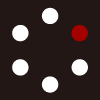
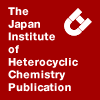
HETEROCYCLES
An International Journal for Reviews and Communications in Heterocyclic ChemistryWeb Edition ISSN: 1881-0942
Published online by The Japan Institute of Heterocyclic Chemistry
e-Journal
Full Text HTML
Received, 30th June, 2012, Accepted, 10th August, 2012, Published online, 24th August, 2012.
DOI: 10.3987/COM-12-S(N)80
■ AZULENOPENTATHIEPIN: PREPARATION AND CONVERSION INTO AZULENES WITH SULFUR GROUPS AT THE 1- AND 2-POSITIONS
Ohki Sato,* Atsushi Sakai, Masami Aoki, Takaaki Kuramochi, and Juzo Nakayama
Department of Chemistry, Faculty of Science, Saitama University, Urawa, Saitama 338-8570, Japan
Abstract
Azulenopentathiepin was prepared by the reaction of azulene with elemental sulfur in boiling pyridine. Bis(thiolate) generated from the pentathiepin reacted with electrophiles such as iodomethane, methyl chloroformate, N,N’-carbonyldiimidazole and N,N’-thiocarbonyldiimidazole to afford the corresponding azulene derivatives. Desulfuration of the pentathiepin and the successive reaction with DMAD or a Pd(0) reagent afforded a 1,4-dithiin compound or Pd complexes.INTRODUCTION
Azulenes are one of the most interesting classes in non-benzenoid aromatics and their chemical, physical and biological properties are of interest. Particularly, their electrochemical properties much attracted our attention and we had already reported azulene-based electron acceptors such as azulenequinones1a and tetracyanoazulenequinodimethanes.1b,c Our next interest is focused on azulene-based electron donors. For their construction, sulfur groups (S-groups) should be introduced into an azulene skeleton. However, synthetic methods for S-substituted azulene derivatives have not been much investigated,2 and no method for direct introduction of S-groups at the 2-position is known so far as we are aware. [1,2,3,4,5]Pentathiepins3 are stable seven-membered ring compounds with five sulfur atoms and a carbon-carbon double bond. Various pentathiepins fused to aromatics such as benzene,4a naphthalene,4b phenanthrene,4b thiophene,4c pyrrole4c and ferrocene4d etc. were prepared, and they could be converted to 1,2-bis(S-substituted) aromatics.
We report herein the preparation of a new class of pentathiepin, azuleno[1,2-f][1,2,3,4,5]pentathiepin (2) by the sulfuration of azulene (1) with elemental sulfur in boiling pyridine, and its conversion into azulene derivatives with S-groups at the 1- and 2-positions.
RESULTS AND DISCUSSION
A reaction of azulene (1) with elemental sulfur in 1,2,4-trichlorobenzene (1,2,4-TCB) under thermal conditions afforded only bis(1-azulyl)sulfides (3) with an insoluble black solid and recovery of 1 (Table 1, Entry 1). 3 were a mixture of biazulenes connecting with one, two, three and more sulfur atoms (n = 1,2d, e 2,2e 3 and more) at a 1-position of both azulene moieties. The black solid might be a complex mixture of azulene oligomers and polymers networking with sulfur atoms at the 1-, 2- and/or 3-positions. In contrast, the reaction in boiling pyridine yielded a novel azulene-based cyclic pentasulfide, azulenopentathiepin (2) together with 3 and a black solid. The yield of 2 was improved owing to prevent intermolecular reactions in the diluted concentration (Entry 2: 6.0 x 10-2 M; Entry 3: 1.0 x 10-2 M). It is well known the low reactivity at a 2-position of azulenes5 in electrophilic substitutions. Nevertheless, the surprising sulfration at the position would proceed on account of the generation of highly reactive species derived from S8-pyridine. Activation of elemental sulfur by using pyridine was reported in preparation of a trisulfane from a cycloheptatriene.6 2 was also obtained by the reaction of 3 (nave. = 4, determined by elemental analysis, from the reaction in Entry 3) with elemental sulfur in pyridine (Entry 4). Although the yield of 2 was still not enough, the supply by successive reactions under the conditions in Entries 3 and 4 was somewhat effective, so far (total yield from 1: 28 %).
