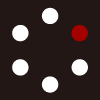
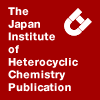
HETEROCYCLES
An International Journal for Reviews and Communications in Heterocyclic ChemistryWeb Edition ISSN: 1881-0942
Published online by The Japan Institute of Heterocyclic Chemistry
e-Journal
Full Text HTML
Received, 6th September, 2012, Accepted, 24th September, 2012, Published online, 5th October, 2012.
DOI: 10.3987/COM-12-S(N)120
■ CATALYTIC ASYMMETRIC INTERMOLECULAR C–H INSERTION OF 1,4-CYCLOHEXADIENE WITH α-ALKYL-α-DIAZOESTERS USING CHIRAL DIRHODIUM(II) CARBOXYLATES
Takayuki Goto, Tomohiro Onozuka, Yuhei Kosaka, Masahiro Anada, Koji Takeda, and Shunichi Hashimoto*
Laboratory of Synthetic and Industrial Chemistry, Graduate School of Life Science, Division of Life Science, Faculty of Pharmaceutical Sciences, Hokkaido University, Kita 12, Nishi 6, Kita-ku, Sapporo, Hokkaido 060-0812, Japan
Abstract
The first example of dirhodium(II) complex-catalyzed asymmetric intermolecular C–H insertion with α-alkyl-α-diazoesters is described. The reaction of 1,4-cyclohexadiene with 2,4-dimethyl-3-pentyl α-alkyl-α-diazoacetates under catalysis by dirhodium(II) tetrakis[N-phthaloyl-(S)-tert-leucinate], Rh2(S-PTTL)4, or dirhodium(II) tetrakis[N-tetrafluorophthaloyl-(S)-tert-leucinate], Rh2(S-TFPTTL)4, gave the corresponding C–H insertion products with enantioselectivities of up to 86% ee, albeit in low to modest yields.Catalytic functionalization of unactivated C–H bonds represents one of the most powerful strategies for C–C bond formation, and the development of asymmetric variants would be one of the ultimate goals in organic synthesis.1 In this context,†C–H insertion of diazo compounds catalyzed by chiral dirhodium(II) complexes has been recognized as an efficient means for enantioselective functionalization of C(sp3)–H bonds.2 In 1997, Davies and Hansen made a major breakthrough in this field when they achieved intermolecular C–H insertion reactions with high yield and excellent enantiocontrol.3a Donor/acceptor rhodium carbenes derived from the combination of aryldiazoacetates or arylvinyldiazoacetates with Rh2(S-DOSP)4 (2) as a chiral catalyst are capable of effective asymmetric C–H insertions with a diverse array of substrates, including alkanes (up to 96% ee),3a,c tetrahydrofuran (up to 60% de and 98% ee),3a,c N-Boc-protected amines (up to 94% de and 97% ee),3b allylic alkenes (up to 88% de and 95% ee),3c,e silyl ethers (up to >90% de and 95% ee),3d silyl enol ethers (up to >90% de and 97% ee),3f ethylbenzenes (up to 68% de and 94% ee),3g and N,N-dimethylanilines (up to 95% ee).3h The power of this strategy3–10 has been verified by the synthesis of a variety of natural products and pharmaceutical agents.4a–c,11–13 However, catalytic asymmetric intermolecular C–H insertion with α-alkyl-α-diazoesters14,15 remains a significant challenge because of the propensity to form α,β-unsaturated esters via a 1,2-hydride shift in metal-carbene intermediates.16 A notable exception is the recent work of Katsuki and Suematsu in which highly diastereo- and enantioselective C–H insertions with tert-butyl α-diazopropionate were achieved by using Ir(III)-salen complexes 4.7
The intermolecular C–H insertion reaction of 1,4-cyclohexadiene with diazocarbonyl compounds under the influence of chiral catalysts represents the bench mark reaction in this area.4–8,13,17 In 1999, Davies and co-workers were the first to demonstrate asymmetric induction (up to 95% ee) in the reaction with aryldiazoacetates using Rh2(S-DOSP)4 (2).4,5 Thereafter, Doyle and co-workers reported the reaction with vinyldiazolactone, in which Rh2(4S,R-MenthAZ)4 (3) provided the corresponding C–H insertion product in up to 80% ee.6 As touched on above, Katsuki and Suematsu recently disclosed that Ir(III)-salen catalyst 4a is highly exceptional for reactions with aryldiazoacetates (up to 99% ee) or tert-butyl α-diazopropionate (up to >99% ee).7 More recently, Che and co-workers explored the reaction with aryldiazoacetates in the presence of iridium(III)-porphyrin catalyst 5, in which high levels of asymmetric induction (up to 98% ee) were achieved.8
In recent years, we have achieved high levels of enantiocontrol in a range of catalytic metal carbene transformations of α-alkyl-α-diazoesters, including intramolecular C–H insertion,18 cyclopropenation,19 cyclopropanation,20 and C–H functionalization of indole,21 by using dirhodium(II) carboxylate catalysts, which incorporate N-phthaloyl- or N-tetrahalophthaloyl-(S)-amino acids as bridging ligands. In a continuation of our work, we addressed the issue of enantiocontrol in intermolecular C–H insertion reactions of 1,4-cyclohexadiene with α-alkyl-α-diazoesters.
