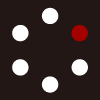
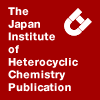
HETEROCYCLES
An International Journal for Reviews and Communications in Heterocyclic ChemistryWeb Edition ISSN: 1881-0942
Published online by The Japan Institute of Heterocyclic Chemistry
e-Journal
Full Text HTML
Received, 15th June, 2012, Accepted, 31st July, 2012, Published online, 8th August, 2012.
DOI: 10.3987/COM-12-S(N)34
■ Direct Asymmetric α-Allylation of Ketones with Allylic Alcohols via Pd/Enamine Cooperative Function
Shigeo Yasuda, Naoya Kumagai,* and Masakatsu Shibasaki*
Institute of Microbial Chemistry, 3-14-23 Kamiosaki, Shinagawa-ku, Tokyo 141-0021, Japan
Abstract
Direct asymmetric α-allylation of ketones with allylic alcohols is described. The combination of palladium with a new phosphine ligand bearing a chiral proline moiety promoted the reaction to afford the corresponding α-allylated ketones in moderate yield and enantioselectivity.The palladium-catalyzed asymmetric Tsuji-Trost allylation is a highly attractive and powerful tool in organic synthesis.1 Typically, preactivation of allylic alcohols as allylic acetates and carbonates is required for the efficient formation of π-allyl electrophiles, and a stoichiometric amount of byproducts are co-produced. In this regard, the direct use of allylic alcohols as substrates would be highly attractive because the extra step required for the preparation of activated allylic alcohols is avoided, and the byproduct is water.2,3 Recent advances in transition-metal catalyzed asymmetric Tsuji-Trost allylations allow for the direct use of allylic alcohols as allylic electrophiles.4 The variety of compatible nucleophiles, however, is limited to heteroatom nucleophiles,5 metal enolates,6 and indoles,7,8 some of which require the combined use of a stoichiometric amount of Brønsted acid or Lewis acid to promote the reaction, and simple carbonyl compounds have not been implemented in this class of reaction. Therefore, the development of asymmetric allylations with allylic alcohols using simple carbonyl compounds is highly desirable. Very recently, the direct catalytic asymmetric α-allylation of aldehydes with allylic alcohols was reported by List and coworkers.9 For an analogous process using less reactive simple ketones as nucleophiles,10,11,12 there is only one example in a racemic system in which a combination of amine organocatalysis and Pd catalysis is used, and the process has not been rendered asymmetric.2i In that system, the enamine nucleophile generated in situ from the amine and ketone undergoes nucleophilic attack on an in situ-generated π-allyl Pd complex.13 Inspired by this work, we hypothesized that by covalently tethering a Pd complex and a chiral proline unit, dual activation of allylic alcohol and ketone would act in close proximity to efficiently promote the reaction in an asymmetric environment (Scheme 1). Herein we report the first example of a direct asymmetric α-allylation of simple ketones with simple allylic alcohols using a tethered complex that harnesses the cooperative function of π-allyl Pd activation and enamine activation.
To realize the dual activation of allylic alcohol and ketone as delineated in Scheme 1, we designed a chiral ligand 1a bearing a proline and 2-(diphenylphosphino)benzylamine, which are covalently tethered by an amide linkage.14 Upon complexation with Pd0, the π-allyl Pd electrophile would be formed from allylic alcohols close to the enamine nucleophile generated in situ from the pyrrolidino group in 1a and the ketone substrate (Figure 1). As an analogous ligand, 1b and 1c bearing fluoro-substituted proline units were also synthesized, and 1a–c were evaluated in an α-allylation of cyclohexanone (3a) with 3-phenyl-2-propen-1-ol (2a) (Table 1).
The reactions were conducted in the presence of [(η3-allyl)PdCl]2 and ligand 1 in a 1:3 ratio in DMSO at room temperature.15 As expected, the reaction with ligand 1a proceeded to afford the desired product 4a with moderate enantioselectivity, although the yield was only 10% (Table 1, Entry 1). Changing the ratio of Pd/1a to 1:1 or 1:2 led to a significantly lower conversion. The yield increased slightly with a higher loading of Pd and ligand 1a (Entry 2). Ligand 1b, bearing a 4-fluoro-substituted proline unit, exhibited a higher reaction efficiency and afforded 4a in better yield, albeit with lower enantioselectivity (Entry 3). The best result was obtained with the reaction using ligand 1c bearing a 4,4-difluoro-substituted proline unit, which afforded the highest yield and modest enantioselectivity (Entry 4). To examine the cooperative function of π-allyl Pd activation and enamine activation in close proximity, the following control experiment was performed. The reaction with 1d, a ligand analogous to 1a lacking a phosphine moiety, and PPh3 hardly proceeded, and resulted in lower enantioselectivity (Entry 5), suggesting that linkage of the phosphine unit and the chiral proline unit allows for dual activation of the allylic alcohol and ketone in proximity. Decreasing the amount of Pd salt and ligand 1 led to a remarkable loss of conversion.
The scope of the present asymmetric α-allylation using the [(η3-allyl)PdCl]2/1c system is summarized in Table 2. In addition to 2a, the present system was applicable to substituted cinnamyl alcohols 2b–e. Although similar enantioselectivity was observed irrespective of the electronic nature of substituents, an electron-donating substituent led to decreased reaction efficiency. The reaction of oxygen-containing heterocyclic ketone 3b produced the corresponding product 4f, albeit with low yield and enantioselectivity (Table 2, Entry 6).
