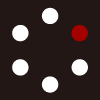
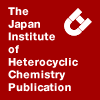
HETEROCYCLES
An International Journal for Reviews and Communications in Heterocyclic ChemistryWeb Edition ISSN: 1881-0942
Published online by The Japan Institute of Heterocyclic Chemistry
e-Journal
Full Text HTML
Received, 6th July, 2012, Accepted, 10th August, 2012, Published online, 24th August, 2012.
DOI: 10.3987/COM-12-S(N)91
■ TOTAL SYNTHESIS OF (+)-HETEROPLEXISOLIDE E
Noriki Kutsumura,* Akito Kiriseko, and Takao Saito*
Faculty of Science, Department of Chemistry, Tokyo University of Science, Kagurazaka 1-3, Shinjuku-ku, Tokyo 162-8601, Japan
Abstract
Total synthesis of (+)-heteroplexisolide E has been demonstrated. The synthetic approach exploits the one-pot regioselective Negishi coupling (methylation) and transformation of a β-methallyl alcohol moiety to a branched prenyl group via palladium-catalyzed hydrogenolysis. For the introduction of the 4-oxo-pentylidene side chain, two independent methods were devised: olefin cross metathesis (CM) (route A) and aldol condensation (route B).INTRODUCTION
Heteroplexisolide E (1) is a novel diterpene lactone. It is isolated from an ethanolic extract of Heteroplexis micocephala (Compositae family) that grows in the limestone terrains of Longzhou and Yangshuo in the Guangxi Province of China.1 This γ-lactonic natural product possesses a 4-oxo-pentylidene side chain and a branched prenyl group. No significant biological activity has been reported so far for this compound. Therefore, we initiated a synthetic study of (+)-1 to acquire sufficient quantities for a biological activity test. In this study, we disclose the first total synthesis of (+)-1 from the known starting material (+)-2 with an overall yield of 62%.2
RESULTS AND DISCUSSION
As shown in Scheme 1, our synthetic strategy involving known γ-lactone (+)-2 as a key starting material2,3 can serve in the diversity-oriented synthesis of various optically active γ-lactonic derivatives that can be studied further in terms of their chemical biology.4 In this context, Nishiyama and co-workers reported the total syntheses of (−)-muricatacin and (−)-(R,R)-sapinofuranone B by using compound (−)-2.3b,3c From our perspective, a straightforward strategy for the synthesis of (+)-1 would entail the development of methods for both the introduction of the 4-oxo-pentylidene side chain and the transformation of the allyl alcohol moiety to the branched prenyl group. For the introduction of the side chain, we envisaged two possible methods: (1) route A: olefin cross metathesis (CM) between the α-methylene-γ-lactone moiety and the corresponding terminal olefin and (2) route B: aldol condensation of the γ-lactone moiety with the corresponding aldehyde. On the other hand, the transformation to the prenyl group would be achieved through several steps, including a one-pot regioselective Negishi coupling.
The total synthesis of (+)-1 was achieved using a known chiral building block (+)-2, which was prepared in three steps from 1,4-pentadiene-3-ol (Scheme 2).2,3 The allyl alcohol derivative (+)-2 was converted into (+)-4 in a two-step sequential, one-pot bromination (i. bromine addition, ii. DBU-promoted elimination),5 or into (+)-5 in a three-step sequential, one-pot methylation (i. bromine addition, ii. DBU-promoted elimination, and iii. PEPPSI (Pyridine-enhanced precatalyst, preparation, stabilization, and initiation)TM-IPr (diisopropylphenylimidazolium derivative)6-catalyzed Negishi coupling).
Our first approach to introducing the alkyl side chain was through chemoselective olefin CM of α-methylene-γ-butyrolactone (+)-67 derived from (+)-4 using a terminal alkene 7 or 8 in the presence of 2,6-dichlorobenzoquinone and Grubbs 2nd or Hoveyda‒Grubbs 2nd generation catalyst (Scheme 3).8
This challenging reaction was based on the expectation that the exo-methylene moiety would be much more reactive than the anomalously low reactive vinyl bromide toward CM in general.9 Unfortunately, however, the expected products 9 or 10 were detected only in trace amounts under all reaction conditions attempted.10 This is probably because of the rapid decomposition of the ruthenium monohalomethylidene intermediate, as explained by Johnson and co-workers.11
An alternative pathway attempted is illustrated in Scheme 4. After the deprotection of the p-methoxybenzyl ether (PMB) group of (+)-5, the hydroxy lactone (+)-11 was derivatized to the desired prenyl-substituted lactone 13 in two ways: (1) a Barton‒McCombie-type transformation12 via the thiocarbonate (+)-12 (68%, 13:14 = 1:1) and (2) the Appel reaction13 and the sequential palladium-catalyzed hydrogenolysis14 of (−)-15 (72%, 13:14 = 11:1). After the methylenation of the lactones 13 and 14, chemoselective CM based on differentiation of the olefins between the resulting mixtures 16/17 and 8 was attempted. However, this approach was also unsuccessful, giving an unidentified complex mixture (Scheme 4).
