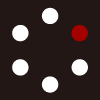
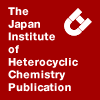
HETEROCYCLES
An International Journal for Reviews and Communications in Heterocyclic ChemistryWeb Edition ISSN: 1881-0942
Published online by The Japan Institute of Heterocyclic Chemistry
e-Journal
Full Text HTML
Received, 13th June, 2012, Accepted, 23rd July, 2012, Published online, 2nd August, 2012.
DOI: 10.3987/COM-12-S(N)32
■ Quantitative Analysis of Cu(I) Concentration in Click Chemistry — Biotinylation at Side Chain of Propargylglycine Using Click Chemistry under Heating Conditions —
Yui Ogasawara, Yuta Murai, Yasuko Sakihama, Yasuyuki Hashidoko, and Makoto Hashimoto*
Graduate School of Agriculture, Hokkaido University, Kita 9 Nishi 9, Kita-ku, Sapporo, Hokkaido 060-8589, Japan
Abstract
The click reaction is one of the latest techniques for the chemical modification of bioactive compounds. Chemical modifications of α-amino acid side chains are gaining significance as useful and important tools for biochemical research. The biotin-avidin specific interaction has been extensively utilized in functional analysis. Synthesis of side chain biotinylated α-amino acid derivative using click reaction and detail quantitative analysis of Cu(I) concentration in the course of the reactions are described.Click chemistry, which is 1,3-dipolar cycloaddition, is well known as a novel chemical modification method for bioactive compounds. The reaction proceeds between alkyne and azide in the presence of Cu(I) to make 1,2,3-triazole compounds.1 Chemically synthesized nonnatural α-amino acids play vital roles in functional analysis of not only α-amino acid but also peptide. Propargylglycine (3) is one of the most useful uncanonical α-amino acids, which is suitable as an alkyne donor for modification of side chain with click chemistry and the click product will be useful for analytical tools for the biomolecules recognized α-amino acid moiety. Unexpectedly, only a few papers have been reported using free propargylglycine to click chemistry.2 Polyethylene glycols are well known as hydrophilic spacer for maintaing hydrophilicity of the modified compounds. The spacer allows one to avoid undesirable hydrophobic interactions and ensures an optimal orientation of a ligand during the recognition process.3 Avidin-biotin specific interactions are one of the most useful tools for the biochemical analysis.4 The compound 1, side chain biotinylated α-amino acid derivative, will be useful to analyze α-amino acid recognized biomolecules (Scheme 1). In this paper, we report the synthesis of side chain biotinylated α-amino acid using propargylglycine (3) and azide derivative of biotinylated polyethylene glycol (Biotin-PEG-azide, 2) with click chemistry. Furthermore, time course quantitative analysis of the Cu(I) concentration was performed to proceed the click reaction.
Biotin-PEG-azide (2) was prepared with slight modifications reported by Vundyala et al.5 Equivalent amounts of biotin N-hydroxysuccinimide ester (4) and O-(2-aminoethyl)-O'-(2-azidoethyl)pentaethylene glycol (H2N-PEG-azide, 5) were reacted in TEA and DMF at rt in good yield (Scheme 2).
The biotin-PEG-azide (2) is subjected to click reaction with DL-propargylglycine (3). Neither Cu(I) generated system with Cu(II) salts (CuSO4 or Cu(OAc)2, 0.1 eq) and sodium ascorbate (0.2 eq) nor Cu(I) directly system with Cu(I) halides (CuI, CuBr and CuCl, 0.1 eq) afford click products at room temperature for 12 h. No improvements of the yields were observed by increasement of the copper catalysts and of reaction time. These results promoted us to investigate detail reactivities of both substrates (2 and 3) to overcome the results.
The biotin-PEG-azide (2) has already been subjected to click reaction with oligo(p-phenylene vinylene) in general manner without any difficulties.5 Boc-PEG-azide and biotin-PEG-azide (octaethyleneglycol) were applied to click reaction with 4-ethynyltoluene to make sure the reactivity of biotin and PEG moiety. The reaction smoothly proceeded in the presence of Cu(I) halides at rt for 12 h. The combination of Cu(II) salt and sodium ascorbate also applied in the same reaction conditions.