The proposed mechanism for the present reaction is shown in Scheme 2. Initially, enamine intermediate A is produced by reaction of the pyrrolidino group in ligand 1 with a ketone. Complexation of A and [(η3-allyl)PdCl]2 through a phosphine moiety forms π-allyl PdII complex B’, and a subsequent intramolecular nucleophilic attack of enamine to π-allyl PdII generates iminium cation C’, in which PdII is reduced to Pd0. Hydrolysis of C’ leads to intermediate D, and the release of α-allyl ketone 4’16 and HCl. There is another possible pathway from C’ to E, in which a ligand exchange of C’ with A occurs and forms E, releasing iminium cation G. Pd0 complex D is responsible for the following catalytic cycle. Coordination of allylic alcohol to E forms Pd0 olefin complex F and oxidative addition occurs with the aid of HCl to generate π-allyl PdII complex B. Subsequent intramolecular nucleophilic attack of enamine on π-allyl PdII and hydrolysis of iminium cation C to release product 4 proceed in a manner similar to that of the initial cycle. We assume that the substituent on the proline unit slightly changes the conformation of the ring, which affects the reactivity and enantioselectivity in this intramolecular attack of enamine, and the most favorable conformation is attained with ligand 1c. Although the Pd/1 = 1:1 complex is shown in Scheme 2 for clarity, spectroscopic analysis of the Pd/1 mixture was unsuccessful and therefore the structure of Pd/1 is not clear. Based on the fact that 3 equivalents of ligand 1 to Pd are required for efficient conversion, an active Pd complex can be the Pd/1 complex in a ratio of 1:2 or 1:3. We assume that another reason for the requirement of excessive amounts of ligand 1 to Pd (3 equiv to Pd, 0.9 equiv to allylic alcohol) is the reluctant hydrolysis of iminium cations (C, C’, G, and G’). If the hydrolysis does not proceed easily, ligand 1 is arrested as an inactive form.
In summary, we demonstrated the allylation of simple ketones at the α-position with simple allylic alcohols under a new system consisting of Pd and phosphine ligand bearing a chiral proline unit. Linkage of a phosphine ligand and a chiral proline unit were essential for enantioinduction. There is still room for improvement, however, with regard to the unsatisfactory yield, low enantioselectivity, and requirement of high loading of Pd and ligand 1. Nevertheless, the reaction presented in this report is the first example of the direct asymmetric α-allylation of simple ketones with simple allylic alcohols.
EXPERIMENTAL
General. Infrared (IR) spectra were recorded on a HORIBA FT210 Fourier transform infrared spectrophotometer. NMR spectra were recorded on JEOL ECS-400 spectrometer. Chemical shifts for proton are reported in parts per million downfield from tetramethylsilane and are referenced to residual protonium in the NMR solvent (CDCl3: δ 7.26 ppm). For 13C NMR, chemical shifts are reported in the scale relative to NMR solvent (CDCl3: δ 77.0 ppm). For 19F NMR, chemical shifts are reported in the scale relative to CF3COOH (δ –76.5 ppm) as an external reference. For 31P NMR, chemical shifts are reported in the scale relative to H3PO4 (δ 0.0 ppm in D2O) as an external reference. NMR data are reported as follows: chemical shifts, multiplicity (s: singlet, d: doublet, dd: doublet of doublets, t: triplet, q: quartet, m; multiplet, br: broad signal), coupling constant (Hz), and integration. Optical rotation was measured using a 2 mL cell with a 1.0 dm path length on a JASCO polarimeter P-1030. High-resolution mass spectra (ESI TOF (+)) were measured on ThermoFisher Scientific LTQ Orbitrap XL. HPLC analysis was conducted on a JASCO HPLC system equipped with Daicel chiral-stationary-phase columns (0.46 cm φ x 25 cm). The absolute configuration of 4a was determined by comparing the sign of optical rotation with the literature data reported by Koga et al.17 The absolute configuration of other products was assigned by analogy.
Materials. Unless otherwise noted, materials were purchased from commercial suppliers and were used without purification. THF and CH2Cl2 were purified by passing through a solvent purification system (Glass Contour). Dry DMSO and CHCl3 were purchased from Kanto Chemical Co. Ltd. and used as received. [(η3-allyl)PdCl]2 was purchased from Aldrich and used as received. Chiral proline derivatives used for synthesis of ligands 1 were purchased from Aldrich and WATANABE CHEMICAL Ind. Ltd. Allylic alcohol 2a was purchased from Kanto Chemical Co. Ltd. 2b was prepared by following the literature procedure.18 Column chromatography was performed with Silica gel Merck 60 (230–400 mesh ASTM).
Preparation of Allylic Alcohols 2c–e. These compounds were synthesized by the following procedure.
(E)-3-(4-Methoxyphenyl)prop-2-en-1-ol (2d). To a 300 mL pear-shaped flask equipped with a magnetic stirring bar, 4-methoxycinnamaldehyde (5.68 g, 35.0 mmol) and MeOH (140 mL) were added at room temperature. The resulting solution was cooled to 0 °C and then NaBH4 (1.59 g, 42.0 mmol) was added to the solution. After stirring for 30 min at 0 °C, sat. NaHCO3 aq. (200 mL) and CH2Cl2 (200 mL) were sequentially added to the reaction mixture. After stirring for 5 min at room temperature, the organic layer and aqueous layer were separated. Aqueous layer was extracted with CH2Cl2 (3 x 100 mL) and combined organic layer was dried over Na2SO4. After filtration, the filtrate was concentrated. The resulting residue was purified by silica gel column chromatography system (combiflash®: n-hexane/EtOAc as eluent) to afford 2d (5.55 g, 97%) as a white solid; 1H NMR (CDCl3) δ 7.32 (d, J = 8.7 Hz, 2H), 6.85 (d, J = 8.7 Hz, 2H), 6.55 (d, J = 16.0 Hz, 1H), 6.23 (dt, J = 6.0, 16.0 Hz, 1H), 4.29 (d, J = 6.0 Hz, 2H), 3.80 (s, 3H), 1.75 (brs, 1H); 13C NMR (CDCl3) δ 159.2, 130.8, 129.4, 127.6, 126.2, 113.9, 63.8, 55.2.
