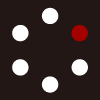
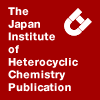
HETEROCYCLES
An International Journal for Reviews and Communications in Heterocyclic ChemistryWeb Edition ISSN: 1881-0942
Published online by The Japan Institute of Heterocyclic Chemistry
e-Journal
Full Text HTML
Received, 4th June, 2012, Accepted, 5th July, 2012, Published online, 13th July, 2012.
DOI: 10.3987/COM-12-S(N)26
■ Asymmetric Syntheses of Pyrrolidine and Piperidine Derivatives via Regio- and Stereo-Selective Ring-Opening Reactions of Chiral Aziridine
Kimio Higashiyama,* Masataka Matsumura, Emiko Kurita, and Takayasu Yamauchi
Institute of Medicinal Chemistry, Hoshi University, Ebara 2-4-41, Shinagawa-ku, Tokyo 142-8501, Japan
Abstract
Asymmetric synthesis of cyclic β-amino alcohols (pyrrolidine and piperidine derivatives) has been achieved using a single chiral aziridine as the starting material. The key step of this synthetic methodology is regio- and stereo-controlled ring-opening of the chiral aziridine with acetic acid or acetyl chloride.INTRODUCTION
Chiral aziridines are an attractive class of compounds because of their ring strain, which is the driving force of their reactivity.1 The regio- and stereo-controlled ring-opening of chiral aziridines with a wide variety of nucleophiles enables easy access to a large variety of compounds in optically active form.2 In a previous paper, we described the regio- and stereo-selective ring-opening reactions of 2-substituted chiral aziridines.3
These reactions are based on the fact that the position of an induced chiral center can be finely controlled, depending on the reagent used for the ring-opening reaction. The reaction of chiral 2-alkyl-substituted aziridines with acetic acid afforded β-amino alcohols with an (S)-chiral center at the β position (with respect to oxygen). In contrast, reaction of the same chiral aziridines with acetyl chloride followed by treatment with water gave β-amino alcohols with an (S)-chiral center at the α position.
To extend the scope of the synthetic applications of this reaction, we report herein the asymmetric synthesis of cyclic β-amino alcohols (pyrrolidine and piperidine derivatives) from a chiral aziridine. The key intermediate in the syntheses of the title compounds, the starting chiral aziridine, was prepared according to our previous report.4
RESULTS AND DISCUSSION
The condensation of (R)-O-benzylphenylglycinol (1)5 with 3-(1,3-dioxolan-2-yl)propanal in CH2Cl2 in the presence of 4Å molecular sieves gave the sensitive chiral imine 2, which, without purification, was treated with an excess of dimethylsulfonium methylide, prepared from trimethylsulfonium iodide with 1 equiv of n-butyllithium at 0 °C, to give the desired chiral aziridine 3 in 75.9% yield with high diastereomeric excess (92% de). The stereochemistry of the newly formed chiral center of the obtained aziridine is also presumed to have the S configuration, based on our previous report4 (Scheme 2).
With the requisite key chiral aziridine in hand, we synthesized the pyrrolidine derivative (prolinol), first using the regio- and stereo-controlled ring-opening reaction of aziridine 3 with acetic acid. The reaction proceeded smoothly at the reflux temperature of toluene by treating 3 with 1.5 equiv of acetic acid to afford the β-amino alcohol 4 in 76.7% isolated yield by C(3)–N bond cleavage of the aziridine ring. Since only 4 was obtained as a single compound, observed regioselectivity in this reaction was excellent. The cyclization of 4 with 10% aqueous hydrochloric acid followed by reduction with excess sodium cyanoborohydride afforded the pyrrolidine derivative 5 in quantitative yield. Finally, hydrogenolysis of the N-substituted benzyl group with palladium oxide as a catalyst gave the prolinol; its spectral and optical rotation data were consistent with those of commercially available (S)-prolinol. The synthesis of (S)-prolinol was thus accomplished in an overall yield of 52.8% from the chiral aziridine derivative 3.
The chiral aziridine 3 was also reacted with 1.5 equiv of acetyl chloride in THF at 0 °C followed by treatment with excess water. The β-amino alcohol 6, with an (S)-chiral center at the α position, was obtained by C(2)–N bond cleavage of the aziridine ring in 70.6% yield. The regioselectivity of this reaction was excellent, and the stereoselectivity of the ring-opened product 7 was extremely high since the signal of the diastereomer 6 was not detected in the 1H-NMR spectrum. Cyclization to form the piperidine ring was readily accomplished in the same manner as the synthesis of prolinol to give (S)-piperidin-3-ol. Our synthesis of (S)-piperidin-3-ol was accomplished in an overall yield of 43.0% from the chiral aziridine derivative 3; its spectral and optical rotation data were consistent with literature values6 (Scheme 3).