DL-Propargylglycine (3) was subjected to click reaction with benzyl azide (6). Neither Cu(I) halides nor Cu(II) salts-sodium ascorbate afforded the click reaction at room temperature. Although the Cu(II) salts-sodium ascorbate systems afforded the click product at 75 °C, Cu(I) halides did not promote the reaction at same temperature. The click product 7 was detected in the presence of Cu(I) halides and sodium ascorbate at 75 °C in good yield (Scheme 3). These results indicated that the free α-amino acid skeleton of 3 hampered the click reaction by decrement of Cu(I) concentration in the reaction mixture and high temperature (75 °C) required for optimal click reaction for 3.
Quantitative analysis of the Cu(I) concentration during the click reaction were investigated, because Cu(I) was sometimes mentioned about auto-oxidation to form Cu(II). To the best of our knowledge, there is no detail quantitative analysis for Cu(I) concentration during the click reactions. Bathocuproine disulphonic acid was one of the specific colorimetric reagents for the Cu(I) at 485 nm, with a ratio of 2:1 for Cu(I) and the reagent.6 The acid is also utilized as a chelator for Cu(I) in order to maintain the state of Cu(I) during the click reaction.7
To CuBr (0.1 eq) in the solvents, propargylglycine (3) and benzyl azide (6) were added in succession. A part of the reaction mixture for each addition was pipetted out, then subjected to colorimetric assay with bathocuproine disulphonic acid. In the click reaction for propargylglycine (3), Cu(I) concentration decreased for the mixture of CuBr and propargylglycine (3) in a time-dependent manner. The decrease was not reversed by the addition of benzyl azide (6) at either rt or 75 °C; furthermore, no triazole formation was observed under these conditions. However, Cu(I) concentration was maintained by the addition of sodium ascorbate (0.2 eq) to the reaction mixture at both rt and 75 °C (Scheme 4A). Although the starting materials were consumed and the triazole (7) was detected at 75 °C, no triazole formation was observed at room temperature. These results consisted with previous results that the effective click reaction for 3 required both high Cu(I) concentration and high temperature.
On the other hand, Cu(I) concentration of the mixture of CuBr and N-Boc-DL-propargylglycine (8) did not change over 4 h at room temperature with or without benzyl azide (6) (Scheme 4B). The click reaction of N-Boc-DL-propargylglycine (8) and benzyl azide (6) proceeded effectively at room temperature. No cyclization of N-Boc-DL-propargylglycine (8), which has been reported as a click side reaction under Cu(II) salt and sodium ascorbate,2b was observed under these conditions.It is reasonable to presume that free α-amino acid effected the Cu(I) concentration in the click reaction mixture by oxidation, and the Cu(I) concentration was recovered by the addition of sodium ascorbate as a reducing reagent.
The click reaction of N-Boc-DL-propargylglycine (8) and benzyl azide (6) at room temperature was carried out in the presence of glycine (10, 1 eq) as α-amino acid. It was found that Cu(I) concentration was decreased by addition of glycine and was not affected by the successive addition of N-Boc-DL-propargylglycine (8) and benzyl azide (6) to the mixture. The click reaction mixture with glycine caused the decrements of Cu(I) in a time-dependent manner and formed the triazole (9) in 60% yield. The formation of the triazole was considered that relatively higher Cu(I) concentration within an hour promoted the click reaction due to the compound N-Boc-DL-propargylglycine (8) and benzyl azide (6) can easily react at room temperature. There was not enough Cu(I) concentration for further click reaction over an hour. The click reaction mixture with glycine and sodium ascorbate maintained high Cu(I) concentration and formed the triazole at room temperature effectively within 2 h (Scheme 5). These results indicated that free α-amino acid inhibited click reaction due to decrement of Cu(I) concentration and sodium ascorbate prevented the decrement of Cu(I) concentration effectively.