(E)-3-(4-Fluorophenyl)prop-2-en-1-ol (2c): white solid; 1H NMR (CDCl3) δ 7.33–7.30 (m, 2H), 7.01–6.97 (m, 2H), 6.55 (d, J = 16.0 Hz, 1H), 6.26 (dt, J = 5.7, 16.0 Hz, 1H), 4.29 (d, J = 5.7 Hz, 2H), 2.32 (brs, 1H); 13C NMR (CDCl3) δ 162.3 (d, JC–F = 245.0 Hz), 132.8 (d, JC–F = 3.8 Hz), 129.8, 128.2 (d, JC–F = 1.9 Hz), 127.9 (d, JC–F = 7.6 Hz), 115.4 (d, JC–F = 21.9 Hz), 63.4; 19F NMR (CDCl3) δ –114.1.
(E)-3-(4-(N,N-Dimethylamino)phenyl)prop-2-en-1-ol (2e): yellow solid; 1H NMR (CDCl3) δ 7.26 (d, J = 9.0 Hz, 2H), 6.66 (d, J = 9.0 Hz, 2H), 6.55 (d, J = 15.8 Hz, 1H), 6.15 (dt, J = 6.0, 15.8 Hz, 1H), 4.23 (d, J = 6.0 Hz, 2H), 3.27 (brs, 1H), 2.92 (s, 6H); 13C NMR (CDCl3) δ 149.7, 131.0, 127.2, 125.1, 124.0, 112.3, 63.5, 40.2.
NMR data of 2c,19 2d,20 and 2e20 are identical to literature data.
Preparation of Phosphine Ligands 1a–c. These compounds were synthesized over 4 steps starting from commercially available 2-(diphenylphosphino)benzoic acid.
2-(Diphenylphosphino)benzamide (S1). To a 100 mL pear-shaped flask equipped with a magnetic stirring bar, 2-(diphenylphosphino)benzoic acid (2.90 g, 10.0 mmol), and CHCl3 (40 mL) were added at room temperature. To the resulting suspension, (NH4)HCO3 (3.64 g, 46.0 mmol) and N-ethoxycarbonyl-2-ethoxy-1,2-dihydroquinone (3.46 g, 14.0 mmol) were added sequentially. After stirring for 28 h at room temperature, the reaction mixture was diluted with CH2Cl2 (350 mL). The mixture was washed with 1N HCl aq. (3 x 100 mL), 1N NaOH aq. (2 x 100 mL), and brine (100 mL), and then dried over Na2SO4. After filtration, the filtrate was concentrated. The resulting residue was purified by silica gel column chromatography (eluent: n-hexane/EtOAc = 1/1 to 1/2) to afford S1 (1.91 g, 62%) as a white solid; 1H NMR (CDCl3) δ 7.55–7.52 (m, 1H), 7.27–7.16 (m, 12H), 6.91–6.88 (m, 1H), 6.07 (brs, 2H); 13C NMR (CDCl3) δ 170.8, 139.8 (d, JC–P = 24.8 Hz), 137.1 (d, JC–P = 10.5 Hz), 136.6 (d, JC–P = 21.9 Hz), 134.2, 133.9, 133.7, 130.4, 128.6 (d, JC–P = 9.5 Hz), 128.5 (d, JC–P = 7.6 Hz), 127.9 (d, JC–P = 3.8 Hz); 31P NMR (CDCl3) δ –8.6.
NMR data are identical to literature data.21
(2-(Diphenylphosphino)phenyl)methylamine (S2). To a 300 mL pear-shaped flask equipped with a magnetic stirring bar, S1 (1.59 g, 5.21 mmol), and THF (100 mL) were added at room temperature. The resulting solution was cooled to 0 °C and then LiAlH4 (593 mg, 15.6 mmol) was added to the solution. After stirring for 12 h at room temperature, the reaction mixture was cooled to 0 °C, and then H2O (15 mL), 8% NaOH aq. (30 mL), H2O (30 mL) were added sequentially. The resulting mixture was extracted with CH2Cl2 (2 x 100 mL) and dried over Na2SO4. After filtration, the filtrate was concentrated. The resulting residue was purified by silica gel column chromatography (eluent: n-hexane/EtOAc = 1/2 to EtOAc only) to afford S2 (1.15 g, 76%) as a white solid; 1H NMR (CDCl3) δ 7.46–7.43 (m, 1H), 7.37–7.25 (m, 11H), 7.16 (ddd, J = 1.2, 7.6, 8.7 Hz, 1H), 6.91–6.88 (m, 1H), 4.03 (d, J = 1.6 Hz, 2H), 1.49 (brs, 2H); 13C NMR (CDCl3) δ 147.3 (d, JC–P = 22.9 Hz), 136.3 (d, JC–P = 9.5 Hz), 135.0 (d, JC–P = 13.4 Hz), 133.8 (d, JC–P = 19.1 Hz), 133.4, 129.2, 128.7, 128.5 (d, JC–P = 19.1 Hz), 127.9 (d, JC–P = 4.8 Hz), 127.0, 45.1 (d, JC–P = 21.9 Hz); 31P NMR (CDCl3) δ –15.3.
NMR data are identical to literature data.22
tert-Butyl (S)-2-((2-(diphenylphosphino)phenyl)methylcarbamoyl)pyrrolidine-1-carboxylate (S3). To a 50 mL pear-shaped flask equipped with a magnetic stirring bar, N-(tert-butoxycarbonyl)-L-proline (513 mg, 2.38 mmol), N-ethoxycarbonyl-2-ethoxy-1,2-dihydroquinone (607 mg, 2.45 mmol), and CH2Cl2 (6.0 mL) were added at room temperature. The resulting solution was cooled to 0 °C, and then S2 (715 mg, 2.45 mmol) in CH2Cl2 (6.0 mL) was added. After stirring for 12 h at room temperature, the reaction mixture was washed with 1N HCl aq. (3 x 50 mL), sat. NaHCO3 aq. (2 x 50 mL), H2O (50 mL), and brine (2 x 50 mL), and then dried over Na2SO4. After filtration, the filtrate was concentrated. The resulting residue was purified by silica gel column chromatography (eluent: n-hexane/EtOAc = 2/1) to afford S3 (929 mg, 80%) as a white solid.