At the outset, we explored the reaction of 2,4-dimethyl-3-pentyl α-diazopropionate (7a) with 4 equiv of 1,4-cyclohexadiene (6) in dichloromethane at room temperature using 1 mol % of Rh2(S-PTTL)4 (1a),15d,18,22 the most generally efficient catalyst of our dirhodium(II) carboxylate catalysts (Table 1, entry 1). The reaction proceeded to completion within 0.5 h and gave the C–H insertion product 8a in 61% yield with no signs of the formation of α,β-unsaturated ester 10a. In order to determine the sense and extent of asymmetric induction, 8a {[α]D23 +25.5 (c 1.36, CHCl3)} was converted by reduction with LiAlH4 and subsequent oxidation with DDQ into the known 2-phenyl-1-propanol (9a).23 The enantiomeric excess of 9a was determined to be 62% by HPLC using a Daicel Chiralcel OB-H column. The absolute stereochemistry of 9a was established as R by comparison of the sign of optical rotation {[α]D23 +9.1 (c 0.77, CHCl3) for 62% ee; lit.,23 [α]D23 +14.3 (c 1.65, CHCl3) for 95% ee of (R)-enantiomer}. The reaction with 10 equiv of 6
showed little variation in either yield or enantioselectivity (entry 2). Although Rh2(S-PTV)4 (1b) and Rh2(S-PTA)4 (1c) provided 8a in good yields with the same sense of asymmetric induction as that observed with 1a, poor enantioselectivities were obtained (25% and 3% ee, entries 3 and 4). We then evaluated the performance of Rh2(S-TFPTTL)4 (1d)24and Rh2(S-TCPTTL)4 (1e),25,26 fluorinated and chlorinated analogues of Rh2(S-PTTL)4 (1a). Catalysis with Rh2(S-TFPTTL)4 was found to provide 8a in 72% yield with 71% ee (entry 5), while Rh2(S-TCPTTL)4 displayed modest enantioselectivity (61% ee, entry 6).
Using Rh2(S-TFPTTL)4 (1d) as a catalyst, the effects of solvents on the enantioselectivity were examined (Table 2, entries 1–4). The use of α,α,α-trifluorotoluene, hexanes, and 2,2-dimethylbutane in place of CH2Cl2 produced good levels of asymmetric induction, in which 2,2-dimethylbutane exhibited the highest enantioselectivity (80% ee, entry 4). The effect of the ester moiety was also evaluated. Reactions with tert-butyl ester 7b and ethyl ester 7c resulted in only modest enantioselectivity (45% ee, entries 5 and 6). These results clearly show that the use of 2,4-dimethyl-3-pentyl ester moiety19,21,27 is crucial for a good degree of enantioselection. An examination of the temperature profile demonstrated that lowering the reaction temperature to 0 or −20 °C had only a marginal effect on the enantioselectivity (82% and 80% ee), though a sharp drop in product yield was observed (50% and 48%, entries 7 and 8).
We next turned our attention to C–H insertion with 2,4-dimethyl-3-pentyl α-diazobutanoate (7d) bearing more reactive β-C–H bonds than the methyl C–H bonds of α-diazopropionates (Table 3). The reaction with 7d using 1 mol % of Rh2(S-TFPTTL)4 (1d) or Rh2(S-PTTL)4 (1a) resulted in complete conversion within 0.5 h at room temperature, but formation of the desired C–H insertion product 8d was not observed by 1H-NMR analysis of the crude reaction mixture (entries 1 and 2). Instead (Z)-alkene 10d via a 1,2-hydride shift was isolated in 76% and 71% yields, respectively. It has been shown that low reaction temperatures are the key to suppression of the 1,2-hydride shift.15b–g,18 Thus, we conducted the reaction at −60 °C. Although Rh2(S-TFPTTL)4 produced almost none of 8d (entry 3), catalysis with Rh2(S-PTTL)4 furnished 8d with 83% ee, albeit in 12% yield (entry 4).28 These results are consistent with Taber’s speculation that a rhodium carbene intermediate bearing more electron-withdrawing ligands is more strongly positive at the carbene carbon and favors the less entropically demanding 1,2-hydride shift via an early transition state.15a,g,16b,d,19 Under the same conditions, the reaction with α-diazopentanoate 7e gave the C–H insertion product 8e in 20% yield with 86% ee, along with 22% of (Z)-alkene 10e (entry 5).29
In summary, we have reported that chiral dirhodium(II) carboxylate catalysts, Rh2(S-PTTL)4 and Rh2(S-TFPTTL)4, display a reasonable level of catalytic performance in intermolecular C–H insertion reactions of 1,4-cyclohexadiene (6) with 2,4-dimethyl-3-pentyl α-alkyl-α-diazoacetates 7. This represents the first example of intermolecular C–H insertion with α-alkyl-α-diazocarbonyl compounds bearing longer-chain alkyl groups than a methyl substituent, though there is considerable room for improvement in terms of chemo- and enantioselectivity as well as product yield. Efforts toward such improvement are currently underway.
