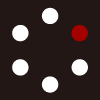
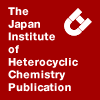
HETEROCYCLES
An International Journal for Reviews and Communications in Heterocyclic ChemistryWeb Edition ISSN: 1881-0942
Published online by The Japan Institute of Heterocyclic Chemistry
e-Journal
Full Text HTML
Received, 18th September, 2012, Accepted, 22nd October, 2012, Published online, 5th November, 2012.
DOI: 10.3987/COM-12-S(N)124
■ DEHYDROGENATIVE N-HETEROCYCLIZATION OF 2-(2-AmINOARYL)ETHYL ALCOHOLS TO INDOLE DERIVATIVES CATALYZED BY (μ-OXO)TETRARUTHENIUM CLUSTER/1,2-BIS(DIPHENYLPHOSPHINO)BENZENE
Teruyuki Kondo,* Takashi Kanda, Daisuke Takagi, Kenji Wada, Yu Kimura, and Akio Toshimitsu
Advanced Biomedical Engineering Research Unit, Kyoto University, Katsura, Nishikyo-ku, Kyoto 615-8510, Japan
Abstract
A novel catalyst system of (μ-oxo)tetraruthenium cluster (2) combined with 1,2-bis(diphenylphosphino)benzene (dppbz) realized a simple, selective, and practical synthesis of indole and its derivatives from 2-(2-aminoaryl)ethyl alcohols via dehydrogenative N-heterocyclization reaction. Spontaneous formation of a stoichiometric amount of hydrogen (H2) was observed, and the present reaction proceeded smoothly under an argon atmosphere without oxidants and/or hydrogen acceptors.Indole is one of the most important heterocycles as a result of its abundance in natural products, biologically active molecules, and pharmaceuticals.1,2 For example, an indole skeleton is included in the essential amino acid tryptophan, the neurotransmitter serotonin, the anti-inflammatory drug indomethacin,3 and antitumoral nortopsentins.4 Furthermore, the neurokinin-, chemokine-, serotonin-, and melanocortin-receptor families have been found to be activated by ligands with an indole skeleton.5 Accordingly, considerable attention has been focused on developing the highly efficient synthesis of indole and its derivatives. Among various methods for the construction of an indole skeleton,6 oxidative or dehydrogenative N-heterocyclization of 2-(2-aminoaryl)ethyl alcohols is one of the most promising protocols,7-11 since the starting alcohols are easily prepared by the condensation of 2-nitrotoluene derivatives with formaldehyde in the presence of bases, followed by the reduction of a nitro functionality to an amino one.8
We have been interested in ruthenium chemistry, and have successfully synthesized many novel low-valent ruthenium complexes,12 which can be used as highly efficient catalysts for characteristic organic synthesis.13 In addition, we have succeeded in the synthesis of a novel and isolable (μ-oxo)tetraruthenium cluster (2)14 by an unusual reaction of a zero-valent ruthenium complex, Ru(η6-cot)(η2-dmfm)2 (1)15 [cot = 1,3,5-cyclooctatriene, dmfm = dimethyl fumarate], with H2O (eq. 1).
More recently, we have reported that this novel (μ-oxo)tetraruthenium cluster (2) efficiently catalyzed a simple, practical, and selective oxidation of primary and secondary alcohols to the corresponding aldehydes and ketones, respectively, under air.16 As part of continuing exploratory work, we have conducted studies aimed at expanding the applicability of 2 to catalytic organic synthesis. The results of this effort show that a new catalyst system of 2 combined with 1,2-bis(diphenylphosphino)benzene (dppbz) is highly effective for dehydrogenative N-heterocyclization of 2-(2-aminoaryl)ethyl alcohols to the corresponding indole derivatives.
2-(2-Aminophenyl)ethyl alcohol (3a) was treated with a catalytic amount of 2 and 1,2-bis(diphenylphosphino)benzene (dppbz) in toluene at 130 °C (bath temp.) for 20 h under 1 atm of O2 (balloon) to give indole (4a) in 77% yield (eq. 2).