A different approach (route B) to carbon-chain elongation was a much more secure and conservative pathway. An aldol reaction between (+)-5 and aldehyde 18 in the presence of lithium diisopropylamide (LDA) followed by dehydration gave only E-alkene (+)-19 in good yield (Scheme 5).15 Consecutive removal of the PMB group with 2,3-dichloro-4,5-dicyano-1,4-benzoquinone (DDQ) and the acetal group with pyridinium p-toluenesulfonate (PPTS) from (+)-19 in a one-pot process afforded the desired (+)-20 in excellent yield.
To efficiently transform the β-methallyl alcohol moiety of (+)-20 into the prenyl group at the final stage, we examined various allylic compounds,2 such as the acetate, ethyl carbonate, mesylate, and bromide, for the palladium-catalyzed hydrogenolysis.14 After many attempts, we finally found the optimal method. The Appel reaction of the sterically hindered secondary alcohol (+)-20 gave a mixture of bromide (+)-21 and the SN2′ isomeric product 21′ (Scheme 6),16 both of which were reasonably assumed to be a good precursor of (+)-1. Therefore, the mixture was exposed to the palladium-catalyzed hydrogenolysis to furnish an 8:1 mixture of (+)-1 and undesired product (+)-22 in excellent yield. Fortunately, the minor isomeric product (+)-22 was completely converted into (+)-1 by the reaction of palladium trifluoroacetate in toluene at 100 °C, even in the presence of the major product (+)-1.
In conclusion, the first total synthesis of (+)-1 from the known chiral building block (+)-2 was achieved with an overall yield of 62%. Synthetic highlights include the one-pot regioselective Negishi coupling (methylation) and the transformation of a β-methallyl alcohol moiety to a branched prenyl group by the palladium-catalyzed hydrogenolysis. The 4-oxo-pentylidene side chain was constructed through the aldol condensation pathway.
EXPERIMENTAL
General. Infrared spectra were recorded on a Horiba FT-710 spectrophotometer. 1H and 13C NMR spectroscopic data were obtained with a Bruker AV 600, a JEOL JNM-EX 500, or a Bruker BioSpin DPX 300 instrument. Chemical shifts (δ) are quoted in ppm using tetramethylsilane (TMS, δ = 0 ppm) for 1H NMR spectroscopy, and CDCl3 (δ = 77.0 ppm) for 13C NMR spectroscopy, as references. Mass spectra were measured on a Bruker Daltonics microTOF or a Hitachi double-focusing M-80B spectrometer. Column chromatography was conducted on silica gel (Kanto Chemical). All reactions were performed under an argon atmosphere.
Synthesis of (+)-5 from (+)-2 in a one-pot manner. A mixture of (+)-2 (1.09 g, 4.17 mmol) and pyridinium bromide perbromide (1.47 g, 4.58 mmol) in MeCN (41.7 mL) was stirred at room temperature for 15 h. DBU (1.96 mL, 12.9 mmol) was added to the reaction mixture at 0 °C and the system was then stirred at 60 °C for 2.5 h. After confirming consumption of (+)-3 by a thin layer chromatography (TLC), THF (10.5 mL), PEPPSITM-IPr (283 mg, 0.427 mmol), and dimethylzinc (24.0 mL, 25.0 mmol, 1.0 M sol. of hexane) were added to the reaction mixture at 0 °C without evaporation, and the system was then stirred at 60 °C for 1 h. The reaction was quenched with H2O at 0 °C and the reaction mixture was extracted with EtOAc. The combined extracts were washed with brine, dried over Na2SO4, concentrated in vacuo, and purified by silica gel column chromatography (hexane/EtOAc = 3/2) to afford (+)-5 (1.00 g, 87% in three steps). Compound (+)-5: [α]D +53.4 (c 1.00, CHCl3); IR (neat) 2947, 1774, 1612 cm-1; 1H NMR (500 MHz, CDCl3, δ) 1.77 (m, 3H), 1.93 (m, 1H), 2.14 (m, 1H), 2.44 (m, 1H), 2.55 (m, 1H), 3.73 (d, J = 5.7 Hz, 1H), 3.80 (s, 3H), 4.25 (d, J = 11.7 Hz, 1H), 4.54 (d, J = 11.7 Hz, 1H), 4.56 (ddd, J = 7.0, 7.0, 5.7 Hz, 1H), 5.03 (m, 1H), 5.12 (m, 1H), 6.87 (d, J = 8.7 Hz, 2H), 7.25 (d, J = 8.7 Hz, 2H); 13C NMR (126 MHz, CDCl3, δ) 18.0, 24.5, 28.3, 55.2, 69.9, 81.2, 84.2, 113.8, 113.8, 116.5, 129.4, 129.4, 129.8, 141.0, 159.2, 177.2; HRMS-ESI (m/z) [M+Na]+ Calcd for C16H20O4Na: 299.1254, Found: 299.1254.
