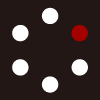
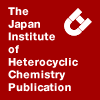
HETEROCYCLES
An International Journal for Reviews and Communications in Heterocyclic ChemistryWeb Edition ISSN: 1881-0942
Published online by The Japan Institute of Heterocyclic Chemistry
e-Journal
Full Text HTML
Received, 28th June, 2012, Accepted, 16th August, 2012, Published online, 21st August, 2012.
DOI: 10.3987/COM-12-S(N)65
■ Furosonin, a Novel Hydrolyzable Tannin from Geranium thunbergii
Shoko Taniguchi, Ryouta Nogaki, Li-Ming Bao, Teruo Kuroda, Hideyuki Ito, and Tsutomu Hatano*
Graduate School of Medicine, Dentistry and Pharmaceutical Sciences, Okayama University, 1-1-1 Tsushima-naka, Okayama 700-8530, Japan
Abstract
Furosonin (2), a novel hydrolyzable tannin, was isolated from Geranium thunbergii (Geraniaceae) leaves, and the structure was determined based on spectroscopic data. The effects of geraniin (1), furosonin (2), and related hydrolyzable tannins on antibiotic resistance were examined, and repandusinic acid A (4) was found to suppress oxacillin resistance of methicillin-resistant Staphylococcus aureus.Aboveground parts of Geranium thunbergii (Geraniaceae, “gen-no-shouko” or “fuu-ro-sou” in Japanese) have been used to treat diarrhea and constipation as a Pharmacopoeia medicine in Japan. This plant is known to be rich in tannin. The major constituent, geraniin (1),1 and structurally related hydrolyzable tannins, such as didehydrogerniin, furosinin, furosin,2 geraniinic acids B and C,3 and elaeocarpusin (ascorgeraniin),4,5 were isolated from the plant. We have isolated furosonin (2), a tannin from Geranium thunbergii with a novel acyl group. In this study, we discuss the isolation and structure of 2. Among the tannins structurally related to 1 and 2, corilagin (3)6 and repandusinic acid A (4)7 suppressed oxacillin resistance of methicillin-resistant Staphylococcus aureus (MRSA).
Dried leaves from G. thunbergii, cultivated in Okayama University Medicinal Botanical Garden, were homogenized in 70% acetone, and the concentrated filtrate from the homogenate was extracted with Et2O, EtOAc, and n-BuOH, successively. Although analysis of the EtOAc extract identified 1 as the major constituent, the n-BuOH extract contained unidentified compounds. The n-BuOH extract was thus chromatographed on Toyopearl HW-40C and MCI-gel CHP-20P. Further purification using high-performance liquid chromatography (HPLC) yielded 2, together with acalyphidin M1 (5).8
Furosonin (2) was obtained as a pale-yellow amorphous powder. High-resolution electrospray ionization mass spectrometry (HR-ESIMS) in negative-ion mode showed the [M-H]- ion at m/z 1083.1163, with the molecular formula C46H36O31 (calculated for C46H36O31-H, 1083.1168). The 1H NMR spectrum showed signals of a 2H singlet assignable to a galloyl group (δ 7.14), two 1H singlets from a hexahydroxydiphenoyl (HHDP) group (δ 6.60 and 7.02), and an additional aromatic singlet which was attributed to a penta-substituted benzene ring (δ 7.27) (unit D in formula 2) in the aromatic proton signal region. On the other hand, the spectrum showed signals ascribed to 1C4 glucopyranose core protons [δ 6.47 (br s, H-1), 5.54 (br s, H-2), 5.61 (br m, H-3), 5.28 (br m, H-4), 4.84 (t-like, J=10 Hz, H-5), 4.70 (dd, J=10, 11 Hz, H-6), and 4.38 (dd, J=8, 11 Hz, H-6)]. In the upper field region of the spectrum, methylene [δ 2.68 and δ 1.58 (each d, J=14 Hz, H-3 of unit E)] and methine [δ 4.68 (s), H-1 of unit E] protons with a long-range coupling were observed by 1H-1H correlation spectroscopy (COSY). In addition, the spectrum also showed signals of a methylene [δ 3.86 (dd, J=2.5, 9.5 Hz) and 4.12 (dd, J=5.5, 9.5 Hz) (H-1 of unit F)] – methine [δ 4.07 (ddd, J=1.5, 2.5, 5.5 Hz, H-2)] – methine [δ 4.15 (d, J=1.5 Hz, H-3)] system, as well as an isolated methine proton at δ 4.98 (s, H-5). These five protons, forming a pattern similar to that of corresponding protons in putranjivain A (6),9 were suggestive of a unit F structure, containing a five-membered ring derived from ascorbic acid. These D, E, and F units were assigned as a novel acyl group.