(S)-N-(2-(Diphenylphosphino)phenyl)methylpyrrolidine-2-carboxyamide (1a). To a 100 mL pear-shaped flask equipped with a magnetic stirring bar, S3 (1.03 g, 2.12 mmol) and CH2Cl2 (2.5 mL) were added at room temperature. The resulting solution was cooled to 0 °C, and then 4N HCl/CPME (2.12 mL, 8.48 mmol) was added. After stirring for 14 h at room temperature, sat. NaHCO3 aq. (60 mL) was added to the reaction mixture. After stirring for additional 20 min, the resulting mixture was extracted with CH2Cl2 (3 x 60 mL). The combined organic layer was dried over Na2SO4. After filtration, the filtrate was concentrated. The resulting residue was purified by silica gel column chromatography (eluent: CHCl3 only to CHCl3/MeOH = 20/1) to afford 1a (750 mg, 92%) as a colorless viscous oil; IR (neat) 3326, 3054, 2970, 2871, 1661, 1513, 1478, 1434, 1092, 747, 697, 665 cm–1; 1H NMR (CDCl3) δ 7.80 (brs, 1H), 7.42 (dd, J = 4.4, 6.8 Hz, 1H), 7.35–7.32 (m, 7H), 7.29–7.23 (m, 4H), 7.19 (dd, J = 7.6, 7.6 Hz, 1H), 6.91 (dd, J = 4.4, 7.6 Hz, 1H), 4.73 (dd, J = 6.2, 14.7 Hz, 1H), 4.60 (dd, J = 5.7, 14.7 Hz, 1H), 3.54 (dd, J = 5.2, 8.9 Hz, 1H), 2.85 (ddd, J = 6.6, 6.9, 10.1 Hz, 1H), 2.71 (ddd, J = 6.2, 6.2, 10.1 Hz, 1H), 2.10–2.01 (m, 1H), 1.87–1.79 (m, 2H), 1.64–1.57 (m, 2H); 13C NMR (CDCl3) δ 174.7, 142.8 (d, JC–P = 24.8 Hz), 136.2 (d, JC–P = 9.5 Hz), 135.5 (d, JC–P = 14.3 Hz), 133.8 (d, JC–P = 7.6 Hz), 133.6 (d, JC–P = 6.7 Hz), 129.2, 129.0 (d, JC–P = 5.7 Hz), 128.7 (d, JC–P = 4.8 Hz), 128.5 (d, JC–P = 1.9 Hz), 128.4 (d, JC–P = 2.9 Hz), 127.5, 60.3, 46.9, 41.6 (d, JC–P = 22.9 Hz), 30.5, 25.9; 31P NMR (CDCl3) δ –15.4; HRMS (ESI) calcd. for C24H26N2OP m/z 389.1777 [M+H]+, found 389.1780; [α]D21 –18.0 (c 2.0, CHCl3).
1b and 1c were synthesized by the above procedure using commercially available N-(tert-butoxycarbonyl)-cis-4-fluoro-L-proline and N-(tert-butoxycarbonyl)-4,4-difluoro-L-proline,23 respectively, instead of N-(tert-butoxycarbonyl)-L-proline.
N-(2-(Diphenylphosphino)phenyl)methyl-(4S)-fluoropyrrolidine-(2S)-carboxyamide (1b): colorless viscous oil; IR (neat) 3336, 3005, 1660, 1520, 1478, 1434, 1271, 1217, 1112, 956, 747, 698, 666 cm–1; 1H NMR (CDCl3) δ 7.75 (brs, 1H), 7.44–7.41 (m, 1H), 7.33–7.20 (m, 11H), 7.15 (dd, J = 7.6, 7.6 Hz, 1H), 6.88 (dd, J = 4.6, 7.6 Hz, 1H), 5.07 (ddd, J = 3.7, 3.9, 53.2 Hz, 1H), 4.72 (dd, J = 6.2, 14.9 Hz, 1H), 4.58 (dd, J = 6.0, 14.9 Hz, 1H), 3.59 (dd, J = 3.0, 10.3 Hz, 1H), 3.15 (dd, J = 12.1, 21.5 Hz, 1H), 3.01 (ddd, J = 3.7, 12.1, 35.7 Hz, 1H), 2.41–2.32 (m, 1H), 2.26–2.09 (m, 1H), 1.85 (brs, 1H); 13C NMR (CDCl3) δ 173.6, 142.6 (d, JC–P = 24.8 Hz), 136.2 (d, JC–P = 9.5 Hz), 136.1 (d, JC–P = 9.5 Hz), 135.2 (d, JC–P = 14.3 Hz), 133.9, 133.7 (d, JC–P = 9.5 Hz), 133.5 (d, JC–P = 10.5 Hz), 129.1, 128.6 (d, JC–P = 2.9 Hz), 128.5 (d, JC–P = 1.9 Hz), 128.4 (d, JC–P = 2.9 Hz), 127.4, 93.4 (d, JC–F = 175.4 Hz), 58.9, 53.0 (d, JC–F = 21.9 Hz), 41.6 (d, JC–P = 23.8 Hz), 37.3 (d, JC–F = 21.9 Hz); 19F NMR (CDCl3) δ –173.4–173.9 (m); 31P NMR (CDCl3) δ –15.3; HRMS (ESI) calcd. for C24H25FN2OP m/z 407.1683 [M+H]+, found 407.1685; [α]D21 –13.5 (c 1.0, CHCl3).