Synthesis of (+)-6 from (+)-4. To a mixture of (+)-4 (583 mg, 1.70 mmol), Eschenmoser’s salt (1.58 g, 8.52 mmol), and DIPEA (1.50 mL, 8.61 mmol) in CH2Cl2 (17.0 mL) was added TMSOTf (0.70 mL, 3.87 mmol) slowly at −5 °C and then stirred for 5 min. The reaction was quenched with a saturated aqueous NaHCO3 solution at 0 °C and the reaction mixture was extracted with EtOAc. The combined extracts were dried over MgSO4 and concentrated in vacuo to afford a crude product as an oil. The crude product was dissolved in CH2Cl2 (17.0 mL) and then mCPBA (1.57 g, 6.83 mmol) was added slowly at room temperature. After being stirred at the same temperature for 1 h, the reaction was quenched with a saturated aqueous Na2SO3 solution and the reaction mixture was extracted with EtOAc. The combined extracts were washed with a saturated aqueous NaHCO3 solution, dried over MgSO4, concentrated in vacuo, and purified by silica gel column chromatography (hexane/EtOAc = 3/1−2/1−1/1) to afford (+)-6 (414 mg, 69% in two steps). Compound (+)-6: [α]D +56.1 (c 1.00, CHCl3); IR (neat) 2931, 2862, 1766, 1666, 1612, 1511 cm-1; 1H NMR (300 MHz, CDCl3, δ) 2.61 (dddd, J = 17.3, 8.4, 2.7, 2.6 Hz, 1H), 2.94 (dddd, J = 17.3, 5.4, 2.7, 2.6 Hz, 1H), 3.81 (s, 3H), 3.84 (d, J = 4.8 Hz, 1H), 4.32 (d, J = 11.6 Hz, 1H), 4.68 (d, J = 11.6 Hz, 1H), 4.75 (ddd, J = 8.4, 5.4, 4.8 Hz, 1H), 5.59 (dd, J = 2.6, 2.6 Hz, 1H), 5.87 (d, J = 1.9 Hz, 1H), 6.06 (d, J = 1.9 Hz, 1H), 6.22 (dd, J = 2.7, 2.7 Hz, 1H), 6.88 (d, J = 8.7 Hz, 2H), 7.25 (d, J = 8.7 Hz, 2H); 13C NMR (126 MHz, CDCl3, δ) 29.8, 55.3, 70.6, 76.0, 83.0, 113.9, 113.9, 121.6, 121.7, 128.1, 128.8, 129.8, 129.8, 133.9, 159.6, 169.9; HRMS-ESI (m/z) [M+Na]+ Calcd for C16H1779BrO4Na: 375.0202, Found: 375.0205; HRMS-ESI (m/z) [M+Na]+ Calcd for C16H1781BrO4Na: 377.0184, Found: 377.0179.
Synthesis of (+)-11 from (+)-5. A mixture of (+)-5 (38.2 mg, 0.14 mmol) and DDQ (37.7 mg, 0.17 mmol) in CH2Cl2 (1.4 mL) − phosphate buffer (pH 6.86 ± 0.02, 0.35 mL) was stirred at 0 °C for 15 min, then at room temperature for 1.5 h. The reaction was quenched with a saturated aqueous NaHCO3 solution and the reaction mixture was extracted with EtOAc. The combined extracts were dried over MgSO4, concentrated in vacuo, and purified by silica gel column chromatography (hexane/EtOAc = 1/1) to afford (+)-11 (20.5 mg, 95%). Compound (+)-11: [α]D +38.5 (c 1.00, CHCl3); IR (neat) 3448, 1759 cm-1; 1H NMR (500 MHz, CDCl3, δ) 1.80 (s, 3H), 2.09 (m, 1H), 2.21 (m, 1H), 2.50−2.65 (m, 2H), 2.75 (d, J = 4.3 Hz, 1H), 4.07 (dd, J = 5.7, 4.3 Hz, 1H), 4.59 (ddd, J = 7.3, 7.3, 5.7 Hz, 1H), 5.01 (m, 1H), 5.06 (m, 1H); 13C NMR (126 MHz, CDCl3, δ) 18.3, 24.0, 28.4, 77.6, 81.5, 114.3, 142.6, 177.1; HRMS-ESI (m/z) [M+Na]+ Calcd for C8H12O3Na: 179.0679, Found: 179.0674.