Azulenopentathiepin (2) was a bluish green powder [mp 142-143 °C (dec.)]. The 1H NMR spectrum showed five protons at δ 7.42, 7.50, 7.77, 8.32 and 8.72 with an appropriate coupling constant (J = 9.8 Hz) as a seven-membered ring moiety of azulenes and a singlet proton at δ 7.60 as a five-membered one. The 13C NMR spectrum showed ten carbons (two carbons were overlapped at δ 139.3) arising from an azulene skeleton. The longest wave-maximum of UV-VIS spectrum appeared at 605 nm (log ε 2.72) as a characteristic peak of azulene derivatives. MS spectra gave molecular ion and fragment ion peaks [FAB: m/z 287 (MH+), 286 (M+); EI: m/z 286 (M+), 222 (M+-2S)]. Together with the results of elemental analysis, those spectral data supported the proposed structure.
Electrochemical properties of 2 were investigated by CV measurement. The voltammogram showed an irreversible wave and the first reduction potential was nearly equal to that of azulene (1) [(E1red)pa: 2; -2.03 V, 1; -1.93 V]. On the other hand, its electron donation ability was slight smaller than that of 1 [(E1ox)pc: 2; +0.97 V, 1; +0.64 V]. These results indicated that the pentathiepin moiety would play a role of electron withdrawing group toward an azulene skeleton rather than that of donating one. 1H NMR and UV-VIS spectra of 2 also suggested the withdrawing property; down field shifts of protons [1: δ 7.18 (t like, J = 9.9 Hz, 2H), 7.41 (d, J = 3.7 Hz, 2H), 7.60 (t like, J = 9.9 Hz, 1H), 7.92 (t, J = 3.7 Hz, 1H), 8.36 (d like, J = 9.9 Hz, 2H)] and a red shift of the longest wave-maximum [1: λmax 580 nm (log ε 2.48)].
Various azulene derivatives with S-groups at the 1- and 2-positions could be presented by the conversion of azulenopentathiepin (2). Reduction of 2 with NaBH4 in chlorobenzene/ethanol generated bis(thiolate) (4), which was methylated by iodomethane to give 1,2-bis(methylthio)azulene (5, a reddish violet viscous oil, 63 %). 4 generated with LiAlH4 in THF was treated with methyl chloroformate to afford 1,2-bis(thiocarbonate) (6, a purple viscous oil, 80 %), whereas, in the case with a reactive carbonylation reagent, N,N’-carbonyldiimidazole to afford a cyclic compound, 2-oxo-1,3-dithiole [7, a blue solid, mp 136-137 °C (dec.), 99 %]. The similar reaction with N,N’-thiocarbonyldiimidazole afforded 2-thioxo-1,3-dithiole [8, green needles, mp 187-187.5 °C (dec.), 81 %]. Physical data of 5, 6, 7 and 8 were consistent with their proposed structures, respectively.
It is known that pentathiepins extruded three sulfur atoms to form intermediates dithiets and dithiones, which reacted with alkynes to give 1,4-dithiin compounds.7 Treatment of azulenopentathiepin (2) with PPh3 in THF would generate azuleno-1,2-dithiet and -1,2-dithione as an equilibrium mixture (Scheme 1). The latter carried out a [4 + 2] cycloaddition with dimethyl acetylenedicarboxylate (DMAD) to produce azuleno-1,4-dithin (9, 56 %), which was a yellowish green powder (mp 90-91 °C). Its structure was confirmed by the measurement of instrumental analysis data (1H and 13C NMR, UV-VIS, IR and MS spectra and elemental analysis). In the case without DMAD, the reaction mixture was too complicated to define the structures.
Azulenopentathiepin (2) should be a precursor for an azuleno-1,2-dithiolate ligand having coordination abilities toward soft metal ions such as Pd, Pt, Hg and so on. Treatment of 2 with Pd(PPh3)4 in CH2Cl2 produced mono Pd complex (10, a dark green solid, 6 %) and dinuclear complexes (11a and 11b, dark brown needles, 27 %) as an inseparable mixture of regioisomers (11a : 11b = 1 : 1). 10 was rather unstable and gradually converted into 11a and 11b in CH2Cl2 at rt. FAB-MS spectra of 10 and 11 gave each molecular ion peaks (10: m/z 821 (MH+), 820 (M+), M = C46H36P2106PdS2; 11: m/z 1117 (MH+), 1116 (M+), M = C56H42P2106Pd2S2) together with their isotopic peaks for 104Pd, 105Pd, 108Pd and 110Pd. In 31P NMR spectra, 10 and the unsymmetrical isomer (11b) showed two peaks with a P-P coupling constant arising from different kinds of phosphines, respectively [10: δ 25.3 (d, J = 31.4 Hz), 27.6 (d, J = 31.4 Hz); 11b: δ 33.2 (d, J = 7.2 Hz), 33.9 (d, J = 7.2 Hz)]. In contrast 11a with a center of symmetry gave only one peak at δ 32.8 (s). This reaction involves the following steps: the extrusion of three sulfur atoms from 2 in the presence of PPh3 arising from Pd(PPh3)4 (generation of azuleno-1,2-dithiet, see in Scheme 1), the oxidative addition of Pd(0) into a S-S bond of the dithiet (formation of 10) and the successive dimerization of 10 with elimination of PPh3 (formation of 11a and 11b). It was reported that an oxidative addition of Pd(0) to a thiophene-fused 1,2-dithiin, which was dimerized to give a dinuclear Pd complex.8
In conclusion, we have succeeded in the preparation of a new azulene-fused pentathiepin, azulenopentathiepin, and found that it could be a useful precursor for the conversion into azulenes with S-groups at the 1- and 2-positions. Further work, aimed at the construction of novel azulene-based electron donors from S-substituted azulenes, is in progress.