The presence of these constituent units was corroborated by the 13C NMR spectrum. Galloyl group signals were observed at δ 119.9 (C-1), 110.5 (C-2, C-6), 145.9 (C-3, C-5), 139.9 (C-4), and 165.2 (C-7). The presence of an HHDP group was shown by the seven pairs of signals at δ 115.3, 117.0 (C-1), 124.4, 125.4 (C-2), 107.6, 110.2 (C-3), 144.6, 145.1 (2 × C), 145.3 (C-4, C-6), 136.3, 137.6 (C-5), 166.3, and 168.6 (C-7). Glucose carbon signals were observed at δ 91.7 (C-1), 70.4 (C-2), 62.9 (C-3), 66.1 (C-4), 73.4 (C-5), and 64.0 (C-6), corresponding to its 1C4 conformation.10
Novel acyl group signals appeared as follows. Unit D signals were observed at δ 111.5 (C-1), 119.3 (C-2) (quaternary carbons), 114.5 (hydrogen-bearing C-3), 145.9 (C-4), 138.6 (C-5), 144.9 (C-6) (oxygen-bearing quaternary carbons), and 165.8 (ester carbonyl C-7), corresponding to the C-substituted galloyl structure. The downfield shift of the C-5 signal, relative to the corresponding carbon signals of HHDP (δ 136.3 and 137.6), was ascribed to the formation of the ether linkage at C-6. Unit F signals containing the furanose-like five-membered ring were observed at δ 75.3 (ether oxygen-bearing methylene C-1), 77.3 (C-2), 81.4 (C-3), 109.7 (hemi-ketal C-4), and 77.1 (C-5). Among them, C-2, C-3, and C-5 were oxygen-bearing methine carbons. The remaining signals attributed to unit E carbons were observed at δ 52.0 (C-1 binding to the phenyl of unit D), 53.2 (C-2 binding to CO), 32.4 (methylene C-3), 98.4 (C-4), 98.9 (C-5), 98.8 (C-6) (hemi-ketal or gem-diol carbons), and 170.6 (C-7) (ester carbonyl).
Assignments of the ester carbonyl groups of the acyl groups and their locations on the glucose core were attained based on the 1H-13C heteronuclear multiple-bond correlation spectroscopy (HMBC), as follows (Figure 3). The galloyl 2H singlet of H-2 and H-6 protons at δH 7.10 showed connectivity with glucose H-1 via the ester carbonyl signal at δC 165.2. The HHDP 1H singlets of H-3 protons at δH 7.00 and δH 6.60 were respectively connected with glucose H-3 and H-6 via ester carbonyl carbons at δC 166.3 and δC 168.6. The remaining glucose H-2 and H-4 were connected respectively with H-3 of unit D via an ester carbonyl carbon at δC 165.8 and with H-1 of unit E via an ester carbonyl carbon at δC 170.6.
Linkages between units D and E were also corroborated by the HMBC correlations. H-1 of unit E showed correlations with unit E (C-2, C-3, and C-6) and unit D carbons (C-1, C-2, and C-6), in addition to C-7 of unit E. Furthermore, H-3a of unit E correlated with C-5 of unit F, along with unit E carbons (C-1, C-2, and C-4). On the other hand, H-3b of unit E correlated with C-1, C-2, and C-5 of unit E, which supported assignments of the unit E structure. H-5 of unit F correlated with C-2, C-5, and C-7 of unit E, in addition to C-3 of unit F, which also satisfied the linkages between units E and F.
The ROESY spectrum showed a correlation between H-1 in unit E with glucose H-1, reflecting the spatial proximity between these two protons, which satisfied the orientation of the acyl group on O-2 – O-4 of the glucose core (Figure 4). The ROESY spectrum also showed a correlation in unit E H-1 and H-3a, which allowed us to discriminate between H-3a and H-3b of unit E. This correlation suggested that H-3a and H-3b were oriented towards units D and F, respectively. H-3b of unit E also showed an ROE correlation with H-1a of unit F, indicating that this H-1a proton was at the front side of the five-membered ring of unit F. In turn, H-1a of unit F showed an ROE correlation with H-3 (unit F).
