrossRef
3. (a) K. Awaga, T. Okuno, Y. Maruyama, A. Kobayashi, H. Kobayashi, S. Schenk, and A. E. Underhill, Inorg. Chem., 1994, 33, 5598; CrossRef (b) N. Robertson, K. Awaga, S. Parsons, A. Kobayashi, and A. E. Underhill, Adv. Mater. Opt. Electron., 1998, 8, 93; (c) L. Pilia, C. Faulmann, I. Malfant, V. Collière, M. L. Mercuri, P. Deplano, and P. Cassoux, Acta Crystallogr., 2002, C58, m240; CrossRef (d) D. Simão, H. Alves, I. C. Santos, V. Gama, and M. Almeida, Inorg. Chem. Commun., 2003, 6, 565. CrossRef
4. S. Horiuchi, Y. Tokunaga, G. Giovannetti, S. Picozzi, H. Itoh, R. Shimano, R. Kumai, and Y. Tokura, Nature, 2010, 463, 789. CrossRef
5. In a 20 mL flask trifluoroacetic acid (TFA) was added dropwise to a solution of (Et4N)2[Ni(tdas)2] (100 mg, 0.12 mmol) in acetonitrile. The solution turned from deep yellow to red. The addition of TFA was continued until the reaction mixture turned reddish purple. Deep purple precipitation appeared and was collected by filtration. Yield: 92% (40 mg). Anal. Calcd for C4H2N4NiS6: C, 13.45; H, 0.56; N, 15.69. Found: C, 13.68; H, 0.51; N, 15.62. IR (KBr) ν/cm–1 3200–2800mbr, 1435s, 1361s, 1314m, 1198s, 1027w, 1004w, 790s, 767w, 729m, 694m, 675m, 484m.
6. In a 20 mL flask TFA was added to a solution of (n-Bu4N)2[Ni(tdas)2] (50 mg, 6.1 × 10–5 mol) in MeCN until the solution turned reddish purple. The red precipitate was collected by filtration. Yield: 83% (30 mg). Anal. Calcd for C20H37N5NiS6: C, 40.13; H, 6.23; N, 11.70. Found: C, 40.22; H, 6.07; N, 11.64. mp > 100 °C (dec.). IR (KBr) ν/cm–1 2955m, 2928m, 2869m, 2717w, 2634w, 2575w, 2522w, 2354wbr, 1850–1650wbr, 1486w, 1461m, 1395w, 1378s, 1325s, 1231sbr, 1175w, 1149w, 1106w, 1036m, 880w, 798s, 771s, 738w, 700w, 502w, 484w.
7. Crystal data for Ni(Htdas)2·2H2O: C4H6N4NiO2S6, Mw = 393.20, monoclinic, P21/c (#14), a = 4.3660(8), b = 10.7663(17), c = 13.527(3) Å, β = 94.051(4)°, V = 634.25(19) Å3, T = 200 K, Z = 2, ρ(calcd) = 2.059 g cm–3, μ(MoKα) = 2.51 mm–1, reflections collected = 6130, unique reflections = 1456, Rint = 0.080, param. refined = 95, R1 = 0.054 (I > 2σ(I)), wR2 = 0.149 (all data), GOF = 1.11. CCDC-901459.
8. According to the reference 2m, the mean bond lengths of S–C and N–C are 1.737 and 1.319 Å, respectively.
9. Crystal data for (n-Bu4N)2[Ni(tdas)2]: C20H37N5NiS6, Mw = 598.62, Tetragonal, P41 (#76), a = 14.0423(11) Å, c = 29.073(4) Å, V = 5732.8(10) Å3, T = 150 K, Z = 8, ρ(calcd) = 1.385 g cm–3, μ(MoKα) = 1.13 mm–1, reflections collected = 56984, unique reflections = 13086, Rint = 0.109, param. refined = 604, R1 = 0.088 (I > 2σ(I)), wR2 = 0.223 (all data), GOF = 1.14. CCDC-901460.
10. Spectrophotometric titrations were carried out with perchloric acid (1 M) in an acetonitrile–water solution (v/v = 1:1). The pH measurements were carried out at room temperature using a HORIBA F-12 pH meter.