EXPERIMENTAL
General. IR spectra were recorded on a JASCO FT/IR-5300 spectrometer and absorbance bands are reported in wavenumber (cm−1). 1H NMR spectra were recorded on a JEOL JNM-AL 400 (400 MHz) spectrometer. Chemical shifts are reported relative to an internal standard (tetramethylsilane at δH 0.00 or CDCl3 at δH 7.26). Data are presented as follows: chemical shift (δ, ppm), multiplicity (s = singlet, d = doublet, t = triplet, m = multiplet), coupling constant and integration. 13C NMR spectra were recorded on a JEOL JNM-AL 400 (100 MHz) spectrometer. Chemical shifts are reported relative to an internal standard (CDCl3 at δ 77.00). Optical rotations were measured on a JASCO P-1030 digital polarimeter at the sodium D line (589 nm). ESI-MS spectra were obtained on a JEOL JMS-T100LP or Thermo Scientific Exactive. Column chromatography was carried out on Kanto silica gel 60 N (63–210 mesh). Analytical thin layer chromatography (TLC) was carried out on Merck Kieselgel 60 F254 plates with visualization by ultraviolet, anisaldehyde stain solution or phosphomolybdic acid stain solution. Analytical high-performance liquid chromatography (HPLC) was performed on a JASCO PU-1580 intelligent HPLC pump with a JASCO UV-1575 intelligent UV/VIS detector. Detection was performed at 254 nm. Chiralpak AD-H, Chiralpak IA, or Chiralcel OB-H columns (0.46 cm × 25 cm) from Daicel were used. Retention times (tR) and peak ratios were determined with a JASCO-Borwin analysis system. All non-aqueous reactions were carried out in flame-dried glassware under an Ar atmosphere unless otherwise noted. Reagents and solvents were purified by standard means. Dehydrated CH2Cl2 was purchased from Kanto Chemical Co., Inc. 2,2-Dimethylbutane and α,α,α-trifluorotoluene were distilled from calcium hydride prior to use. 1,4-Cyclohexadiene (6) and hexanes were purchased from Aldrich, Inc. Chiral dirhodium(II) carboxylates 1a−e22c,24a,25a and α-alkyl-α-diazoesters 719 were prepared according to the literature procedures.
General procedure for enantioselective intermolecular C–H insertion (Table 1, entry 1): (R)-2,4-Dimethyl-3-pentyl 2-(2,5-cyclohexadienyl)propionate (8a). Rh2(S-PTTL)4·2AcOEt (1a) (7.1 mg, 0.005 mmol) was added in one portion to a solution of 6 (160.3 mg, 2.0 mmol) and 7a19 (99.1 mg, 0.50 mmol) in CH2Cl2 (1.5 mL) at room temperature. After stirring for 0.5 h at this temperature, the mixture was concentrated in vacuo. The ratio of 8a/10a was determined to be >99:1 by 1H NMR analysis of the crude reaction mixture. The crude product was purified by column chromatography (silica gel, 25:1 hexane/EtOAc) to provide 8a (76.5 mg, 61%) as a colorless oil; TLC Rf = 0.39 (10:1 hexane/EtOAc); [α]D23 +25.5 (c 1.36, CHCl3) for 62% ee; IR (neat) ν 2934, 2877, 1730, 1464, 1387, 1176 cm–1; 1H NMR (400 MHz, CDCl3) δ 0.86−0.90 (m, 12H, CH(CH3)2), 1.09 (d, J = 6.9 Hz, 3H, CHCH3), 1.87−1.94 (m, 2H, CH(CH3)2), 2.52−2.57 (m, 1H, CHCO), 2.64 (m, 2H, CH2CH=CH), 3.28 (m, 1H, CHCH=CH), 4.63 (t, J = 6.0 Hz, 1H, OCH), 5.56−5.64 (m, 2H, CH2CH=CH), 5.80−5.85 (m, 2H, CH2CH=CH); 13C NMR (100 MHz, CDCl3) δ 12.0 (CH3), 17.3 (CH3), 17.4 (CH3), 19.4 (CH3), 19.5 (CH3), 26.4 (CH2), 29.3 (CH), 29.4 (CH), 37.4 (CH), 52.5 (CH), 83.8 (CH), 127.7 (CH), 129.3 (CH), 133.9 (CH), 133.9 (CH), 175.9 (C=O); HRMS (EI) calcd for C16H27O2 (M + H)+ 251.2006, found 251.2005. The absolute configuration of 8a was determined to be R by chemical correlation (vide infra).
General procedure for reduction with LiAlH4 and oxidation with DDQ (Table 1, entry 1): (R)-2-Phenyl-1-propanol (9a). A solution of 8a (62.6 mg, 0.25 mmol) in THF (0.5 mL) was added dropwise to a stirred suspension of LiAlH4 (19.0 mg, 0.50 mmol) in THF (0.5 mL) at 0 °C. After stirring for 1 h, 1 M hydrochloric acid (1 mL) was added at 0 °C and the whole mixture was extracted with EtOAc (15 mL). The organic layer was washed with water (2 mL) and brine (2 mL) and then dried over anhydrous Na2SO4. Filtration and evaporation in vacuo furnished crude 2-(2,5-cyclohexadienyl)-1-propanol (35.1 mg) as a colorless oil, which was used without purification. 2,3-Dichloro-5,6-dicyano-1,4-benzoquinone (DDQ) (113.5 mg, 0.50 mmol) was added to a stirred solution of 2-(2,5-cyclohexadienyl)-1-propanol in CH2Cl2 (2 mL) at room temperature. After stirring for 1 h at this temperature, the reaction mixture was quenched with saturated aqueous Na2CO3 (2 mL). The mixture was extracted with EtOAc (15 mL). The organic layer was washed with water (2 mL) and brine (2 mL) and then dried over anhydrous Na2SO4. Filtration and evaporation in vacuo furnished the crude product (35 mg), which was purified by column chromatography on silica gel (7:3 hexane/Et2O) to afford 9a (28.3 mg, 83%) as a colorless oil; TLC Rf = 0.35 (3:1 hexane/EtOAc); [α]D23 +9.1 (c 0.77, CHCl3) [lit.,23 [α]D23 +14.3 (c 1.65, CHCl3) for 95% ee of (R)-enantiomer]; 1H NMR (400 MHz, CDCl3) δ 1.28 (d, J = 7.2 Hz, 3H, CHCH3), 2.94−3.00 (m, 1H, CHCH3), 3.71 (d, J = 6.8 Hz, 2H, CH2OH), 7.22−7.26 (m, 3H, Ar), 7.32−7.36 (m, 2H, Ar). The enantiomeric excess of 9a was determined to be 62% by HPLC with a Chiralpak OB-H column (100:1 hexane/iPrOH, 1.0 mL/min): tR (minor) = 16.5 min; tR (major) = 17.7 min.