In addition, we achieved the asymmetric synthesis of (−)-pseudoconhydrine,7 which is one of the alkaloid constituents of the poison hemlock, from the same synthetic intermediate 6. After extensive screening of various reaction conditions, we found the optimal conditions for our synthesis of pseudoconhydrine, namely the β-amino alcohol 6 was treated with trimethylsilyl iodide in CH2Cl2 at room temperature to give the bicyclic compound 8. Although the 1H-NMR results for this product showed that it was a diastereomeric mixture (8:1) as a result of the newly formed chiral center at the 8a position, since this compound was unstable, the bicyclic compound 8 was used for the subsequent reactions without further purification.
The reaction of 8 with an excess of propylmagnesium bromide in THF at room temperature furnished the piperidine derivative 97as a separable diastereomeric mixture in a ratio of 7:1. After separation of the two isomers by silica-gel column chromatography, trans-9 was subjected to catalytic hydrogenation to provide (−)-pseudoconhydrine in 76.8% yield. The spectral and specific optical rotation data of the synthesized (−)-pseudoconhydrine were identical to those previously reported.8
CONCLUSION
The syntheses of compounds with different structures, such as pyrrolidine and piperidine derivatives, from the same aziridine derivative using regio- and stereo-selective ring-opening reactions with acetic acid or acetyl chloride were accomplished. In the synthesis of pseudoconhydrine, it was possible to induce chirality at the α position of the nitrogen as well as at the β position. These results show the usefulness of these reactions.
EXPERIMENTAL
Melting points were measured using a Yanagimoto micro melting point apparatus without collection. IR spectra were recorded on a JASCO FT/IR-200 spectrometer, and the major absorptions are listed (cm−1). 1H- and 13C-NMR spectra were obtained on a JEOL GSX 270 instrument, and the chemical sifts are reported in ppm on the δ scale from internal Me4Si. MS spectra were measured with a JEOL JMS 600 spectrometer using chemical ionization (CI) with isobutene and electron impact (EI) methods. Elemental analyses were performed on a Perkin-Elmer 240-B instrument. Optical rotations were obtained at room temperature using a JASCO-DIP-1000 polarimeter. A Sibata glass tube oven (GTO-350RD) was used as the distillation apparatus. Column chromatography was performed on silica gel (45–75 μm, Wakogel C-300). The reaction solvents were prepared as follows: THF was distilled over potassium metal; CH2Cl2 was distilled over phosphorus pentoxide.
(1’R,2S)-1-(2’-Benzyloxy-1’-phenylethyl)-2-(2-[1,3]dioxolan-2-ylethyl)aziridine (3)
A solution of n-butyllithium (14.4 mL, 1.6 M in hexane, 23.0 mmol) was added dropwise over several minutes to a stirred solution of powdered trimethylsulfonium iodide (4.70 g, 23.0 mmol) and HMPA (4.01 mL, 23.0 mmol) in 40 mL of dry THF under nitrogen at 0 °C. After stirring for 20 min, a solution of chiral imine 2, which was prepared from (R)-2-benzyloxy-1-phenylethylamine (1.75 g, 7.68 mmol) and 1,3-dioxolanyl-2-propranal (1.00 g, 7.68 mmol), in 5 mL of dry THF was added. After stirring for 30 min at 0 °C, the resulting mixture was diluted with 30 mL of water and extracted with Et2O (2 × 40 mL). The combined organic layers were washed with brine (2 × 30 mL), dried over anhydrous Na2SO4, and evaporated to give the crude product, which was purified using silica-gel flash chromatography with hexane/EtOAc (1:1) to afford the pure chiral aziridine (1’R,2S-3) and (1’R,2R-3) as a colorless viscous oil.
(1’R,2S)-3 (Major product): Yield 75.9%. IR (film): 2960 cm−1. EI-MS: m/z 232 (M+−CH2OCH2Ph). CI-MS: m/z 354 (M++1). 1H-NMR (CDCl3): δ 1.39–1.50 (5H, m), 1.71 (1H, d, J = 6.1 Hz), 1.79 (1H, d, J = 3.3 Hz), 2.64 (1H, dd, J = 7.0, 5.3 Hz), 3.67 (1H, dd, J = 9.7, 5.3 Hz), 3.73–3.87 (5H, m), 4.48 (1H, d, J = 12.2 Hz), 4.57 (1H, d, J = 12.2 Hz), 4.65 (1H, br), 7.22–7.40 (10H, m). 13C-NMR (67.8 MHz, CDCl3): δ = 140.86, 138.25, 128.10, 128.08, 127.64, 127.33, 127.24, 127.22, 103.81, 75.23, 74.05, 73.10, 64.55, 64.54, 36.51, 35.32, 31.20, 27.18. Anal. Calcd for C24H31NO5: C, 74.76; H, 7.70; N, 3.96. Found: C, 74.72; H, 7.67; N, 3.95. [α]D −7.10 (c 1.07, CHCl3).