As shown in Table 1, dppbz has a crucial effect on the catalytic activity of 2. In the absence of dppbz, indole was obtained in only 14% yield. Among diphosphines, 1,2-bis(diphenylphosphino)ethane (dppe) was applicable (4a, 71%), and the moderate catalytic activity of 2 combined with other diphosphines, such as 1,2-bis(diphenylphosphino)- methane (dppm), and 1,2-bis(diphenylphosphino)- propane (dppp), was observed. The use of two equivalents of PPh3 instead of dppbz also increased the catalytic activity of 2; however, the catalytic activity of 2 combined with 1,2-bis(diphenylphosphino)butane (dppb) or tricyclohexylphosphine (PCy3) was low. No positive effect of diamine ligands, such as N,N,N’,N’-tetraethylethylenediamine (TEEDA) and 2,2’-bipyridine (2,2’-bipy), was observed.
As for solvents,17 toluene and mesitylene were suitable for the present reaction, and the temperature over 130 °C is required for complete conversion of 3a to 4a.
The results obtained from the reaction of several 2-(2-aminoaryl)ethyl alcohols 3 under optimum reaction conditions are summarized in Table 2. 2-(2-Aminoaryl)ethyl alcohols 3b-f bearing chloro, bromo, methyl and methoxy substituents on a phenyl ring were smoothly converted into the corresponding indole derivatives 4b-f in high yields.18 Especially, 4-bromoindole, which is a key intermediate in the synthesis of ergot alkaloids,19 can be readily synthesized.
In all reactions, no indoline derivatives were formed. Since dehydrogenation of indoline (5a) to indole (4a) did not proceed at all under the present catalytic reaction conditions, the present reaction does not involve the formation of indoline as an intermediate.8,20 In addition, the present reaction proceeds under an air or an argon atmosphere, and neither oxidant nor hydrogen acceptor was required (Scheme 1).
When the reaction was carried out under an argon atmosphere, the spontaneous formation of a stoichiometric amount of H2 (1.0 mmol, 22.4 mL) was detected in a gas phase by the careful GC analysis (MS-5A).
Considering the results obtained above, the most plausible mechanism is illustrated in Scheme 2. We believe that the oxidation of an alcohol functionality in 3 to an aldehyde one first proceeded, and subsequent intramolecular dehydration between an aldehyde group with an amino group gave a cyclic imine intermediate, 3H-indole. Direct isomerization of 3H-indole proceeded to give the desired indole,21 not via an indoline intermediate (vide supra).
In order to investigate the role of dppbz, the stoichiometric reaction of 2 with dppbz was monitored by 31P NMR, which clearly showed that -PPh2 (dppbz, -15.19 ppm, s) was completely converted into -P(=O)Ph2 (dppbzO2, 28.03 ppm, s) during the reaction.22 This result clearly showed that the transfer of oxo ligands from 2 to dppbz occurred first to give a coordinatively unsaturated ruthenium species.23 There are no further evidences about the real catalyst species, but an excess amount of dppbz (10 equivalents) is needed for the success of the present reaction, which strongly suggests that the coordination of an excess dppbz to a coordinatively unsaturated ruthenium species may give a real catalyst species (Scheme 3).24
In conclusion, we have developed a novel ruthenium catalyst system of 2 combined with dppbz, which realized a simple, selective, and practical synthesis of indole and its derivatives 4 from 2-(2-aminoaryl)ethyl alcohols 3 in toluene at 130 °C for 4-20 h under neutral conditions. A stoichiometric amount of hydrogen (H<sub>2</sub>) was formed spontaneously during the reaction, and neither oxidant nor hydrogen acceptor was required. Investigation of an active ruthenium species (cluster or mononuclear) as well as an application of the present catalyst system to other oxidative reactions are now in progress.
ACKNOWLEDGEMENTS
This work was supported in part by a Grant-in-Aid for Challenging Exploratory Research (No. 24655138) from the Japan Society for the Promotion of Science (JSPS). T.K. acknowledges financial support from the Asahi Glass Foundation and the Yazaki Memorial Foundation for Science and Technology. This research was conducted in part at the Advanced Research Institute (Research Project of Engineering for Sustainable Environment), Katsura-Int’tech Center, Graduate School of Engineering, Kyoto University.