According to the results of the experiments listed above, Bio-PEG-azide (2) was subjected to click reaction with DL-propargylglycine (3). The temperature-dependent manners of the click reaction with Biotin-PEG-azide (2) and DL-propargylglycine (3) in the presence of CuBr and sodium ascorbate were investigated. As same as previous results high temperature effectively promoted the click reaction (Scheme 6A). The reactivities of several catalysts were summarized in Scheme 6B. There was no marked difference between Cu(II) salts and Cu(I) halides in the presence of sodium ascorbate at 75 °C. All catalytic systems afforded the click product with excellent yields.
Although propargylglycine skeleton is one of the suitable for alkyne donor for click chemistry, only a few references have been reported using free propargylglycine.2 To the best of our knowledge, all references applied the Cu(II) salts-sodium ascorbate system to perform the click reaction for free propargylglycine. One of the references revealed that free propargylglycine could form the triazole at rt in analytical experiments, but N-Boc-DL-propargylglycine was used for click reaction at rt, followed by deprotection of Boc group to afford N-terminal free click products for preparative scale.2a The click reaction of DL-propargylglycine (3) with Cu(II) and sodium ascorbate systems carried out at high temperature in other references. Our results, in which the click reaction of DL-propargylglycine (3) with catalytic amounts of Cu(II) salt and sodium ascorbate depended on temperature-dependent manner, were consistent with these previous reports.
Our quantitative analysis revealed first time that the Cu(I) concentration was decreased by free α-amino acid when Cu(I) halides were used as Cu(I) source. The decrement of Cu(I) concentration was recovered by addition of sodium ascorbate effectively. Although recover the Cu(I) concentration in the reaction mixture is important but it was not enough to afford the click product. The heating process also needed to afford the click product effectively. Both these conditions are important for the click reaction for free DL-propargylglycine (3) in the presence of Cu(I) halides as catalyst. These results convinced us that there has been no report of the click condition with free propargylglycine and Cu(I) halides. It has been reported that the combination of CuI and histidine promoted a click reaction at room temperature.8 However, the authors used an equivalent of CuI for the click reaction, the condition was quite different from our systems in which 0.1 equivalent of Cu(I) halide is used. We have observed that Cu(I) concentration was enough to carry out the click reaction in their condition. It has been reported that Cu(0) generated Cu(I) in situ in the presence of amine hydrochloride to proceed the click reactions.9 The amino group of α-amino acids might have important role for the our observations. The click product (1) can be useful tool for the functional analysis of α-amino acid skeleton recognized biomolecules (eg α-amino acid transporter).
EXPERIMENTAL
General methods. 1H- and 13C- NMR spectra were measured by JEOL ECA 500 and EX270 spectrometers for structural determinations. In the 1H-NMR spectra, the chemical shifts are expressed in ppm downfield from the signal for tetramethylsilane used as an internal standard. In the 13C-NMR spectra, the 13C chemical shifts of the solvents were used as the internal standard (13CDCl3 77.0 ppm; or 13CD3OD, 49.5 ppm). ESI-MS spectra were obtained with a Waters LCT Premier XE spectrometer. DL-propargylglycine and H2N-PEG-azide were purchased from Aldrich. (+)-Biotin N-hydroxysuccinimide ester and bathocuproine disulphonic acid were purchased from Dojin. Cu(I) halides and Cu(II) salts are obtained from Wako.
Synthesis of biotin-PEG-azide (2)
(+)-Biotin N-hydroxysuccinimide ester (4, 0.0300 g, 0.088 mmol) and NH2-PEG-azide (5, 0.0310 g, 0.088 mmol) and TEA (0.0178 g, 0.176 mmol) were dissolved in DMF (1.2 mL). The reaction mixture was stirred at rt for 12 h and concentrated. The residue was subjected to silica gel chromatography (CHCl3 : MeOH =15 : 1) to afford product as colorless amorphous mass (0.0421 g, 83%). 1H-NMR (CDCl3) δ: 6.90 (1H, t, J = 5.4 Hz), 6.16 (1H, s), 5.21 (1H, s), 4.49 (1H, t, J = 6.0 Hz), 4.31 (1H, t, J = 6.0 Hz), 3.67-3.61 (22H, m), 3.55 (2H, t, J = 5.2 Hz), 3.44-3.40 (2H, m), 3.38 (2H, t, J = 4.6 Hz), 3.14 (1H, td, J = 6.0, 4.8 Hz), 2.90 (1H, dd, J = 13.2, 6.0Hz), 2.73 (1H, d, J = 13.2 Hz), 2.22 (2H, td, J = 7.4, 2.7 Hz), 1.79-1.61 (4H, m), 1.48-1.40 (2H, m); 13C-NMR (CDCl3) δ: 173.29, 163.82, 70.68-69.96 (12C), 61.72, 60.15, 55.53, 50.67, 40.55, 39.14, 35.90, 29.70, 28.11, 25.58; ESI-TOF-MS: [M+H]+ calculated for C24H45N6O8S, 577.3020, found 577.2990.