EXPERIMENTAL
Mps were determined with a Mitamura air-bath apparatus and are uncorrected. 1H and 13C NMR spectra (SiMe4 as the internal standard) were obtained with Bruker AV500, AM400, AV300, AC300 and/or AC200 spectrometers. IR spectra were obtained with a Perkin Elmer System 2000 FT instrument and electronic spectra (UV-VIS) with a JASCO V-560 spectrophotometer. MS spectra were obtained with a JEOL JMS700AM spectrometer and CV with an ALS-600 electrochemical measuring apparatus. Unless otherwise stated the spectra were taken in the following solvents/media: IR, KBr; UV-VIS, CH2Cl2; 1HNMR (500,400, 300 and 200 MHz), CDCl3; MS spectra were taken at 70 eV by electron impact (EI) and/or fast atom bombardment (FAB) method; CV, V vs. Ag/Ag+, GC, Pt wire, 0.1 M TEAP in acetonitrile. The progress of reactions was followed by TLC method using Merck Silica gel 60F254.
General procedure for the reaction of azulene (1) or bis(1-azulyl)sulfides (3) with elemental sulfur. A solution of 1 or 3 with elemental sulfur in the solvent was stirred for the time at the temperature under Ar. After removal of the solvent in vacuo, the residue was dissolved in CH2Cl2. The soluble component was purified by SiO2 column chromatography to give azulenopentathiepin (2) and/or bis(1-azulyl)sulfides (3, an inseparable mixture of sulfides). Atoms of elemental sulfur, solvents, concentrations, times, temperatures and yields were indicated in Table 1.
Azulenopentathiepin (2): a bluish green powder; mp 142-143 °C (dec.); 1H NMR δ 7.42 (t like, J = 9.8 Hz, 1H), 7.50 (t like, J = 9.8 Hz, 1H), 7.60 (s, 1H), 7.77 (t like, J = 9.8 Hz, 1H), 8.32 (d like, J = 9.8 Hz, 1H), 8.72 (d like, J = 9.8 Hz, 1H); 13C NMR δ 123.5, 127.1, 127.2, 127.4, 139.3 (2C), 140.3, 141.3, 142.4, 151.3; UV-VIS (log ε) λmax 605 (2.72), 293 (4.49), 232 (4.25), 223 (4.25); CV (V vs. Ag/Ag+) (Ered)pa -2.72, -2.40, -2.03, (Eox)pc +0.97, +1.29, +1.85, +2.05; MS (FAB, NBA) m/z 287 (MH+), 286 (M+); MS (EI) m/z 286 (M+), 222 (M+-2S). Anal. Calcd for C10H6S5: C, 41.92; H, 2.11. Found: C, 41.73; H, 2.01.