References
1. J. Barluenga and C. Valdés, 'Modern Heterocyclic Chemistry,' Vol. 1, Chap. 5 ed. by J. Alvarez-Builla, J. J. Vaquero, and J. Barluenga, Wiley-VCH, Weinheim, 2011, pp. 377-531. CrossRef
2. S. Bräse, C. Gil, and K. Knepper, Bioorg. Med. Chem., 2002, 10, 2415; CrossRef C. Gil and S. Bräse, J. Comb. Chem., 2009, 11, 175. CrossRef
3. F. D. Hart and P. L. Boardmin, Br. Med. J., 1963, 2, 965. CrossRef
4. X.-H. Gu, X.-Z. Wan, and B. Jiang, Bioorg. Med. Chem. Lett., 1999, 9, 569. CrossRef
5. R. W. DeSimone, K. S. Currie, S. A. Mitchell, J. W. Darrow, and D. A. Pippin, Comb. Chem. High Throughput Screening, 2004, 7, 473. CrossRef
6. L. S. Hegedus, Angew. Chem., Int. Ed. Engl., 1988, 27, 1113; CrossRef U. Pindur and R. Adam, J. Heterocycl. Chem., 1988, 25, 1; CrossRef T. L. Gilchrist, J. Chem. Soc., Perkin Trans. 1, 1999, 2849; CrossRef G. W. Gribble, J. Chem. Soc., Perkin Trans. 1, 2000, 1045; CrossRef G. R. Humphrey and J. T. Kuethe, Chem. Rev., 2006, 106, 2875; CrossRef J. Barluenga, F. Rodríguez, and F. T. Fañanás, Chem. Asian J., 2009, 4, 1036. CrossRef
7. J. Bakke, H. Heikman, and E. B. Hellgren, Acta Chem. Scand., Ser. B, 1974, 28, 393; W. Hammerschmidt, A. Baiker, A. Wokaun, and W. Fluhr, Appl. Catal., 1986, 20, 305. CrossRef
8. For Ru catalysts, see: Y. Tsuji, K.-T. Huh, Y. Yokoyama, and Y. Watanabe, J. Chem. Soc., Chem. Commun., 1986, 1575; CrossRef Y. Tsuji, S. Kotachi, K.-T. Huh, and Y. Watanabe, J. Org. Chem., 1990, 55, 580. CrossRef
9. Other examples of Ru catalysts, see: R. R. Webb II, M. C. Venuti, and C. Eigenbrot, J. Org. Chem., 1991, 56, 4706; CrossRef T. Izumi and T. Yokota, J. Heterocycl. Chem., 1992, 29, 1085; CrossRef S. C. Ghosh and S. H. Hong, Eur. J. Org. Chem., 2010, 4266. CrossRef
10. For Ir catalysts, see: K. Fujita, K. Yamamoto, and R. Yamaguchi, Org. Lett., 2002, 4, 2691; CrossRef S. Whitney, R. Grigg, A. Derrick, and A. Keep, Org. Lett., 2007, 9, 3299; CrossRef C.-F. Fu, Y.-H. Chang, Y.-H. Liu, S.-M. Peng, C. J. Elsevier, J.-T. Chen, and S.-T. Liu, Dalton Trans., 2009, 6991; CrossRef A. Zanardi, J. A. Mata, and E. Peris, Chem. Eur. J., 2010, 16, 13109. CrossRef
11. For heterogeneous catalysts, see: R. Bernini, S. Cacchi, G. Fabrizi, S. Niembro, A. Prastaro, A. Shafir, and A. Vallribera, ChemSusChem, 2009, 2, 1036; CrossRef X. Guo, Z. Peng, S. Jiang, and J. Shen, Synth. Commun., 2011, 41, 2044; CrossRef S. Shimura, H. Miura, K. Wada, S. Hosokawa, S. Yamazoe, and M. Inoue, Catal. Sci. Technol., 2011, 1, 1340. CrossRef
12. T. Mitsudo, Y. Ura, and T. Kondo, Chem. Rec., 2006, 6, 107; CrossRef T. Mitsudo, Y. Ura, and T. Kondo, Proc. Jpn. Acad., Ser. B, 2007, 83, 65, and references therein. CrossRef
13. T. Kondo, Synlett, 2008, 629; CrossRef T. Kondo, Bull. Chem. Soc. Jpn., 2011, 84, 441, and references therein. CrossRef
14. T. Kondo, F. Tsunawaki, T. Suzuki, Y. Ura, K. Wada, S. Yamaguchi, H. Masuda, K. Yoza, M. Shiro, and T. Mitsudo, J. Organomet. Chem., 2007, 692, 530. CrossRef
15. T. Mitsudo, T. Suzuki, S.-W. Zhang, D. Imai, K. Fujita, T. Manabe, M. Shiotsuki, Y. Watanabe, K. Wada, and T. Kondo, J. Am. Chem. Soc., 1999, 121, 1839. CrossRef