Barton‒McCombie-type transformation via the thiocarbonate (+)-12. A mixture of (+)-11 (13.1 mg, 0.0839 mmol), DMAP (30.8 mg, 0.252 mmol), and o-phenylchlorothionoformate (17 µL, 0.126 mmol) in MeCN (0.84 mL) was stirred at room temperature for 17 h. After the evaporation of the solvent, the residue was purified by silica gel column chromatography (CHCl3/MeCN = 50/1) to afford (+)-12 (22.6 mg, 92%). Compound (+)-12: [α]D +91.5 (c 0.14, CHCl3); IR (neat) 2924, 1774, 1188, 1103, 748 cm-1; 1H NMR (500 MHz, CDCl3, δ) 1.87 (s, 3H), 2.12 (m, 1H), 2.33 (m, 1H), 2.53−2.67 (m, 2H), 4.83 (ddd, J = 7.3, 7.3, 6.0 Hz, 1H), 5.20 (m, 1H), 5.22 (m, 1H), 5.72 (d, J = 6.0 Hz, 1H), 7.12 (d, J = 7.7 Hz, 2H), 7.30 (dd, J = 7.4, 7.4 Hz, 1H), 7.42 (dd, J = 7.7, 7.4 Hz, 2H); 13C NMR (126 MHz, CDCl3, δ) 19.2, 24.0, 28.0, 79.0, 87.2, 117.6, 121.8, 121.8, 126.7, 129.6, 129.6, 137.8, 153.3, 176.1, 194.0; HRMS-ESI (m/z) [M+Na]+ Calcd for C15H16O4SNa: 315.0662, Found: 315.0662.
A mixture of (+)-12 (22.6 mg, 0.0773 mmol), tributyltin hydride (13 µL, 0.101 mmol), and azobisisobutyronitrile (1.3 mg, 0.00792 mmol) in toluene (0.77 mL) was stirred at 100 °C for 5.5 h. After the evaporation of the solvent, the residue was purified by column chromatography (10% w/w anhydrous K2CO3-silica;17 hexane/EtOAc = 5/1) to afford a 1:1 mixture of 13 and 14 (8.0 mg, 68%). Compound 13: 1H NMR (300 MHz, CDCl3, δ) 1.74 (d, J = 1.1 Hz, 3H), 1.78 (d, J = 1.0 Hz, 3H), 1.93 (m, 1H), 2.36 (m, 1H), 2.55 (m, 2H), 5.22 (m, 1H), 5.22 (m, 1H); 13C NMR (126 MHz, CDCl3, δ) 18.3, 25.7, 29.1, 29.3, 77.6, 122.8, 139.8, 177.2. Compound 14: 1H NMR (300 MHz, CDCl3, δ) 1.78 (s, 3H), 1.91 (m, 1H), 2.26−2.38 (m, 2H), 2.45−2.57 (m, 3H), 4.66 (dddd, J = 14.4, 13.4, 7.7, 6.7 Hz, 1H), 4.80 (m, 1H), 4.88 (m, 1H); 13C NMR (126 MHz, CDCl3, δ) 22.8, 27.6, 28.6, 43.4, 79.1, 113.7, 140.4, 177.0.
Synthesis of (−)-15. A mixture of (+)-11 (55.2 mg, 0.353 mmol), tetrabromomethane (211 mg, 0.636 mmol), and triphenylphosphine (166 mg, 0.634 mmol) in CH2Cl2 (3.5 mL) was stirred at room temperature for 3.5 h. After the evaporation of the solvent, the residue was purified by silica gel column chromatography (hexane/EtOAc = 3/1) to afford (−)-15 (52.4 mg, 68%). Compound (−)-15: [α]D −17.2 (c 1.00, CHCl3); IR (neat) 1782, 640 cm-1; 1H NMR (500 MHz, CDCl3, δ) 1.90 (s, 3H), 2.17 (m, 1H), 2.52−2.67 (m, 3H), 4.48 (d, J = 8.9 Hz, 1H), 4.73 (m, 1H), 5.06 (m, 1H), 5.18 (m, 1H); 13C NMR (126 MHz, CDCl3, δ) 18.0, 27.0, 28.5, 59.3, 79.2, 117.5, 140.9, 176.1; HRMS-ESI (m/z) [M+Na]+ Calcd for C8H1179BrO2Na: 240.9835, Found: 240.9840; HRMS-ESI (m/z) [M+Na]+ Calcd for C8H1181BrO2Na:
242.9815, Found: 242.9816.