Synthesis of compound 9 (Click reaction of N-Boc-DL-propargylglycine (8) and benzyl azide (6))
Benzyl azide (6, 4.7 mg, 35.5 μmol) and N-Boc-DL-propargylglycine (8, 7.6 mg, 35.8 μmol) were dissolved in tert-BuOH - water (1:1; 126 μL) including CuBr (0.52 mg, 3.6 µmol). The reaction mixture was stirred at rt 4h, diluted with EtOAc and washed with brine. The aqueous solution was extracted with EtOAc. The combined organic extract was dried over MgSO4, filtrated and concentrated. The residue was subjected to silica gel chromatography (CH2Cl2 : MeOH = 4:1 then 2:1) to afford the product 9 as a pale yellow amorphous mass (10.7 mg, 87%). 1H-NMR (CD3OD) δ: 7.72 (1H, s), 7.39-7.29 (5H, m), 5.54 (2H, s), 4.23 (1H, s), 3.24 (1H, dd, J = 14.5, 4.6 Hz), 3.07 (1H, dd, J = 14.5, 7.3 Hz), 1.37 (9H, s); 13C-NMR (CD3OD) δ: 157.57, 136.77, 129.96, 129.49, 129.06, 125.04 (broad), 80.32, 79.49, 54.88, 29.79, 28.68; ESI-TOF-MS: [M+H]+ calculated for C17H23N4O4, 347.1719, found 347.1719.
Synthesis of compound 7 (Click reaction of DL-propargylglycine (3) and benzyl azide (6))
Benzyl azide (6, 4.7 mg, 35.5 μmol), DL-propargylglycine (3, 4.1 mg, 36.2 μmol), and sodium ascorbate (1.4 mg, 7.7 µmol) were dissolved in tert-BuOH : water (1 : 1; 126 μL) including CuBr (0.52 mg, 3.6 µmol). The reaction mixture was stirred at 75 ℃ for 4 h, filtrated and washed with water and ether, successively. The yield was determined by 1H-NMR and 13C-NMR. 1H-NMR (D2O containing 0.5% DCl) δ: 7.81(1H, s), 7.15-7.04 (5H, m), 5.34 (2H, s), 4.13 (1H, t, J = 5.9 Hz), 3.14 (2H, d, J = 6.3 Hz); 13C-NMR (D2O containing 0.5% DCl) δ: 170.82, 134.61, 129.76, 129.58, 128.90, 127.08 (broad), 55.29, 52.69, 25.75; ESI-TOF-MS: [M+H]+ calculated for C12H15N4O2, 247.1195, found 247.1189.
Synthesis of compound 1 (Click reaction of DL-propargylglycine (3) and biotin-PEG-azide (2))
Biotin-PEG-azide (2, 15.0 mg, 26 μmol) and DL-propargylglycine (3, 5.9 mg, 52 μmol) and sodium ascorbate (1.0 mg, 5.2 µmol) were suspended in DMF : H2O (1 : 7; 300 μL) including of CuBr (0.4 mg, 2.6 µmol). The reaction mixture was stirred for 5 h at 75 ℃ and concentrated. The residue was subjected to silica gel chromatography (H2O : MeOH = 9 : 1) to afford product as pale yellow amorphous mass (16.4 mg, 90%). 1H-NMR (D2O) δ: 7.79 (1H, s), 4.59 (1H, m), 4.47 (2H, m), 4.28 (1H, m), 3.91 (1H, t, J = 4.9 Hz), 3.83 (2H, t, J = 4.9 Hz), 3.57-3.49 (22H, m), 3.48 (2H, s), 3.24 (2H, t, J = 5.3 Hz), 3.19 (1H, m), 2.88 (1H, dd, J = 12.9, 4.9 Hz), 2.63 (1H, d, J = 12.9 Hz), 2.13 (2H, t, J = 7.1 Hz), 1.60-1.24 (6H, m); 13C-NMR (D2O) δ: 177.54, 176.63 (broad), 165.97, 125.65 (broad), 70.68-69.86 (12C), 69.57, 69.43, 62.76, 60.92, 56.05, 50.63, 40.40, 39.58, 36.14, 28.59, 28.39, 25.83; 1carboxy, 2triazole missing ESI-TOF-MS: [M+H]+ calculated for C29H52N7O10S, 690.3496, found 690.3517.