(R)-tert-Butyl 2-(2,5-cyclohexadienyl)propionate (8b).7 According to the general procedure for intermolecular C–H insertion, 8b was prepared from 6 (160.3 mg, 2.0 mmol) and 7b (78.1 mg, 0.50 mmol) using Rh2(S-TFPTTL)4·2AcOEt (1d) (8.6 mg, 0.005 mmol). The crude product was purified by column chromatography (silica gel, 25:1 hexane/EtOAc) to provide 8b (57.3 mg, 55%) as a colorless oil; TLC Rf = 0.39 (10:1 hexane/EtOAc); [α]D25 +13.0 (c 1.41, CHCl3) for 45% ee [lit.,7 [α]D21 +29.1 (c 1.1, CHCl3) for >99% ee of (R)-enantiomer]; 1H NMR (400 MHz, CDCl3) δ 1.02 (d, J = 7.0 Hz, 3H, CHCH3), 1.45 (s, 9H, C(CH3)3), 2.33−2.44 (m, 1H, CHCO), 2.62 (m, 2H, CH2CH=CH), 3.19 (m, 1H, CHCH=CH), 5.49−5.59 (m, 2H, CH2CH=CH), 5.73−5.84 (m, 2H, CH2CH=CH). The enantiomeric excess of 8b was determined to be 45% after conversion to 9a.
(R)-Ethyl 2-(2,5-cyclohexadienyl)propionate (8c).7 According to the general procedure for intermolecular C–H insertion, 8c was prepared from 6 (160.3 mg, 2.0 mmol) and 7c (64.1 mg, 0.50 mmol) using Rh2(S-TFPTTL)4·2AcOEt (1d) (8.6 mg, 0.005 mmol). The crude product was purified by column chromatography (silica gel, 25:1 hexane/EtOAc) to provide 8c (65.9 mg, 73%) as a colorless oil; TLC Rf = 0.36 (10:1 hexane/EtOAc); [α]D25 +15.8 (c 1.28, CHCl3) for 45% ee [lit.,7 [α]D23 +27.4 (c 1.1, CHCl3) for 83% ee of (R)-enantiomer]; 1H NMR (400 MHz, CDCl3) δ 1.07 (d, J = 6.8 Hz, 3H, CHCH3), 1.26 (t, J = 6.9 Hz, 3H, OCH2CH3), 2.50 (m, 1H, CHCO), 2.66 (m, 2H, CH2CH=CH), 3.16−3.27 (m, 1H, CHCH=CH), 4.04−4.22 (m, 2H, OCH2CH3), 5.51−5.64 (m, 2H, CH2CH=CH), 5.77−5.88 (m, 2H, CH2CH=CH). The enantiomeric excess of 8c was determined to be 45% after conversion to 9a.
(R)-2,4-Dimethyl-3-pentyl 2-(2,5-cyclohexadienyl)butanoate (8d). According to the general procedure for intermolecular C–H insertion, 8d was prepared from 6 (320.1 mg, 4.0 mmol) and 7d19 (212.3 mg, 1.0 mmol) using Rh2(S-PTTL)4·2AcOEt (1a) (14.2 mg, 0.01 mmol). The crude product was purified by column chromatography (silica gel, 9:1 hexane/benzene) to give 8d (31.7 mg, 12%) as a colorless oil and 10d19 (47.9 mg, 26%) as a colorless oil; Data for 8d: TLC Rf = 0.38 (15:1 hexane/EtOAc); [α]D25 +23.3 (c 0.67, CHCl3) for 83% ee; IR (neat) ν 2965, 2876, 1730, 1464, 1388, 1177 cm–1; 1H NMR (400 MHz, CDCl3) δ 0.87 (d, J = 6.7 Hz, 6H, CH(CH3)2), 0.89 (d, J = 6.7 Hz, 6H, CH(CH3)2), 0.91 (t, J = 7.4 Hz, 3H, CH2CH3), 1.48 (m, 1H, CHCH2), 1.66 (m, 1H, CHCH2), 1.85-1.95 (m, 2H, CH(CH3)2), 2.35 (dt, J = 3.8, 10.5 Hz, 1H, CHCO), 2.64 (m, 2H, CH2CH=CH), 3.28 (m, 1H, CHCH=CH), 4.64 (t, J = 6.1 Hz, 1H, OCH), 5.59−5.67 (m, 2H, CH2CH=CH), 5.76−5.82 (m, 2H, CH2CH=CH); 13C NMR (100 MHz, CDCl3) δ 12.6 (CH3), 17.2 (CH3), 17.5 (CH3), 19.6 (CH3), 19.7 (CH3), 20.7 (CH2), 26.3 (CH2), 29.4 (CH), 29.4 (CH), 37.4 (CH), 52.5 (CH), 82.4 (CH), 125.7 (CH), 125.9 (CH), 125.9 (CH), 127.5 (CH), 174.4 (C=O); HRMS (ESI) calcd for C17H28O2Na (M+Na)+ 287.1987, found 287.1987. Data for 10d: TLC Rf = 0.41 (15:1 hexane/EtOAc); 1H NMR (400 MHz, CDCl3) δ 0.88 (d, J = 6.8 Hz, 6H, CH(CH3)2), 0.89 (d, J = 7.2 Hz, 6H, CH(CH3)2), 1.88–1.96 (m, 2H, CH(CH3)2), 2.14 (dd, J = 1.8, 7.2 Hz, 3H, CH3CH=CH), 4.65 (t, J = 6.3 Hz, 1H, OCH), 5.83 (dq, J = 1.8, 11.3 Hz, 1H, CH3CH=CH), 6.32 (dq, J = 7.2, 11.8 Hz, 1H, CH3CH=CH). The absolute configuration of 8d was determined to be R by chemical correlation (vide infra).