Palladium-catalyzed hydrogenolysis of (−)-15. To a solution of (−)-15 (31.9 mg, 0.146 mmol) and Pd(PPh3)4 (8.6 mg, 0.0074 mmol) in toluene (1.5 mL) was added tributyltin hydride (50 µL, 0.186 mmol) slowly at 100 °C. After being stirred at the same temperature for 20 min, the solvent was removed under reduced pressure, and the residue was purified by column chromatography (10% w/w anhydrous K2CO3-silica;17 hexane/EtOAc = 4/1−2/1) to afford the mixture of 13 and 14 (14.7 mg, 13:14 = 11:1, 72%).
Synthesis of 16 and 17. To a solution of an 11:1 mixture of 13 and 14 (14.8 mg, 0.106 mmol) in THF (1.1 mL) was added NaH (60% dispersion in mineral oil, 7.6 mg, 0.174 mmol) at 0 °C. After being stirred at 0 °C for 10 min, ethyl formate (17.5 µL, 0.217 mmol) in EtOH (1 drop) was added and the system was stirred at room temperature for 4 h. The browny crystals were collected by filtration with a suction filter and dissolved in THF (1.1 mL). Paraformaldehyde (14.3 mg, 0.477 mmol) was added to the stirred suspension and the system was stirred at 60 °C for 1 h. The reaction was quenched with a saturated aqueous K2CO3 solution and the reaction mixture was extracted with EtOAc. The combined extracts were dried over MgSO4, concentrated in vacuo, and purified by silica gel column chromatography (hexane/EtOAc = 2/1) to afford an 11:1 mixture of 16 and 17 (5.0 mg, 31%). Compound 16: 1H NMR (300 MHz, CDCl3, δ) 1.76 (s, 3H), 1.78 (s, 3H), 2.63 (dddd, J = 16.8, 6.0, 2.9, 2.6 Hz, 1H), 3.12 (dddd, J = 16.8, 7.2, 2.9, 2.6 Hz, 1H), 5.22 (m, 1H), 5.22 (m, 1H), 5.62 (dd, J = 2.6, 2.6 Hz, 1H), 6.22 (dd, J = 2.9, 2.9 Hz, 1H).
Aldol reaction: synthesis of (+)-19. To a solution containing diisopropylamine (0.57 mL, 4.06 mmol) in THF (8.0 mL) under argon at −78 °C was added n-butyllithium (1.6 mL, 4.06 mmol, 2.54 M sol. of hexane). Stirring at −78 °C was continued for 10 min, followed by the addition of (+)-5 (668 mg, 2.42 mmol) in THF (8.0 mL) via a cannula. The mixture was stirred at −78 °C for 45 min, followed by the addition of aldehyde 18 (702 mg, 4.87 mmol) in THF (8.0 mL) via a cannula. Stirring was continued at −78 °C for 1 h and then at 0 °C for 15 min, followed by the addition of a saturated aqueous NH4Cl solution. The mixture was extracted with EtOAc. The combined extracts were washed with brine, and dried over MgSO4. The residue obtained after the evaporation of the solvent was dissolved in 1,2-dichloroethane (24 mL), then pyridine (0.77 mL, 9.54 mmol), DMAP (31.3 mg, 0.256 mmol), and acetic anhydride (0.91 mL, 9.63 mmol) were added at 0 °C, and solution was stirred at room temperature for 14.5 h. DBU (1.8 mL, 11.9 mmol) was added, and the mixture was stirred at 70 °C for 6 h. The reaction was quenched with a saturated aqueous NH4Cl solution at 0 °C. The mixture was extracted with EtOAc and the combined extracts were washed with brine, dried over MgSO4, concentrated in vacuo, and purified by silica gel column chromatography (hexane/EtOAc = 3/2−1/1) to afford (+)-19 (837 mg, 86% from (+)-5) as the sole product. Compound (+)-19: [α]D +54.4 (c 1.00, CHCl3); IR (neat) 2939, 2877, 1759, 1682, 1612, 1511 cm-1; 1H NMR (300 MHz, CDCl3, δ) 1.32 (s, 3H), 1.77 (s, 3H), 1.80 (m, 2H), 2.24 (m, 2H), 2.53 (m, 1H), 2.76 (m, 1H), 3.72 (d, J = 6.2 Hz, 1H), 3.80 (s, 3H), 3.94 (m, 4H), 4.26 (d, J = 11.7 Hz, 1H), 4.54 (d, J = 11.7 Hz, 1H), 4.60 (ddd, J = 8.4, 6.2, 5.8 Hz, 1H), 5.03 (brs, 1H), 5.14 (dd, J = 1.5, 1.5 Hz, 1H), 6.69 (tdd, J = 7.5, 2.8, 2.8 Hz, 1H), 6.87 (d, J = 8.7 Hz, 2H), 7.24 (d, J = 8.7 Hz, 2H); 13C NMR (126 MHz, CDCl3, δ) 18.0, 23.8, 24.7, 27.6, 37.1, 55.2, 64.7, 64.7, 69.9, 77.8, 84.0, 109.2, 113.7, 113.7, 116.8, 125.9, 129.3, 129.3, 129.8, 139.6, 140.8, 159.1, 170.6; HRMS-ESI (m/z) [M+Na]+ Calcd for C23H30O6Na: 425.1935, Found: 425.1931.