Cu(I) analysis in the click reaction with bathocuproine disulphonic acid
A fraction of the click reaction (9 μL) was pipetted out and added 17 mM bathocuproine disulphonic acid aqueous solution (15 μL) and sodium acetate solution (1.22 M, 840 μL). The assay mixture was adjusted to 1 mL with distilled water, then subjected to absorption measurement at 485 nm with spectrophotometer.
ACKNOWLEDGEMENTS
This research was partially supported by a Ministry of Education, Science, Sports and Culture Grant-in-Aid for Scientific Research (C), 19510210, 21510219.
References
1. H. C. Kolb, M. G. Finn, and K. B. Sharpless, Angew. Chem. Int. Ed., 2001, 40, 2004. CrossRef
2. a) T. L. Mindt, H. Struthers, L. Brans, T. Anguelov, C. Schweinsberg, V. Maes, D. Tourwe, and R. Schibli, J. Am. Chem. Soc., 2006, 128, 15096; CrossRef b) T. L. Mindt and R. Schibli, J. Org. Chem., 2007, 72, 10247; CrossRef c) T. L. Mindt, C. Müller, M. Melis, M. de Jong, and R. Schibli, Bioconjugate Chem., 2008, 19, 1689; CrossRef d) L. Zhou, A. Amer, M. Korn, R. Burda, J. Balzarini, E. De Clercq, E. R. Kern, and P. F. Torrence, Antivir. Chem. Chemother., 2005, 16, 375; e) P. Goyal, K. Yoon, and M. Weck, Chem. Eur. J., 2007, 13, 8801; CrossRef f) J. Xie and C. T. Seto, Bioorg. Med. Chem., 2007, 15, 458; CrossRef g) R. Duval, S. Kolb, E. Braud, D. Genest, and C. Garbay, J. Comb. Chem., 2009, 11, 947. CrossRef
3. Y. E. Tsvetkov, M. Burg-Roderfeld, G. Loers, A. Ardá, E. V. Sukhova, E. A. Khatuntseva, A. A. Grachev, A. O. Chizhov, H.-C. Siebert, M. Schachner, J. Jiménez-Barbero, and N. E. Nifantiev, J. Am. Chem. Soc., 2012, 134, 426. CrossRef
4. M. Wilchek and E. A. Bayer, Methods Enzymol., 1990, 184, 5. CrossRef
5. N. Vundyala, C. Sun, F. Sidime, W. Shi, W. L’Amoreaux, K. Raja, and R. M. Peetz, Tetrahedron Lett., 2008, 49, 6386. CrossRef
6. A. T. Faizullah and A. Townshend, Anal. Chim. Acta, 1985, 172, 291. CrossRef
7. a) W. G. Lewis, F. G. Magallon, V. V. Fokin, and M. G. Finn, J. Am. Chem. Soc., 2004, 126, 9152; CrossRef b) S. S. Gupta, J. Kuzelka, P. Singh, W. G. Lewis, M. Manchester, and M. G. Finn, Bioconjug. Chem., 2005, 16, 1572. CrossRef
8. K. Tanaka, C. Kageyama, and K. Fukase, Tetrahedron Lett., 2007, 48, 6475. CrossRef
9. H. A. Orgueira, D. Fokas, Y. Isome, P. C.-M. Chan, and C. M. Baldino, Tetrahedron Lett., 2005, 46, 2911. CrossRef