(S)-N-(2-(Diphenylphosphino)phenyl)methyl-4,4-difluoropyrrolidine-2-carboxyamide (1c): colorless viscous oil; IR (neat) 3330, 3055, 3004, 1667, 1519, 1478, 1434, 1358, 1249, 1157, 1093, 746, 698 cm–1; 1H NMR (CDCl3) δ 7.59 (brs, 1H), 7.45 (dd, J = 4.6, 7.3 Hz, 1H), 7.38–7.36 (m, 7H), 7.30–7.22 (m, 5H), 6.95 (ddd, J = 0.9, 4.6, 7.6 Hz, 1H), 4.77 (dd, J = 6.4, 14.4 Hz, 1H), 4.64 (dd, J = 5.5, 14.4 Hz, 1H), 3.66 (dd, J = 7.1, 9.2 Hz, 1H), 3.14–3.06 (m, 1H) 2.92–2.82 (m, 1H), 2.59–2.48 (m, 1H), 2.37–2.24 (m, 1H), 1.90 (brs, 1H); 13C NMR (CDCl3) δ 171.7, 142.3 (d, JC–P = 25.7 Hz), 136.3 (d, JC–P = 1.9 Hz), 136.2 (d, JC–P = 1.9 Hz), 135.7 (d, JC–P = 14.3 Hz), 134.0, 133.9 (d, JC–P = 7.6 Hz), 133.7 (d, JC–P = 6.7 Hz), 130.1 (dd, JC–F = 246.0, 251.7 Hz), 129.6 (d, JC–P = 4.8 Hz), 129.4, 128.8 (d, JC–P = 3.8 Hz), 128.6 (d, JC–P = 2.9 Hz), 128.5 (d, JC–P = 3.8 Hz) 127.9, 58.5–58.4 (m), 52.9 (dd, JC–F = 28.6, 28.6 Hz), 42.2 (d, JC–P = 21.0 Hz), 38.1 (dd, JC–F = 24.8, 25.8 Hz); 19F NMR (CDCl3) δ –99.1–99.9 (m), –104.9–105.6 (m); 31P NMR (CDCl3) δ –15.6; HRMS (ESI) calcd. for C24H24F2N2OP m/z 425.1589 [M+H]+, found 425.1590; [α]D21 –8.3 (c 1.0, CHCl3).
(S)-N-Benzylpyrrolidine-2-carboxyamide (1d). This compound was synthesized by the same procedure as for S3 and 1a starting from commercially available N-(tert-butoxycarbonyl)-L-proline and benzylamine; colorless viscous oil; IR (neat) 3316, 3062, 3030, 2966, 2871, 1655, 1522, 1454, 1360, 1249, 1104, 733, 699 cm–1; 1H NMR (CDCl3) δ 7.93 (brs, 1H), 7.25–7.21 (m, 2H), 7.18–7.15 (m, 3H), 4.33 (d, J = 6.0 Hz, 2H), 3.68 (dd, J = 5.3, 9.2 Hz, 1H), 2.89 (ddd, J = 6.6, 6.9, 10.3 Hz, 1H), 2.77 (ddd, J = 6.2, 6.4, 10.3 Hz, 1H), 2.12 (brs, 1H), 2.10–2.01 (m, 1H), 1.89–1.81 (m, 1H), 1.66–1.55 (m, 2H); 13C NMR (CDCl3) δ 175.0, 138.5, 128.4, 127.3, 127.0, 60.4, 47.0, 42.6, 30.6, 26.0; HRMS (ESI) calcd. for C12H17N2O m/z 205.1335 [M+H]+, found 205.1337; [α]D21 –45.7 (c 1.0, CHCl3).
NMR data are identical to literature data.24
Typical Procedure for the Direct Asymmetric α-Allylation of Ketones 3 with Allylic Alcohols 2.
(R)-2-((E)-3-Phenylprop-2-en-1-yl)cyclohexanone (4a). To a flame dried test tube equipped with a stirring bar and 3-way glass stopcock, [(η3-allyl)PdCl]2 (8.2 mg, 0.023 mmol), 3-phenyl-prop-2-en-1-ol (2a, 20 mg, 0.15 mmol) in DMSO (0.60 mL), cyclohexanone (44 mg, 0.45 mmol), and DMSO solution of ligand 1c (1.35 mL, 0.135 mmol, 0.10 M) were added sequentially at room temperature. After stirring for 20 h at room temperature, EtOAc (5 mL) and H2O (5 mL) were added to the reaction mixture. After separation of organic layer and aqueous layer, aqueous layer was extracted with EtOAc (3 x 5 mL). The combined organic layer was filtered through a short pad of silica gel with EtOAc as eluent, and the filtrate was concentrated. The resulting residue was purified by silica gel column chromatography (eluent: n-hexane/EtOAc = 40/1 to 20/1) to afford 4a (21.1 mg, 66%) as a colorless oil; 1H NMR (CDCl3) δ 7.37–7.27 (m, 4H), 7.19 (t, J = 7.1 Hz, 1H), 6.40 (d, J = 15.8 Hz, 1H), 6.20 (ddd, J = 6.6, 8.0, 15.8 Hz, 1H), 2.71–2.64 (m, 1H), 2.47–2.39 (m, 2H), 2.36–2.29 (m, 1H), 2.22–2.12 (m, 2H), 2.09–2.04 (m, 1H), 1.90–1.85 (m, 1H), 1.74–1.61 (m, 2H), 1.46–1.36 (m, 1H); 13C NMR (CDCl3) δ 212.5, 137.5, 131.6, 128.4, 128.3, 127.0, 126.0, 50.7, 42.1, 33.5, 33.0, 27.9, 25.0; [α]D21 16.3 (c 1.1, CHCl3, 55% ee); HPLC [DAICEL CHIRALCEL OD-H, detection at 254 nm, 9:1 n-hexane/iPrOH, flow rate = 1.0 mL/min, tR = 5.7 min (minor), 6.5 min (major)].