Synthesis of (+)-20. A mixture of (+)-19 (449 mg, 1.11 mmol) and DDQ (320 mg, 1.41 mmol) in CH2Cl2 (8.0 mL) − H2O (2.0 mL) was stirred at room temperature for 6 h. To the reaction mixture were added H2O (3.0 mL), acetone (5.0 mL), and PPTS (84.0 mg, 0.334 mmol) and the system was stirred at 50 °C for 4 h. After the evaporation of the solvent, the reaction was quenched with a saturated aqueous NaHCO3 solution and the reaction mixture was extracted with EtOAc. The combined extracts were dried over MgSO4, concentrated in vacuo, and purified by silica gel column chromatography (hexane/EtOAc = 1/2−1/3) to afford (+)-20 (241 mg, 90%). Compound (+)-20: [α]D +32.2 (c 1.00, CHCl3); IR (neat) 3455, 3078, 1751, 1712, 1681 cm-1; 1H NMR (300 MHz, CDCl3, δ) 1.82 (brs, 3H), 2.16 (s, 3H), 2.33 (brm, 1H), 2.42 (dt, J = 7.1, 7.1 Hz, 2H), 2.65 (t, J = 7.1 Hz, 2H), 2.73 (m, 1H), 2.92 (m, 1H), 4.04 (dd, J = 6.0, 6.0 Hz, 1H), 4.61 (ddd, J = 8.3, 6.0, 6.0 Hz, 1H), 5.05 (brm, 1H), 5.08 (brs, 1H), 6.60 (tdd, J = 7.1, 2.9, 2.9 Hz, 1H); 13C NMR (126 MHz, CDCl3, δ) 18.3, 24.0, 27.7, 29.9, 41.3, 78.0, 78.4, 114.9, 127.0, 138.8, 142.3, 170.2, 206.7; HRMS-ESI (m/z) [M+Na]+ Calcd for C13H18O4Na: 261.1097, Found: 261.1097.
Synthesis of a mixture of (+)-1 and (+)-22 utilizing bromination and sequential hydrogenolysis. A mixture of (+)-20 (10.3 mg, 0.0429 mmol), tetrabromomethane (29.1 mg, 0.0877 mmol), and triphenylphosphine (23.0 mg, 0.0877 mmol) in CH2Cl2 (0.43 mL) was stirred at room temperature for 22 h. After evaporation, the residue was purified by silica gel column chromatography (hexane/EtOAc = 1/1) to afford a mixture of (+)-21 and the isomeric product 21'. Compound (+)-21: [α]D +10.4 (c 0.62, CHCl3); IR (neat) 2924, 1759, 1712, 1682, 640 cm-1; 1H NMR (300 MHz, CDCl3, δ) 1.91 (brs, 3H), 2.17 (s, 3H), 2.45 (m, 2H), 2.66 (t, J = 7.0 Hz, 2H), 2.87 (m, 1H), 3.19 (m, 1H), 4.46 (d, J = 8.8 Hz, 1H), 4.76 (ddd, J = 8.8, 5.5, 5.5 Hz, 1H), 5.07 (m, 1H), 5.17 (s, 1H), 6.64 (tdd, J = 7.0, 2.9, 2.9 Hz, 1H); 13C NMR (126 MHz, CDCl3, δ) 18.1, 24.1, 30.0, 30.8, 41.3, 60.1, 76.3, 117.7, 126.6, 139.6, 140.9, 169.9, 206.4; HRMS-ESI (m/z) [M+Na]+ Calcd for C13H1779BrO3Na: 323.0253, Found: 323.0256; HRMS-ESI (m/z) [M+Na]+ Calcd for C13H1781BrO3Na: 325.0234, Found: 325.0239.