H-1b – H-5 and H-2 – H-5 correlations were also observed among the unit F protons. Thus, the configurations on the carbons C-2 – C-5 of unit F were assigned as shown in the structural formulae.
A negative Cotton effect at 246 nm ([θ] -4.1 × 104) in the circular dichroism spectrum was indicative of an R-configuration of the biphenyl moiety of the B – C HHDP units.11 Structure 2 was thus assigned to furosonin.
MRSA, which often acquires multi-drug resistance, causes serious clinical problems in hospitals, and identifying compounds that suppress drug resistance is an important strategy for treatment of infectious diseases. Since various tannins and related polyphenols,12-15 including 3,7 are known to suppress β-lactam resistance, we examined the effects of 1, 2 and structurally related tannins on MRSA antibiotic resistance. Since 3 and 4 were easily obtained from 1, we also examined these compounds. Efflux pumps play an important role in multi-drug resistance, and therefore we examined the effects of tannins on norfloxacin.16,17
The results were summarized in Table 1. All tannins except for 2 decreased the MIC of oxacillin against the MRSA OM623 strain at 1/4 MIC concentrations. Compounds 3 and 4 decreased the MIC of oxacillin from 256 to 8 μg/mL (for 4) or <1 μg/mL (for 3). On the other hand, the tannins did not decrease the MIC of norfloxacin noticeably. Further studies are required to identify additional compounds that suppress MRSA drug resistance.
EXPERIMENTAL
Specific rotations were recorded on a JASCO DIP-1000 digital polarimeter. CD spectra were measured on a JASCO J-720W spectrophotometer. ESI-MS was recorded on a Bruker Daltonics MicrOTOF II instrument in negative ion mode. The 1H and 13C NMR spectra were recorded on a Varian INOVA AS600 spectrophotometer (600 MHz for 1H, and 150.8 MHz for 13C) at 300 K. Chemical shifts are given in δ (ppm) values relative to that of the solvent signal [acetone-d6 (δH 2.04; δC 29.8)] on the tetramethylsilane scale. MRSA strains used in this study were clinical isolates from Okayama University Hospital.
solation of 2: Dried Geranium thunbergii leaves (200 g), cultivated in Okayama University Medicinal Botanical Garden, were homogenized with 70% aq. acetone (700 mL × 3). The homogenate was filtered and the filtrate was concentrated to 600 mL and extracted with Et2O (600 mL × 3), EtOAc (600 mL × 3) and n-BuOH (600 mL × 3), successively. A part (3.6 g) of n-BuOH extract (13 g) was subjected to column chromatography over Toyopearl HW-40C with 70% aq. EtOH and the eluate was monitored by HPLC. Combined fractions 46-53 (134.4 mg), which showed HPLC peaks representing unidentified compounds, were purified by column chromatography on MCI-gel CHP-20P with aqueous MeOH. Combined fractions 46-61 (11.2 mg, eluted with 20% aq. MeOH) from the MCI-gel column were purified by preparative HPLC to yield 2 (3.6 mg) under the following conditions: Column, YMC-Pack ODS-A A-324 (YMC) column (10 i.d. × 300 mm); solvent, 0.01 M H3PO4, 0.01 M KH2 PO4, and MeOH (11:11:3; flow rate, 2 mL/min; 280 nm UV detection); column temperature, 40 °C. Fractions 10-20 (15.2 mg, eluted with 40% MeOH) from the MCI-gel chromatography were separated on a Sep-Pak (Plus) C18 cartridge to yield 5 (5.6 mg, eluted with 30% aq. MeOH). Similarly, the residual (9.4 g) n-BuOH extract was purified by column chromatography on Toyopearl HW-40, MCI-gel CHP-20P, and Sephadex LH-20, and the fraction containing 2 was purified by preparative HPLC to yield 2 (12.5 mg).
Compound 2: Pale-yellow amorphous powder, [α]D - 33.3 (c 1.0, MeOH). HR-ESI MS m/z: 1083.1163 [M - H]- (calculated for C46H35O31, 1083.1168). UV λmax (MeOH) nm (log ε): 220 (4.97), 279 (4.60). CD (MeOH) [θ] (nm): +3.5 × 104 (200, shortest wavelength measured), -2.8 ×104 (222), +1.4 × 104 (236), -4.1 × 104 (246), +1.0 × 104 (263), -4.1 × 104 (289).