(R)-2-Phenyl-1-butanol.28 According to the general procedure for preparation of 9a, 2-phenyl-1-butanol was prepared from 8d (26.4 mg, 0.10 mmol). The crude product was purified by column chromatography on silica gel (7:3 hexane/Et2O) to afford 2-phenyl-1-butanol (11.9 mg, 79%) as a colorless oil; TLC Rf = 0.35 (3:1 hexane/EtOAc); [α]D23 –15.3 (c 0.35, CHCl3) [lit.,28 [α]D25 –21.1 (c 1.00, CHCl3) for (R)-enantiomer]; 1H NMR (400 MHz, CDCl3) δ 0.77 (t, J = 7.5 Hz, 3H, CH2CH3), 1.52 (m, 1H, CHCH2), 1.68 (m, 1H, CHCH2), 2.62 (m, 1H, CHCH2), 3.64−3.71 (m, 2H, CH2OH), 7.10−7.20 (m, 3H, Ar), 7.22–7.39 (m, 2H, Ar). The enantiomeric excess of 2-phenyl-1-butanol was determined to be 83% by HPLC with a Chiralpak AD-H column (300:1 hexane/iPrOH, 1.0 mL/min): tR (minor) = 54.6 min; tR (major) = 59.0 min.
(R)-2,4-Dimethyl-3-pentyl 2-(2,5-cyclohexadienyl)pentanoate (8e). According to the general procedure for intermolecular C–H insertion, 8e was prepared from 6 (320.6 mg, 4.0 mmol) and 7e19 (226.3 mg, 1.0 mmol) using Rh2(S-PTTL)4·2AcOEt (1a) (14.2 mg, 0.01 mmol). The crude product was purified by column chromatography (silica gel, 9:1 hexane/benzene) to provide 8e (55.7 mg, 20%) as a colorless oil and 10e19 (43.6 mg, 22%) as a colorless oil; Data for 8e: TLC Rf = 0.39 (15:1 hexane/EtOAc); [α]D23 +24.7 (c 1.10, CHCl3) for 86% ee; IR (neat) ν 2962, 2874, 1730, 1465, 1388, 1176 cm–1; 1H NMR (400 MHz, CDCl3) δ 0.87 (d, J = 6.7 Hz, 6H, CH(CH3)2), 0.88 (d, J = 6.7 Hz, 6H, CH(CH3)2), 0.90 (t, J = 7.4 Hz, 3H, CH2CH3), 1.19−1.41 (m, 3H, CH2), 1.60−1.69 (m, 1H, CHCH2), 1.85−1.95 (m, 2H, CH(CH3)2), 2.42−2.45 (m, 1H, CHCO), 2.63−2.65 (m, 2H, CH2CH=CH), 3.16−3.20 (m, 1H, CHCH=CH), 4.63 (t, J = 6.1 Hz, 1H, OCH), 5.58−5.67 (m, 2H, CH2CH=CH), 5.78−5.82 (m, 2H, CH2CH=CH); 13C NMR (100 MHz, CDCl3) δ 14.1 (CH3), 17.2 (CH3), 17.5 (CH3), 19.6 (CH3), 19.7 (CH3), 21.2 (CH2), 26.3 (CH2), 29.4 (CH2), 29.4 (CH), 29.6 (CH2), 37.5 (CH), 50.4 (CH), 82.4 (CH), 125.7 (CH), 126.0 (CH), 126.0 (CH), 127.5 (CH), 174.6 (C=O); HRMS (ESI) calcd for C18H30O2Na (M+Na)+ 301.2138, found 301.2136. Data for 10e: TLC Rf = 0.42 (15:1 hexane/EtOAc); 1H NMR (400 MHz, CDCl3) δ 0.87 (d, J = 6.8 Hz, 6H, CH(CH3)2), 0.89 (d, J = 6.8 Hz, 6H, CH(CH3)2), 1.07 (t, J = 7.7 Hz, 3H, CH2CH3), 1.87−1.96 (m, 2H, CH(CH3)2), 2.66 (dd, J = 7.7, 7.7 Hz, 2H, CH2CH3), 4.65 (t, J = 6.3 Hz, 1H, OCH), 5.78 (dt, J = 1.8, 11.3 Hz, 1H, CH2CH=CH), 6.21 (dt, J = 7.2, 11.3 Hz, 1H, CH2CH=CH). The absolute configuration of 8e was determined to be R by chemical correlation (vide infra).