16. T. Kondo, Y. Kimura, T. Kanda, D. Takagi, K. Wada, and A. Toshimitsu, Green Sus. Chem., 2011, 1, 149.
17. Anhydrous toluene, THF, and CH2Cl2 were obtained by filtration through a drying column on a GlassContour system, and used after bubbling with argon. A. B. Pangborn, M. A. Giardello, R. H. Grubbs, R. K. Rosen, and F. J. Timmers, Organometallics, 1996, 15, 1518. CrossRef
18. A mixture of 2-(2-aminoaryl)ethyl alcohol (3, 1.0 mmol), (μ-oxo)Ru4 cluster (2) (0.0050 mmol), dppbz (0.050 mmol), and toluene (2.0 mL) was placed in a 20-mL Pyrex flask equipped with a magnetic stirring bar and a reflux condenser under a flow of argon. The reaction was carried out at 130 °C for 4-20 h with stirring under an oxygen or argon atmosphere (1 atm, balloon). After being allowed to cool, the reaction mixture was analyzed by GLC and GC-MS. The products were isolated by Kugelrohr distillation, if necessary, followed by silica gel column chromatography (40-50 μm (spherical, neutral), eluent: hexane / EtOAc = 8 / 1). 1H NMR spectra were recorded at 400 MHz, and 13C NMR spectra were recorded at 100 MHz in CDCl3. The chemical shift values are expressed relative to Me4Si as an internal standard.
19. P. J. Harrington and L. S. Hegedus, J. Org. Chem., 1984, 49, 2657. CrossRef
20. Catalytic conversion of indoline to indole, see: K. Yamaguchi and N. Mizuno, Chem. Eur. J., 2003, 9, 4353; CrossRef T. Hara, K. Mori, T. Mizugaki, K. Ebitani, and K. Kaneda, Tetrahedron Lett., 2003, 44, 6207; CrossRef Y. Maeda, T. Nishimura, and S. Uemura, Bull. Chem. Soc. Jpn., 2003, 76, 2399; CrossRef K. Kamata, J. Kasai, K. Yamaguchi, and N. Mizuno, Org. Lett., 2004, 6, 3577; CrossRef T. Chandra, S. Zou, and K. L. Brown, Tetrahedron Lett., 2004, 45, 7783; CrossRef T. Noguchi, N. Tanaka, T. Nishimura, R. Goto, M. Hayakawa, A. Sugidachi, T. Ogawa, F. Asai, Y. Matsui, and K. Fujimoto, Chem. Pharm. Bull., 2006, 54, 163; CrossRef H. Choi and M. P. Doyle, Chem. Commun., 2007, 745; CrossRef S.-I. Murahashi, Y. Okano, H. Sato, T. Nakae, and N. Komiya, Synlett, 2007, 1675; CrossRef F. Li, J. Chen, Q. Zhang, and Y. Wang, Green Chem., 2008, 10, 553; CrossRef M.-H. So, Y. Liu, C.-M. Ho, and C.-M. Che, Chem. Asian J., 2009, 4, 1551; CrossRef K. Yamaguchi, J. W. Kim, J. He, and N. Mizuno, J. Catal., 2009, 268, 343; CrossRef L. Aschwanden, T. Mallat, F. Krumeich, and A. Baiker, J. Mol. Catal. A; Chemical, 2009, 309, 57; CrossRef L. Aschwanden, T. Mallat, M. Maciejewski, F. Krumeich, and A. Baiker, ChemCatChem, 2010, 2, 666; CrossRef P. Preedasuriyachai, W. Chavasiri, H. Sakurai, Synlett, 2011, 1121. CrossRef
21. I. G. Gut and J. Wirz, Angew. Chem. Int. Ed. Engl., 1994, 33, 1153. CrossRef
22. 31P NMR spectra were recorded at 162 MHz in (CD2Cl)2 with P(OMe)3 as an internal standard.
23. F. J. Arnáiz, R. Aguado, M. R. Pedrosa, J. Mahía, and M. A. Maestro, Polyhedron, 2002, 21, 1635; CrossRef A. Xu, H. Xiong, and G. Yin, Chem. Eur. J., 2009, 15, 11478.. CrossRef
24. For diphosphine-coordinated ruthenium catalysts, see: C. Bergounhou, P. Fompeyrine, G. Commenges, and J. J. Bonnet, J. Mol. Catal., 1988, 48, 285; CrossRef U. Matteoli, V. Beghetto, and A. Scrivanti, J. Mol. Catal. A: Chemical, 1996, 109, 45. CrossRef