Preparation of 3 and 4 from 1: Compound 1 (100 mg) was dissolved in phosphate buffer (pH 7.4, 50 mL), and the solution was maintained at 40 °C for 16 h. After acidifying the solution to end the reaction, the solution was extracted with ethyl acetate. The EtOAc extract was applied to column chromatography on Toyopearl HW-40C to yield 3 (9.3 mg), and the aqueous layer was subjected to an MCI-gel column chromatography to produce 3 (14.9 mg) and 4 (6.3 mg), which were identified based on their 1H NMR spectra.
Effects of tannins on MIC of oxacillin and norfloxacin: The MIC of antimicrobial agents were determined using the broth dilution method.15 Briefly, an inoculum of about 105 CFU in 100 µL of Mueller–Hinton broth (Difco) supplemented with 0.85% NaCl were incubated in 96-well microtiter plates at 35 °C for 24 h. The lowest concentration of each of the antibiotics or tannins where the visual turbidity was low after incubation was considered to be the MIC.
ACKNOWLEDGMENTS
The NMR instrument used in this study is the property of the SC-NMR Laboratory of Okayama University. The MS spectrometer is the property of The MS Laboratory of Faculty of Agriculture, Okayama University. This study was supported in part by Intractable Infectious Diseases Research Project Okayama (IIDTPO).
References
1. T. Okuda, T. Yoshida, and T. Hatano, J. Chem. Soc., Perkin Trans. 1, 1982, 9. CrossRef
2. K, Yazaki, T. Hatano, and T. Okuda, J. Chem. Soc., Perkin Trans. 1, 1989, 2289. CrossRef
3. H. Ito, T. Hatano, O. Namba, T. Shirono, T. Okuda, and T. Yoshida, Chem. Pharm. Bull., 1999, 47, 1148. CrossRef
4. G. Nonaka, S. Morimoto, and I. Nishioka, Chem. Pharm. Bull., 1986, 34, 941. CrossRef
5. T. Okuda, T. Yoshida, T. Hatano, Y. Ikeda, T. Shingu, and T. Inoue, Chem. Pharm. Bull., 1986, 34, 4075. CrossRef
6. M. Shimizu, S. Shiota, T. Mizushima, H. Ito, T. Hatano, T. Yoshida, and T. Tsuchiya, Antimicrob. Agents Chemother., 2001, 45, 3198. CrossRef
7. R. Saijo, G. Nonaka, and I. Nishioka, Chem. Pharm. Bull., 1989, 37, 2624. CrossRef
8. Y. Amakura, M. Miyake, H. Ito, S. Murakaku, S. Araki, Y. Itoh, C.-F. Lu, L. L. Yang, K.-Y. Yen, T. Okuda, and T. Yoshida, Phytochemistry, 1999, 50, 667. CrossRef
9. S. El-Mekkawy, M. R. Meselhy, I. T. Kusumoto, S. Kadota, M. Hattori, and T. Namba, Chem. Pharm. Bull., 1995, 43, 641. CrossRef
10. T. Hatano, T. Yoshida, T. Shingu, and T. Okuda, Chem. Pharm. Bull., 1988, 36, 3849. CrossRef
11. T. Okuda, T. Yoshida, T. Hatano, T. Koga, N. Toh, and K. Kuriyama, Tetrahedron Lett., 1982, 23, 3937. CrossRef
12. S. Shiota, M. Shimizu, T. Mizushima, H. Ito, T. Hatano, T. Yoshida, and T. Tsuchiya, Biol. Pharm. Bull., 1999, 22, 1388. CrossRef
13. S. Shiota, M. Shimizu, T. Mizusima, H. Ito, T. Hatano, T. Yoshida, and T. Tsuchiya, FEMS Microbiol. Lett., 2000, 185, 135. CrossRef
14. T. Hatano, M. Kusuda, M. Hori, S. Shiota, T. Tsuchiya, and T. Yoshida, Planta Med., 2003, 69, 984. CrossRef
15. M. Kusuda, K. Inada, T. Ogawa, T. Yoshida, S. Shiota, T. Tsuchiya, and T. Hatano, Biosci. Biotechnol. Biochem., 2006, 70, 1423. CrossRef
16. M. M. Kristiansen, C. Leandro, D. Ordway, M. Martins, M. Viveiros, T. Pacheco, J. Molnar, J. E. Kristiansen, and L. Amaral, In Vivo, 2006, 20, 361.
17. H. Nakaminami, N. Noguchi, and M. Sasatsu, Antimicrob. Agents Chemother., 2010, 54, 4107. CrossRef