(R)-2-Phenyl-1-pentanol.29 According to the general procedure for preparation of 9a, 2-phenyl-1-pentanol was prepared from 8e (50.1 mg, 0.18 mmol). The crude product was purified by column chromatography on silica gel (7:3 hexane/Et2O) to give 2-phenyl-1-pentanol (23.7 mg, 80%) as a colorless oil; TLC Rf = 0.35 (3:1 hexane/EtOAc); [α]D23 –9.9 (c 0.71, MeOH) for 86% ee [lit.,29 [α]D23 –12 (c 3.76, MeOH) for (R)-enantiomer]; 1H NMR (400 MHz, CDCl3) δ 0.86 (t, J = 7.2 Hz, 3H, CH2CH3), 1.15−1.26 (m, 2H, CH2CH3), 1.53−1.67 (m, 2H, CHCH2), 2.73 (m, 1H, CHCH2), 3.65−3.76 (m, 2H, CH2OH), 7.19−7.33 (m, 5H, Ar). The enantiomeric excess of 2-phenyl-1-pentanol was determined to be 86% by HPLC with a Chiralpak IA column (300:1 hexane/iPrOH, 1.0 mL/min): tR (minor) = 23.4 min; tR (major) = 26.6 min.
ACKNOWLEDGEMENTS
This research was supported, in part, by a Grant-in-Aid for Scientific Research from the Japan Society for the Promotion of Science and also by a Grant-in-Aid for Scientific Research on Innovative Areas "Organic Synthesis Based on Reaction Integration" (No. 2105) from the Ministry of Education, Culture, Sports, Science and Technology, Japan. We thank S. Oka and M. Kiuchi from the Center for Instrumental Analysis at Hokkaido University for technical assistance with mass spectrometry.
References
1. For a book and reviews, see: (a) 'Handbook of C–H Transformation,' Vols 1 and 2, ed. by G. Dyker, Wiley-VCH, Weinheim, 2005; CrossRef (b) K. Godula and D. Sames, Science, 2006, 312, 67; CrossRef (c) R. Giri, B.-F. Shi, K. M. Engle, N. Maugel, and J.-Q. Yu, Chem. Soc. Rev., 2009, 38, 3242; CrossRef (d) D. A. Colby, R. G. Bergman, and J. A. Ellman, Chem. Rev., 2010, 110, 624; CrossRef (e) J.-Q. Yu and Z. Shi, Top. Curr. Chem., 2010, 292, 1; CrossRef (f) T. Newhouse and P. S. Baran, Angew. Chem. Int. Ed., 2011, 50, 3362; CrossRef (g) J. Wencel-Delord, T. Dröge, F. Liu, and F. Glorius, Chem. Soc. Rev., 2011, 40, 4740; CrossRef (h) H. Lu and X. P. Zhang, Chem. Soc. Rev., 2011, 40, 1899; CrossRef (i) C.-M. Che, V. K.-Y. Lo, C.-Y. Zhou, and J.-S. Huang, Chem. Soc. Rev., 2011, 40, 1950; CrossRef (j) K. M. Engle, T.-S. Mei, M. Wasa, and J.-Q. Yu, Acc. Chem. Res., 2012, 45, 788; CrossRef (k) L. Yang and H. Huang, Catal. Sci. Technol., 2012, 2, 1099; CrossRef (l) J. Yamaguchi, A. D. Yamaguchi, and K. Itami, Angew. Chem. Int. Ed., 2012, 51, 8960. CrossRef
2. For books and reviews, see: (a) M. P. Doyle, M. A. McKervey, and T. Ye, 'Modern Catalytic Methods for Organic Synthesis with Diazo Compounds,' Wiley-Interscience, New York, 1998; (b) M. P. Doyle and D. C. Forbes, Chem. Rev., 1998, 98, 911; CrossRef (c) C. A. Merlic and A. L. Zechman, Synthesis, 2003, 1137; CrossRef (d) H. M. L. Davies and R. E. J. Beckwith, Chem. Rev., 2003, 103, 2861; CrossRef (e) P. M. P. Gois and C. A. M. Afonso, Eur. J. Org. Chem., 2004, 3773; CrossRef (f) Z. Zhang and J. Wang, Tetrahedron, 2008, 64, 6577; CrossRef (g) H. M. L. Davies and J. R. Manning, Nature, 2008, 451, 417; CrossRef (h) H. M. L. Davies and J. R. Denton, Chem. Soc. Rev., 2009, 38, 3061; CrossRef (i) H. M. L. Davies and J. Hansen, 'Catalytic Asymmetric Synthesis,' 3rd ed, ed. by I. Ojima, Wiley-VCH, New York, 2010, chap. 4; (j) C. N. Slattery, A. Ford, and A. R. Maguire, Tetrahedron, 2010, 66, 6681; CrossRef (k) M. P. Doyle, R. Duffy, M. Ratnikov, and L. Zhou, Chem. Rev., 2010, 110, 704; CrossRef (l) M. P. Doyle, M. Ratnikov, and Y. Liu, Org. Biomol. Chem., 2011, 9, 4007; CrossRef (m) H. M. L. Davies and D. Morton, Chem. Soc. Rev., 2011, 40, 1857. CrossRef