Bis(1-azulyl)sulfides [3 (nave. = 4), from Entry 3 in Table 1]: a green solid; Selected 1H NMR of 3 (n = 12d) δ 7.15 (t like, J = 9.8 Hz, 2H), 7.22 (t like, J = 9.8 Hz, 2H), 7.28 (d, J = 4.1 Hz, 2H), 7.61 (t like, J = 9.8 Hz, 2H), 7.74 (d, J = 4.1 Hz, 2H), 8.24 (d like, J = 9.8 Hz, 2H), 8.78 (d like, J = 9.8 Hz, 2H); Selected 1H NMR of 3 (n = 2) δ 6.80 (t like, J = 9.8 Hz, 2H), 7.16 (t like, J = 9.8 Hz, 2H), 7.32 (d, J = 4.1 Hz, 2H), 7.45 (t like, J = 9.8 Hz, 2H), 7.77 (d like, J = 9.8 Hz, 2H), 7.86 (d, J = 4.1 Hz, 2H), 8.26 (d like, J = 9.8 Hz, 2H); Selected MS (FAB, NBA) m/z 383 [MH+ (n = 4)], 382 [M+ (n = 4)], 351 [MH+ (n = 3)], 350 [M+ (n = 3)], 319 [MH+ (n = 2)], 318 [M+ (n = 2)], 287 [MH+ (n = 1)], 286 [M+ (n = 1)]. Anal. Calcd for C20H14S4 (n = 4): C, 62.79; H, 3.69. Found: C, 62.71; H, 4.02.
The preparation of 1,2-bis(methylthio)azulene (5): To a solution of 2 (16 mg, 5.6 x 10-2 mmol) in dry chlorobenzene (4.5 mL) and abs. EtOH (4.5 mL), 10 mol eq. of NaBH4 was added at rt under N2. The solution was stirred for 15 min at rt and then 200 mol eq. of iodomethane was added and stirred for 1 h. The reaction mixture was quenched with 2 N HCl and the aqueous layer was extracted with ether. The organic layer was dried over MgSO4 and the solvent was removed under reduced pressure. The residue was purified by SiO2 column chromatography to give 5 (7.8 mg, 63 %); a reddish violet viscous oil; 1H NMR δ 2.31 (s, 3H), 2.68 (s, 3H), 7.13 (s, 1H), 7.24 (dd, J = 9.6, 9.2 Hz, 1H), 7.32 (dd, J = 9.8, 9.6 Hz, 1H), 7.51 (dd, J = 9.8, 9.6 Hz, 1H), 8.08 (d, J = 9.2 Hz, 1H), 8.54 (d, J = 9.6 Hz, 1H); UV-VIS (log ε) λmax 535 (2.18), 405 (3.74), 388 (3.64), 317 (4.43), 253 (4.06); MS (FAB, NBA) m/z 221 (MH+), 220 (M+); MS (EI) m/z 220 (M+); HRMS (EI) Calcd for C12H12S2: 220.0380. Found: 220.0383.
The preparation of bis(thiocarbonate) (6), 2-oxo-1,3-dithiole (7) or 2-thioxo-1,3-dithiole (8). To a solution of 2 in dry THF (concentration: 1.8 x 10-2 mM), 10 mol eq. of LiAlH4 was added at 0 °C under Ar. The solution was stirred for 30 min at rt and then 20 mol eq. of methyl chloroformate, N,N’-carbonyldiimidazole or N,N’-thiocarbonyldiimidazole was added and stirred for 10 min at rt. The reaction mixture was quenched with 2 N HCl and the aqueous layer was extracted with ether. The organic layer was dried over MgSO4 and the solvent was removed under reduced pressure. The resulting residue was purified by SiO2 column chromatography to give 6 (80 %), 7 (99 %) or 8 (81 %), respectively.
Bis(thiocarbonate) (6) : a purple viscous oil; 1H NMR δ 3.81 (s, 3H), 3.91 (s, 3H), 7.38 (t like, J = 9.6 Hz, 1H), 7.42 (t like, J = 9.6 Hz, 1H), 7.73 (t like, J = 9.6 Hz, 1H), 7.89 (s, 1H), 8.39 (d like, J = 9.6 Hz, 1H), 8.55 (d like, J = 9.6 Hz, 1H); UV-VIS (log ε) λmax 555 (2.18), 375 (3.58), 345 (3.70), 310 (4.45), 299 (4.45); IR ν 1727; MS (FAB, NBA) m/z 309 (MH+), 308 (M+); MS (EI) m/z 308 (M+); HRMS (EI) Calcd for C14H12O4S2: 308.0177. Found: 308.0181.
2-Oxo-1,3-dithiole (7): a blue solid; mp 136-137 °C (dec.); 1H NMR δ 7.26 (t like, J = 9.8 Hz, 2H), 7.45 (s, 1H), 7.65 (t like, J = 9.8 Hz, 1H), 8.04 (d like, J = 9.8 Hz, 1H), 8.25 (d like, J = 9.8 Hz, 1H); UV-VIS (log ε) λmax 585 (2.11), 386 (3.08), 372 (3.40), 355 (3.36), 312 (4.29), 303 (4.23), 251 (3.71); IR ν 1708; MS (FAB, NBA) m/z 219 (MH+), 218 (M+). Anal. Calcd for C11H6OS2: C, 60.52; H, 2.77. Found: C, 60.31; H, 2.89.