NMR data are identical to literature data.2i
(R)-2-((E)-3-(4-Trifluoromethylphenyl)prop-2-en-1-yl)cyclohexanone (4b): colorless oil; IR (neat) 2936, 2863, 1711, 1614, 1449, 1414, 1326, 1164, 1122, 1067, 1016, 971, 858 cm–1; 1H NMR (CDCl3) δ 7.52 (d, J = 8.2 Hz, 2H), 7.40 (d, J = 8.2 Hz, 2H), 6.41 (d, J = 16.0 Hz, 1H), 6.35–6.27 (m, 1H), 2.71–2.65 (m, 1H), 2.48–2.40 (m, 2H), 2.36–2.28 (m, 1H), 2.21–2.13 (m, 2H), 2.10–2.02 (m, 1H), 1.90–1.83 (m, 1H), 1.75–1.61 (m, 2H), 1.46–1.36 (m, 1H); 13C NMR (CDCl3) δ 212.2, 140.9, 131.3, 130.3, 128.7 (q, JC–F = 32.4 Hz), 126.1, 125.3 (q, JC–F = 3.8 Hz), 124.2 (q, JC–F = 269.8 Hz), 50.5, 42.1, 33.7, 33.0, 27.9, 25.0; 19F NMR (CDCl3) δ –62.2; HRMS (ESI) calcd. for C16H18F3O m/z 283.1304 [M+H]+, found 283.1304; [α]D21 11.8 (c 1.1, CHCl3, 54% ee); HPLC [DAICEL CHIRALCEL OJ-3, detection at 254 nm, 99:1 n-hexane/iPrOH, flow rate = 1.0 mL/min, tR = 14.6 min (minor), 15.6 min (major)].
(R)-2-((E)-3-(4-Fluorophenyl)prop-2-en-1-yl)cyclohexanone (4c): colorless oil; IR (neat) 3036, 2935, 2861, 1709, 1601, 1508, 1448, 1430, 1227, 1158, 1129, 970, 852, 823 cm–1; 1H NMR (CDCl3) δ 7.60–7.56 (m, 2H), 7.26 (dd, J = 8.7, 8.9 Hz, 2H), 6.64 (d, J = 15.8 Hz, 1H), 6.40 (ddd, J = 6.6, 7.8, 15.8 Hz, 1H), 2.98–2.91 (m, 1H), 2.75–2.67 (m, 2H), 2.65–2.58 (m, 1H), 2.49–2.34 (m, 3H), 2.18–2.15 (m, 1H), 2.03–1.91 (m, 2H), 1.75–1.65 (m, 1H); 13C NMR (CDCl3) δ 212.5, 161.9 (d, JC–F = 244.1 Hz), 133.7 (d, JC–F = 3.8 Hz), 130.4, 128.1 (d, JC–F = 1.9 Hz), 127.4 (d, JC–F = 7.6 Hz), 115.3 (d, JC–F = 21.0 Hz), 50.7, 42.1, 33.6, 33.0, 27.9, 25.0; 19F NMR (CDCl3) δ –115.3; HRMS (ESI) calcd. for C15H18FO m/z 233.1336 [M+H]+, found 233.1337; [α]D21 15.5 (c 0.7, CHCl3, 56% ee); HPLC [DAICEL CHIRALPAK AY-3, detection at 254 nm, 9:1 n-hexane/iPrOH, flow rate = 1.0 mL/min, tR = 8.0 min (minor), 9.5 min (major)].
(R)-2-((E)-3-(4-Methoxyphenyl)prop-2-en-1-yl)cyclohexanone (4d): colorless oil; IR (neat) 3583, 2934, 2860, 1708, 1607, 1510, 1464, 1446, 1290, 1248, 1175, 1128, 1035, 969 cm–1; 1H NMR (CDCl3) δ 7.26 (d, J = 8.7 Hz, 2H), 6.83 (d, J = 8.7 Hz, 2H), 6.33 (d, J = 15.8 Hz, 1H), 6.05 (ddd, J = 6.6, 8.0, 15.8 Hz, 1H), 3.79 (s, 3H), 2.68–2.61 (m, 1H), 2.44–2.28 (m, 3H), 2.20–2.03 (m, 3H), 1.89–1.85 (m, 1H), 1.74–1.60 (m, 2H), 1.46–1.35 (m, 1H); 13C NMR (CDCl3) δ 212.7, 158.7, 130.9, 130.3, 127.0, 126.1, 113.9, 55.3, 50.8, 42.1, 33.5, 33.0, 27.9, 25.0; HRMS (ESI) calcd. for C16H20NaO2 m/z 267.1356 [M+Na]+, found 267.1356; [α]D21 18.5 (c 0.7, CHCl3, 60% ee); HPLC [DAICEL CHIRALCEL OD-H, detection at 254 nm, 9:1 n-hexane/iPrOH, flow rate = 1.0 mL/min, tR = 6.6 min (minor), 7.1 min (major)].
(R)-2-((E)-3-(4-(N,N-Dimethylamino)phenyl)prop-2-en-1-yl)cyclohexanone (4e): yellow oil; IR (neat) 2932, 2859, 2801, 1708, 1610, 1521, 1445, 1352, 1223, 1188, 1165, 1127, 966, 814 cm–1; 1H NMR (CDCl3) δ 7.23 (d, J = 8.9 Hz, 2H), 6.67 (d, J = 8.9 Hz, 2H), 6.30 (d, J = 15.6 Hz, 1H), 5.98 (ddd, J = 6.6, 8.0, 15.6 Hz, 1H), 2.94 (s, 6H), 2.68–2.61 (m, 1H), 2.44–2.28 (m, 3H), 2.21–2.00 (m, 3H), 1.88–1.85 (m, 1H), 1.74–1.59 (m, 2H), 1.46–1.35 (m, 1H); 13C NMR (CDCl3) δ 212.8, 149.7, 131.3, 126.8, 126.2, 123.9, 112.5, 51.0, 42.1, 40.6, 33.4, 33.0, 27.9, 25.0; HRMS (ESI) calcd. for C17H24NO m/z 258.1852 [M+H]+, found 258.1855; [α]D21 24.0 (c 0.1, CHCl3, 52% ee); HPLC [DAICEL CHIRALPAK AY-H, detection at 254 nm, 9:1 n-hexane/iPrOH, flow rate = 1.0 mL/min, tR = 19.1 min (minor), 21.7 min (major)].