3. (a) H. M. L. Davies and T. Hansen, J. Am. Chem. Soc., 1997, 119, 9075; CrossRef (b) H. M. L. Davies, T. Hansen, D. W. Hopper, and S. A. Panaro, J. Am. Chem. Soc., 1999, 121, 6509; CrossRef (c) H. M. L. Davies, T. Hansen, and M. R. Churchill, J. Am. Chem. Soc., 2000, 122, 3063; CrossRef (d) H. M. L. Davies and E. G. Antoulinakis, Org. Lett., 2000, 2, 4153; CrossRef (e) H. M. L. Davies, P. Ren, and Q. Jin, Org. Lett., 2001, 3, 3587; CrossRef (f) H. M. L. Davies and P. Ren, J. Am. Chem. Soc., 2001, 123, 2070; CrossRef (g) H. M. L. Davies, Q. Jin, P. Ren, and A. Y. Kovalevsky, J. Org. Chem., 2002, 67, 4165; CrossRef (h) H. M. L. Davies and Q. Jin, Org. Lett., 2004, 6, 1769; CrossRef (i) H. M. L. Davies and Q. Jin, J. Am. Chem. Soc., 2004, 126, 10862. CrossRef
4. (a) H. M. L. Davies, D. G. Stafford, and T. Hansen, Org. Lett., 1999, 1, 233; CrossRef (b) H. M. L. Davies and T. M. Gregg, Tetrahedron Lett., 2002, 43, 4951; CrossRef (c) H. M. L. Davies, A. M. Walji, and R. J. Townsend, Tetrahedron Lett., 2002, 43, 4981; CrossRef (d) H. M. L. Davies and A. M. Walji, Org. Lett., 2003, 5, 479; CrossRef (e) H. M. L. Davies and J. Nikolai, Org. Biomol. Chem., 2005, 3, 4176; CrossRef (f) A. Ni, J. E. France, and H. M. L. Davies, J. Org. Chem., 2006, 71, 5594; CrossRef (g) P. Pelphrey, J. Hansen, and H. M. L. Davies, Chem. Sci., 2010, 1, 254. CrossRef
5. P. Müller and S. Tohill, Tetrahedron, 2000, 56, 1725. CrossRef
6. D. Bykowski, K.-H. Wu, and M. P. Doyle, J. Am. Chem. Soc., 2006, 128, 16038. CrossRef
7. H. Suematsu and T. Katsuki, J. Am. Chem. Soc., 2009, 131, 14218. CrossRef
8. J.-C. Wang, Z.-J. Xu, Z. Guo, Q.-H. Deng, C.-Y. Zhou, X.-L. Wan, and C.-M. Che, Chem. Commun., 2012, 48, 4299. CrossRef
9. Fokin and co-workers recently reported highly enantioselective intermolecular C–H insertion reactions of alkanes with 1-sulfonyl triazoles using Rh2(S-NTTL)4 or Rh2(S-PTAD)4 (up to 97% ee). S. Chuprakov, J. A. Malik, M. Zibinsky, and V. V. Fokin, J. Am. Chem. Soc., 2011, 133, 10352. CrossRef
10. For other examples of catalytic asymmetric intermolecular C–H insertions, see: (a) J. M. Fraile, J. I. García, J. A. Mayoral, and M. Roldán, Org. Lett., 2007, 9, 731; CrossRef (b) H.-Y. Thu, G. S.-M. Tong, J.-S. Huang, S. L.-F. Chan, Q.-H. Deng, and C.-M. Che, Angew. Chem. Int. Ed., 2008, 47, 9747; CrossRef (c) J. M. Fraile, P. López-Ram-de-Viu, J. A. Mayoral, M. Roldán, and J. Santafé-Valero, Org. Biomol. Chem., 2011, 9, 6075. CrossRef
11. J. M. Axten, R. Ivy, L. Krim, and J. D. Winkler, J. Am. Chem. Soc., 1999, 121, 6511. CrossRef
12. (a) H. M. L. Davies and Q. Jin, Tetrahedron: Asymmetry, 2003, 14, 941; CrossRef (b) H. M. L. Davies and A. Ni, Chem. Commun., 2006, 3110. CrossRef
13. For intermolecular C–H insertion of 1,4-cyclohexadiene using the combination of piperidinyl mandelate chiral auxiliary and Rh2(S-DOSP)4 (2) and it application to the stereocontrolled synthesis of kainoids. T. Higashi, Y. Isobe, H. Ouchi, H. Suzuki, Y. Okazaki, T. Asakawa, T. Furuta, T. Wakimoto, and T. Kan, Org. Lett., 2011, 13, 1089. CrossRef
14. (a) P. Panne and J. M. Fox, J. Am. Chem. Soc., 2007, 129, 22; CrossRef (b) P. Müller and C. Gränicher, Helv. Chim. Acta, 1995, 78, 129; CrossRef (c) A. Padwa, J. M. Kassir, and S. L. Xu, J. Org. Chem., 1997, 62, 1642. CrossRef
15. For other examples of dirhodium(II) complex-catalyzed intermolecular transformation of α-alkyl-α-diazoesters without alkene formation, see: (a) G. G. Cox, D. Haigh, R. M. Hindley, D. J. Miller, and C. J. Moody, Tetrahedron Lett., 1994, 35, 3139; CrossRef (b) A. DeAngelis, P. Panne, G. P. A. Yap, and J. M. Fox, J. Org. Chem., 2008, 73, 1435; CrossRef (c) P. Panne, A. DeAngelis, and J. M. Fox, Org. Lett., 2008, 10, 2987; CrossRef (d) A. DeAngelis, O. Dmitrenko, G. P. A. Yap, and J. M. Fox, J. Am. Chem. Soc., 2009, 131, 7230; CrossRef (e) A. DeAngelis, V. W. Shurtleff, O. Dmitrenko, and J. M. Fox, J. Am. Chem. Soc., 2011, 133, 1650; CrossRef (f) D. T. Boruta, O. Dmitrenko, G. P. A. Yap, and J. M. Fox, Chem. Sci., 2012, 3, 1589; CrossRef (g) A. DeAngelis, O. Dmitrenko, and J. M. Fox, J. Am. Chem. Soc., 2012, 134, 11035. CrossRef