To the mixture in toluene (0.43 mL) was added Pd(PPh3)4 (2.5 mg, 0.00216 mmol) and then heated to 100 °C. Tributyltin hydride (13 µL, 0.0483 mmol) was added and the system was stirred for 10 min. After being cooled to room temperature, the solvent was removed under reduced pressure, and the residue was purified by column chromatography (10% w/w anhydrous K2CO3-silica;17 hexane/EtOAc = 2/1−1/1) to afford the mixture of (+)-1 and (+)-22 (9.3 mg, (+)-1:(+)-22 = 8:1, 98% in two steps). Heteroplexisolide E (1): [α]D +74.0 (c 0.38, MeOH); IR (neat) 2978, 2922, 1755, 1720, 1684 cm-1; 1H NMR (600 MHz, acetone-d6, δ) 1.75 (s, 3H), 1.75 (s, 3H), 2.10 (s, 3H), 2.37 (dt, J = 7.5, 7.1 Hz, 2H), 2.55 (m, 1H), 2.70 (t, J = 7.1 Hz, 2H), 3.17 (m, 1H), 5.25 (m, 1H), 5.25 (m, 1H), 6.49 (tdd, J = 7.5, 2.9, 2.9 Hz, 1H); 1H NMR (500 MHz, CDCl3, δ) 1.73 (s, 3H), 1.76 (s, 3H), 2.15 (s, 3H), 2.40 (dt, J = 7.5, 7.2 Hz, 2H), 2.54 (m, 1H), 2.62 (t, J = 7.2 Hz, 2H), 3.10 (m, 1H), 5.21 (m, 1H), 5.21 (m, 1H), 6.56 (tdd, J = 7.5, 2.9, 2.9 Hz, 1H); 13C NMR (150 MHz, acetone-d6, δ) 18.2, 24.6, 25.7, 30.2, 32.8, 41.6, 74.7, 125.2, 128.8, 138.5, 139.2, 170.8, 206.8; 13C NMR (126 MHz, CDCl3, δ) 18.4, 24.1, 25.7, 30.0, 32.5, 41.5, 74.4, 123.7, 127.9, 137.9, 139.6, 170.8, 206.6; HRMS-ESI (m/z) [M+Na]+ Calcd for C13H18O3Na: 245.1148, Found: 245.1152. Compound (+)-22: [α]D +31.4 (c 0.26, CHCl3); IR (neat) 1751, 1712 cm-1; 1H NMR (300 MHz, CDCl3, δ) 1.78 (s, 3H), 2.16 (s, 3H), 2.28 (dd, J = 14.4, 7.1 Hz, 1H), 2.42 (m, 2H), 2.52 (dd, J = 14.4, 6.6 Hz, 1H), 2.57 (m, 1H), 2.64 (t, J = 7.1 Hz, 2H), 3.02 (m, 1H), 4.70 (m, 1H), 4.80 (brs, 1H), 4.89 (brs, 1H), 6.60 (tdd, J = 7.1, 2.9, 2.9 Hz, 1H); 13C NMR (126 MHz, CDCl3, δ) 22.8, 24.0, 30.0, 30.9, 41.4, 44.3, 75.9, 113.9, 127.5, 138.5, 140.3, 170.6, 206.6; HRMS-ESI (m/z) [M+Na]+ Calcd for C13H18O3Na: 245.1148, Found: 245.1146.
Palladium-catalyzed conversion into (+)-1. To a solution of a 8:1 mixture of (+)-1 and (+)-22 (3.5 mg, 0.0157 mmol) in toluene (0.16 mL) was added Pd(OCOCF3)2 (0.5 mg, 0.00150 mmol) at room temperature. After being stirred at 100 °C for 10 h, the solvent was removed under reduced pressure, and the residue was purified by column chromatography (hexane/EtOAc = 3/2) to afford (+)-1 (3.3 mg, 94%) as a single product.
ACKNOWLEDGEMENTS
This work was partly supported by the Sasakawa Scientific Research Grant from The Japan Science Society and by MEXT-Supported Program for the Strategic Research Foundation at Private Universities, 2012-2016.