(R)-3-((E)-3-Phenylprop-2-en-1-yl)-4H-pyran-4-one (4f): colorless oil; 1H NMR (CDCl3) δ 7.33–7.27 (m, 4H), 7.22–7.18 (m, 1H), 6.41 (d, J = 15.6 Hz, 1H), 6.15 (ddd, J = 6.2, 8.0, 15.6 Hz, 1H), 4.22–4.16 (m, 2H), 3.76 (ddd, J = 3.4, 10.8, 14.4 Hz, 1H), 3.47 (dd, J = 9.2, 11.4 Hz, 1H), 2.72–2.58 (m, 3H), 2.44 (dt, J = 3.4, 14.4 Hz, 1H), 2.24–2.16 (m, 1H); 13C NMR (CDCl3) δ 207.6, 137.1, 132.2, 128.5, 127.2, 126.6, 126.0, 72.2, 68.6, 51.2, 42.4, 29.3; [α]D21 15.0 (c 0.4, CHCl3, 36% ee); HPLC [DAICEL CHIRALCEL OD-H, detection at 254 nm, 9:1 n-hexane/iPrOH, flow rate = 1.0 mL/min, tR = 11.0 min (minor), 13.2 min (major)].
NMR data are identical to literature data.2i
ACKNOWLEDGEMENTS
This work was financially supported by KAKENHI (No. 20229001 and 23590038). N. K. thanks the Japan Prize Foundation for supporting this research by their grants in 2012.
References
1. For recent reviews, see: (a) B. M. Trost, M. R. Machacek, and A. Aponick, Acc. Chem. Res., 2006, 39, 747; CrossRef (b) J. T. Mohr and B. M. Stoltz, Chem. Asian J., 2007, 2, 1476; CrossRef (c) Z. Lu and S. Ma, Angew. Chem. Int. Ed., 2008, 47, 258; CrossRef (d) M. Diéguez and O. Pàmies, Acc. Chem. Res., 2010, 43, 312. CrossRef
2. For recent reviews on Pd catalyzed allylic alkylations with allylic alcohols, see: (a) J. Muzart, Eur. J. Org. Chem., 2007, 3077; CrossRef (b) M. Kimura and Y. Tamaru, Mini-Rev. Org. Chem., 2009, 6, 392; CrossRef For selected examples of related studies, see: (c) F. Ozawa, H. Okamoto, S. Kawagishi, S. Yamamoto, T. Minami, and M. Yoshifuji, J. Am. Chem. Soc., 2002, 124, 10968; CrossRef (d) K. Manabe and S. Kobayashi, Org. Lett., 2003, 5, 3241; CrossRef (e) Y. Kayaki, T. Koda, and T. Ikariya, J. Org. Chem., 2004, 69, 2595; CrossRef (f) H. Kinoshita, H. Shinokubo, and K. Oshima, Org. Lett., 2004, 6, 4085; CrossRef (g) M. Kimura, M. Futamata, R. Mukai, and Y. Tamaru, J. Am. Chem. Soc., 2005, 127, 4592; CrossRef (h) I. Usui, S. Schmidt, M. Keller, and B. Breit, Org. Lett., 2008, 10, 1207; CrossRef (i) I. Usui, S. Schmidt, and B. Breit, Org. Lett., 2009, 11, 1453; CrossRef (j) R. Matsubara, K. Masuda, J. Nakano, and S. Kobayashi, Chem. Commun., 2010, 46, 8662; CrossRef (k) G. Jiang and B. List, Adv. Synth. Catal., 2011, 353, 1667; CrossRef (l) H. Hikawa and Y. Yokoyama, J. Org. Chem., 2011, 76, 8433; CrossRef (m) R. Ghosh and A. Sarkar, J. Org. Chem., 2011, 76, 8508; CrossRef (n) Y. Tao, B. Wang, J. Zhao, Y. Song, L. Qu, and J. Qu, J. Org. Chem., 2012, 77, 2942. CrossRef
3. For recent examples of allylic alkylations with allylic alcohols using other transition-metal catalysis, see: (a) M. Utsunomiya, Y. Miyamoto, J. Ipposhi, T. Ohshima, and K. Mashima, Org. Lett., 2007, 9, 3371; CrossRef (b) A. B. Zaitsev, S. Gruber, and P. S. Pregosin, Chem. Commun., 2007, 4692; CrossRef (c) A. B. Zaitsev, S. Gruber, P. A. Plüss, P. S. Pregosin, L. F. Veiros, and M. Wörle, J. Am. Chem. Soc., 2008, 130, 11604; CrossRef (d) T. Ohshima, Y. Miyamoto, J. Ipposhi, Y. Nakahara, M. Utsunomiya, and K. Mashima, J. Am. Chem. Soc., 2009, 131, 14317; CrossRef (e) T. Ohshima, Y. Nakahara, J. Ipposhi, Y. Miyamoto, and K. Mashima, Chem. Commun., 2011, 47, 8322. CrossRef
4. For reviews, see: (a) M. Bandini, Angew. Chem. Int. Ed., 2011, 50, 994; CrossRef (b) M. Bandini, G. Cera, and M. Chiarucci, Synthesis, 2012, 504. CrossRef
5. (a) Y. Yamashita, A. Gopalarathnam, and J. F. Hartwig, J. Am. Chem. Soc., 2007, 129, 7508; CrossRef (b) C. Defieber, M. A. Ariger, P. Moriel, and E. M. Carreira, Angew. Chem. Int. Ed., 2007, 46, 3139; CrossRef (c) M. Roggen and E. M. Carreira, Angew. Chem. Int. Ed., 2011, 50, 5568; CrossRef (d) M. Lafrance, M. Roggen, and E. M. Carreira, Angew. Chem. Int. Ed., 2012, 51, 3470. CrossRef