2-Thioxo-1,3-dithiole (8): green needles; mp 187-187.5 °C (dec.); 1H NMR δ 7.30 (t like, J = 9.8 Hz, 2H), 7.38 (s, 1H), 7.71 (t like, J = 9.8 Hz, 1H), 8.10 (d like, J = 9.8 Hz, 1H), 8.32 (d like, J = 9.8 Hz, 1H); UV-VIS (log ε) λmax 602 (2.60), 436 (3.68), 415 (4.36), 399 (4.30), 355 (4.07), 344 (4.08), 325 (4.30), 278 (4.28), 253 (4.01); IR ν 1074; MS (FAB, NBA) m/z 235 (MH+), 234 (M+). Anal. Calcd for C11H6S3: C, 56.37; H, 2.58. Found: C, 56.39; H, 2.57.
The preparation of azuleno-1,4-dithiin (9): Into a mixture of 2 (20 mg, 7.0 x 10-2 mmol) and 130 mol eq. of DMAD in dry THF (7.0 mL), 5.0 mol eq. of PPh3 was added by potions over a 2 h period at rt under N2. The solvent was removed in vacuo and the residue was purified by SiO2 column chromatography to give 9 (13 mg, 56 %); a yellowish green powder; mp 90-91 °C; 1H NMR δ 3.84 (s, 3H), 3.87 (s, 3H), 7.04 (s, 1H), 7.05 (t like, J = 9.8 Hz, 1H), 7.07 (t like, J = 9.8 Hz, 1H), 7.40 (t like, J = 9.8 Hz, 1H), 7.73 (d like, J = 9.8 Hz, 1H), 7.89 (d like, J = 9.8 Hz, 1H); 13C NMR δ 53.3, 53.4, 109.3, 113.8, 125.0, 126.0, 127.2, 131.9, 132.1, 133.0, 134.7, 137.6, 137.7, 143.5, 163.1, 163.9; UV-VIS (log ε) λmax 620 (2.74), 340 (4.62), 270 (4.59); IR ν 1735, 1716; MS (FAB, NBA) m/z 333 (MH+), 332 (M+). Anal. Calcd for C16H12O4S2: C, 57.81; H, 3.64. Found: C, 57.64; H, 3.56.
The reaction of azulenopentathiepin (2) with Pd(PPh3)4: To a solution of 2 (15 mg, 5.2 x 10-2 mmol) in dry CH2Cl2 (3.0 mL), 1.0 mol eq. of Pd(PPh3)4 was added at 0 °C under Ar and stirred for 15 min at the same temperature. The reaction mixture was quenched with saturated aqueous NH4Cl and the aqueous layer was extracted with CH2Cl2. The organic layer was removed under reduced pressure and the residue was purified by short SiO2 column chromatography to give 10 (2.4 mg, 6 %) and an inseparable mixture of regioisomers, 11a and 11b (16 mg, 11a : 11b = 1 : 1, 27 %). 10 was rather unstable and gradually converted to 11a and 11b in CH2Cl2 (concentration: 10-2-10-3 mM) at rt.
Mononuclear Pd complex (10): a dark green solid; 1H NMR δ 6.69 (t like, J = 9.8 Hz, 1H), 6.71 (t like, J = 9.8 Hz, 1H), 6.82 (s, 1H), 7.07 (t like, J = 9.8 Hz, 1H), 7.13-7.38 (m, 18H), 7.42-7.60 (m, 13H), 7.62 (d like, J = 9.8 Hz, 1H); 31P NMR δ 25.3 (d, J = 31.4 Hz, 1P), 27.6 (d, J = 31.4 Hz, 1P); UV-VIS (log ε) λmax 641 (1.95), 446 (3.28), 428 (3.43), 369 (4.20), 352 (4.20), 294 (3.89); MS (FAB, NBA) m/z 825 (MH++4), 824 (M++4), 823 (MH++2), 822 (M++2), 821 (MH+), 820 (MH+-1, M+), 819 (MH+-2, M+-1) 818 (M+-2), M = C46H36P2106PdS2.