16. (a) N. Ikota, N. Takamura, S. D. Young, and B. Ganem, Tetrahedron Lett., 1981, 22, 4163; CrossRef (b) D. F. Taber, M. J. Hennessy, and J. P. Louey, J. Org. Chem., 1992, 57, 436; CrossRef (c) D. F. Taber, R. J. Herr, S. K. Pack, and J. M. Geremia, J. Org. Chem., 1996, 61, 2908; CrossRef (d) D. F. Taber and P. V. Joshi, J. Org. Chem., 2004, 69, 4276. CrossRef
17. Hilt and co-workers reported intermolecular C–H insertion reactions of diazocarbonyl compounds with various polysubstituted and functionalized 1,4-dienes using Rh2(OAc)4. (a) G. Hilt and F. Galbiati, Org. Lett., 2006, 8, 2195; CrossRef (b) G. Hilt and F. Galbiati, Synthesis, 2006, 3589. CrossRef
18. K. Minami, H. Saito, H. Tsutsui, H. Nambu, M. Anada, and S. Hashimoto, Adv. Synth. Catal., 2005, 347, 1483. CrossRef
19. T. Goto, K. Takeda, N. Shimada, H. Nambu, M. Anada, M. Shiro, K. Ando, and S. Hashimoto, Angew. Chem. Int. Ed., 2011, 50, 6803. CrossRef
20. T. Goto, K. Takeda, M. Anada, K. Ando, and S. Hashimoto, Tetrahedron Lett., 2011, 52, 4200. CrossRef
21. T. Goto, Y. Natori, K. Takeda, H. Nambu, and S. Hashimoto, Tetrahedron: Asymmetry, 2011, 22, 907. CrossRef
22. (a) N. Watanabe, T. Ogawa, Y. Ohtake, S. Ikegami, and S. Hashimoto, Synlett, 1996, 85; CrossRef (b) H. Saito, H. Oishi, S. Kitagaki, S. Nakamura, M. Anada, and S. Hashimoto, Org. Lett., 2002, 4, 3887; CrossRef (c) H. Tsutsui, T. Abe, S. Nakamura, M. Anada, and S. Hashimoto, Chem. Pharm. Bull., 2005, 53, 1366; CrossRef (d) K. Takeda, T. Oohara, M. Anada, H. Nambu, and S. Hashimoto, Angew. Chem. Int. Ed., 2010, 49, 6979. CrossRef
23. C. Spino and C. Beaulieu, J. Am. Chem. Soc., 1998, 120, 11832. CrossRef
24. (a) H. Tsutsui, Y. Yamaguchi, S. Kitagaki, S. Nakamura, M. Anada, and S. Hashimoto, Tetrahedron: Asymmetry, 2003, 14, 817; CrossRef (b) M. Anada, M. Tanaka, T. Washio, M. Yamawaki, T. Abe, and S. Hashimoto, Org. Lett., 2007, 9, 4559; CrossRef (c) N. Shimada, S. Nakamura, M. Anada, M. Shiro, and S. Hashimoto, Chem. Lett., 2009, 38, 488. CrossRef
25. (a) M. Yamawaki, H. Tsutsui, S. Kitagaki, M. Anada, and S. Hashimoto, Tetrahedron Lett., 2002, 43, 9561; CrossRef (b) N. Shimada, M. Anada, S. Nakamura, H. Nambu, H. Tsutsui, and S. Hashimoto, Org. Lett., 2008, 10, 3603; CrossRef (c) N. Shimada, T. Oohara, J. Krishnamurthi, H. Nambu, and S. Hashimoto, Org. Lett., 2011, 13, 6284. CrossRef
26. (a) V. N. G. Lindsay, W. Lin, and A. B. Charette, J. Am. Chem. Soc., 2009, 131, 16383; CrossRef (b) V. N. G. Lindsay, C. Nicolas, and A. B. Charette, J. Am. Chem. Soc., 2011, 133, 8972; CrossRef (c) V. N. G. Lindsay and A. B. Charette, ACS Catal., 2012, 2, 1221.
27. (a) S. Hashimoto, N. Watanabe, T. Sato, M. Shiro, and S. Ikegami, Tetrahedron Lett., 1993, 34, 5109; CrossRef (b) S. Hashimoto, N. Watanabe, and S. Ikegami, Synlett, 1994, 353; CrossRef (c) S. Kitagaki, H. Matsuda, N. Watanabe, and S. Hashimoto, Synlett, 1997, 1171. CrossRef
28. The enantioselectivity was determined by HPLC after transformation of 8d to (R)-2-phenyl-1-butanol. C. H. Senanayake, R. D. Larsen, T. J. Bill, J. Liu, E. G. Corley, and P. J. Reider, Synlett, 1994, 199. CrossRef
29. The enantioselectivity was determined by HPLC after transformation of 8e to (R)-2-phenyl-1-pentanol. K. Kawazu, T. Fujita, and T. Mitsui, J. Am. Chem. Soc., 1959, 81, 932. CrossRef