6. C. García-Yebra, J. P. Janssen, F. Rominger, and G. Helmchen, Organometallics, 2004, 23, 5459. CrossRef
7. B. M. Trost and J. Quancard, J. Am. Chem. Soc., 2006, 128, 6314. CrossRef
8. For intramolecular asymmetric allylations with allylic alcohols, see: (a) S. Tanaka, T. Seki, and M. Kitamura, Angew. Chem. Int. Ed., 2009, 48, 8948; CrossRef (b) M. Bandini and A. Eichholzer, Angew. Chem. Int. Ed., 2009, 48, 9533; CrossRef (c) H. Yamamoto, E. Ho, K. Namba, H. Imagawa, and M. Nishizawa, Chem. Eur. J., 2010, 16, 11271; CrossRef (d) M. Bandini, M. Monari, A. Romaniello, and M. Tragni, Chem. Eur. J., 2010, 16, 14272; CrossRef (e) K. Miyata, H. Kutsuna, S. Kawakami, and M. Kitamura, Angew. Chem. Int. Ed., 2011, 50, 4649; CrossRef (f) P. Mukherjee and R. A. Widenhoefer, Angew. Chem. Int. Ed., 2012, 51, 140. CrossRef
9. G. Jiang and B. List, Angew. Chem. Int. Ed., 2011, 50, 9471. CrossRef
10. For the direct use of ketones in Pd catalyzed asymmetric allylic alkylations with other allylic electrophiles, see: (a) X. Zhao, D. Liu, F. Xie, Y. Liu, and W. Zhang, Org. Biomol. Chem., 2011, 9, 1871; CrossRef (b) X. Zhao, D. Liu, H. Guo, Y. Liu, and W. Zhang, J. Am. Chem. Soc., 2011, 133, 19354. CrossRef
11. For catalytic asymmetric allylic alkylations with ketone enamines, see: (a) D. J. Weix and J. F. Hartwig, J. Am. Chem. Soc., 2007, 129, 7720; CrossRef (b) X. Zhao, D. Liu, F. Xie, and W. Zhang, Tetrahedron, 2009, 65, 512. CrossRef
12. For reviews on catalytic asymmetric allylic alkylations with ketone enolates, see: (a) M. Braun and T. Meier, Synlett, 2006, 661; CrossRef (b) M. Braun and T. Meier, Angew. Chem. Int. Ed., 2006, 45, 6952. CrossRef
13. For reviews on the combination of transition-metal catalysis and amine organocatalysis, see: (a) Z. Shao and H. Zhang, Chem. Soc. Rev., 2009, 38, 2745; CrossRef (b) A. E. Allen and D. W. C. MacMillan, Chem. Sci., 2012, 3, 633. CrossRef
14. Our group has reported various catalytic asymmetric reactions utilizing amide-based ligand, see: (a) A. Nojiri, N. Kumagai, and M. Shibasaki, J. Am. Chem. Soc., 2009, 131, 3779; CrossRef (b) T. Nitabaru, A. Nojiri, M. Kobayashi, N. Kumagai, and M. Shibasaki, J. Am. Chem. Soc., 2009, 131, 13860; CrossRef (c) T. Mashiko, N. Kumagai, and M. Shibasaki, J. Am. Chem. Soc., 2009, 131, 14990; CrossRef (d) A. Matsuzawa, T. Mashiko, N. Kumagai, and M. Shibasaki, Angew. Chem. Int. Ed., 2011, 50, 7616. CrossRef
15. The reactions using other Pd salts, Pd(OAc)2, Pd2(dba)3·CHCl3, Pd(PPh3)4, did not proceed at all.
16. 4’ was detected by 1H NMR analysis, while quantity of 4’ could not be estimated due to its volatility.
17. M. Murakata, M. Nakajima, and K. Koga, J. Chem. Soc., Chem. Commun., 1990, 1657. CrossRef
18. Z. Wu, G. S. Minhas, D. Wen, H. Jiang, K. Chen, P. Zimniak, and J. Zheng, J. Med. Chem., 2004, 47, 3282. CrossRef
19. D. Craig and N. K. Slavov, Chem. Commun., 2008, 6054. CrossRef
20. A. Bouziane, M. Hélou, B. Carboni, F. Carreaux, B. Demerseman, C. Bruneau, and J.-L. Renaud, Chem. Eur. J., 2008, 14, 5630. CrossRef
21. H. Y. Kwon, S. Y. Lee, B. Y. Lee, D. M. Shin, and Y. K. Chung, Dalton Trans., 2004, 921. CrossRef
22. (a) M. Hingst, M. Tepper, and O. Stelzer, Eur. J. Inorg. Chem., 1998, 73; CrossRef (b) J. P. Cahill, F. M. Bohnen, R. Goddard, C. Krüger, and P. J. Guiry, Tetrahedron: Asymmetry, 1998, 9, 3831. CrossRef
23. These proline derivatives can be prepared following the literature procedure from an inexpensive compound, see: J. Chiba, G. Takayama, T. Takashi, M. Yokoyama, A. Nakayama, J. J. Baldwin, E. McDonald, K. J. Moriarty, C. R. Sarko, K. W. Saionz, R. Swanson, Z. Hussain, A. Wong, and N. Machinaga, Bioorg. Med. Chem., 2006, 14, 2725. CrossRef
24. I. Held, E. Larionov, C. Bozler, F. Wagner, and H. Zipse, Synthesis, 2009, 2267. CrossRef