References
1. X. Fan, J. Zi, C. Zhu, W. Xu, W. Cheng, S. Yang, Y. Guo, and J. Shi, J. Nat. Prod., 2009, 72, 1184. CrossRef
2. N. Kutsumura, A. Kiriseko, and T. Saito, Tetrahedron Lett., 2012, 53, 3274. CrossRef
3. (a) L. Zhu and D. R. Mootoo, Org. Lett., 2003, 5, 3475; CrossRef (b) N. Kutsumura, T. Yokoyama, T. Ohgiya, and S. Nishiyama, Tetrahedron Lett., 2006, 47, 4133; CrossRef (c) T. Yokoyama, N. Kutsumura, T. Ohgiya, and S. Nishiyama, Bull. Chem. Soc. Jpn., 2007, 80, 578. CrossRef
4. (a) E. Rodriguez, G. H. N. Towers, and J. C. Mitchell, Phytochemistry, 1976, 15, 1573; CrossRef (b) R. R. A. Kitson, A. Millemaggi, and R. J. K. Taylor, Angew. Chem. Int. Ed., 2009, 48, 9426; CrossRef (c) J.-P. Lepoittevin, V. Berl, and E. Giménez-Arnau, Chem. Rec., 2009, 9, 258. CrossRef
5. N. Kutsumura, K. Niwa, and T. Saito, Org. Lett., 2010, 12, 3316. CrossRef
6. (a) C. Valente, M. E. Belowich, N. Hadei, and M. G. Organ, Eur. J. Org. Chem., 2010, 4343; CrossRef (b) J. Nasielski, N. Hadei, G. Achonduh, E. A. B. Kantchev, C. J. O’Brien, A. Lough, and M. G. Organ, Chem. Eur. J., 2010, 16, 10844. CrossRef
7. (a) M. Inoue, T. Sato, and M. Hirama, J. Am. Chem. Soc., 2003, 125, 10772; CrossRef (b) J. Zhou, A. M. Schmidt, and H. Ritter, Macromolecules, 2010, 43, 939. CrossRef
8. (a) J. Moïse, S. Arseniyadis, and J. Cossy, Org. Lett., 2007, 9, 1695; CrossRef (b) R. Raju, L. J. Allen, T. Le, C. D. Taylor, and A. R. Howell, Org. Lett., 2007, 9, 1699. CrossRef
9. (a) W. Chao and S. M. Weinreb, Org. Lett., 2003, 5, 2505; CrossRef (b) V. Sashuk, C. Samojłowicz, A. Szadlowska, and K. Grela, Chem. Commun., 2008, 2468; CrossRef (c) M. Gatti, E. Drinkel, L. Wu, I. Pusterla, F. Gaggia, and R. Dorta, J. Am. Chem. Soc., 2010, 132, 15179. CrossRef
10. The best result in this examination was only 3.5% and (+)-6 was recovered (91%).
11. M. L. Macnaughtan, J. B. Gary, D. L. Gerlach, M. J. A. Johnson, and J. W. Kampf, Organometallics, 2009, 28, 2880. CrossRef
12. D. H. R. Barton and S. W. McCombie, J. Chem. Soc., Perkin Trans. 1, 1975, 1574. CrossRef
13. S. Khan, N. Kato, and M. Hirama, Synlett, 2000, 1494. CrossRef
14. J. Tsuji and T. Mandai, Synthesis, 1996, 1. CrossRef
15. (a) M. Tanaka, C. Mukaiyama, H. Mitsuhashi, M. Maruno, and T. Wakamatsu, J. Org. Chem., 1995, 60, 4339; CrossRef (b) Y. Choi, J.-H. Kang, N. E. Lewin, P. M. Blumberg, J. Lee, and V. E. Marquez, J. Med. Chem., 2003, 46, 2790; CrossRef (c) D. J. Edmonds, K. W. Muir, and D. J. Procter, J. Org. Chem., 2003, 68, 3190; CrossRef (d) X.-S. Peng and H. N. C. Wong, Chem. Asian. J., 2006, 1-2, 111; CrossRef (e) G. O. Berger and M. A. Tius, J. Org. Chem., 2007, 72, 6473. CrossRef
16. (a) F. Bohlmann and A. Steinmeyer, Tetrahedron Lett., 1986, 27, 5359; CrossRef (b) M. E. Bouillon and H. H. Meyer, Tetrahedron, 2007, 63, 2712. CrossRef
17. D. C. Harrowven, D. P. Curran, S. L. Kostiuk, I. L. Wallis-Guy, S. Whitinag, K. J. Stenning, B. Tang, E. Packard, and L. Nanson, Chem. Commun., 2010, 46, 6335. CrossRef