Dinuclear Pd complexes (11a and 11b, a mixture of regioisomers): dark brown needles; Selected 1H NMR of 11a δ 6.10 (t like, J = 9.8 Hz, 2H), 6.39 (s, 2H), 6.72 (t like, J = 9.8 Hz, 2H), 6.91 (d like, J = 9.8 Hz, 2H), 7.15-7.38 (m, 20H), 7.49-7.66 (m, 14H); Selected 1H NMR of 11b δ 6.10 (t like, J = 9.8 Hz, 1H), 6.39 (s, 1H), 6.49 (t like, J = 9.8 Hz, 1H), 6.60 (t like, J = 9.8 Hz, 1H), 6.74 (t like, J = 9.8 Hz, 1H), 6.87- 6.93 (m, 3H), 7.10 (t like, J = 9.8 Hz, 1H), 7.11 (t like, J = 9.8 Hz, 1H), 7.15-7.38 (m, 18H), 7.46 (d like, J = 9.8 Hz, 1H), 7.49-7.66 (m, 13H); Selected 31P NMR of 11a δ 32.8 (s, 2P); Selected 31P NMR of 11b δ 33.2 (d, J = 7.2 Hz, 1P), 33.9 (d, J = 7.2 Hz, 1P); UV-VIS (log ε) λmax 606 (2.90), 441 (3.76), 363 (3.80), 337 (3.89), 228 (4.05); MS (FAB, NBA) m/z 1125 (MH++8), 1124 (M++8), 1123 (MH++6), 1122 (M++6), 1121 (MH++4), 1120 (M++4), 1119 (MH++2) 1118 (MH++1, M++2), 1117 (MH+, M++1), 1116 (MH+-1, M+), 1115 (MH+-2, M+-1), 1114 (MH+-3, M+-2), 1113 (MH+-4, M+-3), 1112 (M+-4), M = C56H42P2106Pd2S4. Anal. Calcd for C56H42P2Pd2S4: C, 60.16; H, 3.79. Found: C, 60.57; H, 4.15.
References
1. a) O. Sato, N. Matsuda, S. Yoshioka, A. Takahashi, Y. Sekiguchi, J. Tsunetsugu, and (the late) T. Nozoe, J. Chem. Res. (M), 1998, 635; and J. Chem. Res. (S), 1998, 108; CrossRef b) O. Sato, Y. Koizumi, Y. Sekiguchi, S. Yoshioka, J. Tsunetsugu, Y. Z. Yan, A. Mori, H. Takeshita, and T. Nozoe, Chem. Lett., 2000, 1078; CrossRef c) O. Sato, M. Sato, H. Sugimoto, T. Kuramochi, T. Shirahata, and K. Takahashi, J. Sulfur Chem., 2009, 30, 360. CrossRef
2. a) A. G. Anderson, Jr. and R. N. McDonald, J. Am. Chem. Soc., 1959, 81, 5669; CrossRef b) K. Hafner, H. Patzelt, and H. Kaiser, Ann., 1962, 656, 24; c) L. L. Replogle, R. M. Arluck, and J. R. Maynard, J. Org. Chem., 1965, 30, 2715; CrossRef d) L. L. Replogle, G. C. Peters, and J. R. Maynard, J. Org. Chem., 1969, 34, 2022; CrossRef e) T. Asao, S. Ito, and N. Morita, Tetrahedron Lett., 1989, 30, 6345. CrossRef
3. L. S. Konstantinova and O. A. Rakitin, Chem. Rev., 2004, 104, 2617; and cited references in this review. CrossRef
4. a) F. Fehér and M. Langer, Tetrahedron Lett., 1971, 2125; CrossRef b) A. Alam, M. Kon-no, S. Ogawa, and R. Sato, Tetrahedron, 2007, 63, 927; CrossRef c) L. S. Konstantinova, O. A. Rakitin, and C. W. Rees, Chem. Commun., 2002, 1204; CrossRef d) H. Muraoka, S. Ogawa, N. Nagahora, Y. Kawai, and R. Sato, Bull. Chem. Soc. Jpn., 2005, 78, 2026. CrossRef
5. R. D. Brown, Trans. Faraday Soc., 1948, 44, 984. CrossRef
6. H. Fritz and C. D. Weis, Tetrahedron Lett., 1974, 18, 1659. CrossRef
7. a) R. Sato, K. Chino, and M. Saito, Sulfur Lett., 1990, 10, 233; b) S. A. Amelichev, L. S. Konstantinova, N. V. Obruchnikova, O. A. Rakitin, and C. W. Rees, Org. Lett., 2006, 8, 4529. CrossRef
8. K. Kudo, T. Okamoto, and S. Yamaguchi, Organometallics, 2006, 25, 2374. CrossRef