(1’R,2R)-3 (Minor product): Yield 5.1%. IR (film): 2960 cm−1. EI-MS: m/z 232 (M+−CH2OCH2Ph). CI-MS: m/z 354 (M++1). 1H-NMR (CDCl3): δ 1.20 (1H, d, J = 6.3 Hz), 1.44 (1H, d, J = 3.5 Hz), 1.56–1.89 (5H, m), 2.66 (1H, d, J = 7.3 Hz), 3.65 (1H, dd, J = 5.1, 9.6 Hz), 3.74–3.97 (5H, m), 4.45 (1H, d, J = 12.0 Hz), 4.55 (1H, d, J = 12.0 Hz), 4.90 (1H, t, J = 4.8 Hz), 7.23–7.40 (10H, m). 13C-NMR (67.8 MHz, CDCl3): δ = 140.81, 138.16, 128.13, 128.04, 127.56, 127.45, 127.29, 127.16, 104.16, 75.63, 73.68, 73.12, 64.71, 64.70, 41.01, 31.52, 31.40, 27.59. Anal. Calcd for C24H31NO5: C, 74.76; H, 7.70; N, 3.96. Found: C, 74.67; H, 7.78; N, 3.96. [α]D −21.3 (c 1.10, CHCl3).
(1’R,2S)-2-(2’-Benzyloxy-1’-phenylethylamino)-4-[1,3]dioxolan-2-ylbutyl acetate (4)
A mixture of aziridine 3 (1.03 g, 2.91 mmol) and acetic acid (0.25 mL, 4.36 mmol) in toluene (20 mL) was stirred at reflux temperature for 5 h. After cooling, the reaction mixture was quenched with saturated aqueous Na2CO3 (10 mL). The organic layer was separated, and the aqueous layer was extracted with Et2O (10 mL × 2). The combined organic layers were washed with brine, dried over anhydrous Na2SO4, and the solvent was evaporated to give the crude product, which was purified using silica-gel flash chromatography with hexane/EtOAc (1:1) to afford pure product 4 as a yellowish viscous oil.
Yield 76.7%. IR (film): 3330, 1740, and 1240 cm−1. EI-MS: m/z 292 (M+−CH2OCH2Ph). CI-MS: m/z 413 (M++1). 1H-NMR (CDCl3): δ 1.45–1.66 (3H, m), 1.86–1.99 (1H, m), 1.96 (3H, s), 2.55–2.62 (1H, m), 3.41–3.53 (2H, m), 3.76–3.94 (5H, m), 4.11 (1H, dd, J = 4.2, 8.8 Hz), 4.22 (1H, dd, J = 4.4, 11.4 Hz), 4.51 (2H, s) 4.77 (1H, t, J = 4.5 Hz), 7.21–7.40 (10H, m). 13C-NMR (67.8 MHz, CDCl3): δ = 171.03, 140.69, 138.10, 128.27, 128.24, 127.75, 127.49, 127.43, 127.40, 104.41, 75.56, 72.80, 64.72, 64.71, 64.55, 59.29, 52.49, 30.37, 27.55, 20.70. Anal. Calcd for C24H31NO5: C, 69.71; H, 7.56; N, 3.39. Found: C, 69.71; H, 7.56; N, 3.39. [α]D −54.7 (c 1.02, CHCl3).
(1’R,2S)-[1-(2’-Benzyloxy-1’-phenylethyl)pyrrolidin-2-yl]methanol (5)
A solution of 4 (1.00 g, 2.42 mmol) in 10% aqueous solution of HCl (24 mL) was stirred at rt for 20 h. Then NaBH3CN [0.232 g (95%), 3.51 mmol] was added to the reaction mixture over a period of 5 min and stirred for 30 min at rt. After the reaction mixture had been made basic with a saturated aqueous solution of Na2CO3, the aqueous layer was extracted with CH2Cl2 (15 mL × 2). The combined organic layers were washed with brine, dried over anhydrous Na2SO4, and the solvent was evaporated to give the crude product, which was purified using silica-gel flash chromatography with hexane/EtOAc (1:1) to afford pure product 5 as a yellowish viscous oil.
Yield 92.9%. IR (film): 3460 cm−1. EI-MS: m/z 312 (M+). 1H-NMR (CDCl3): δ 1.49–1.77 (4H, m), 2.32 (1H, m), 2.86 (1H, m), 3.09 (2H, m), 3.41 (1H, dd, J = 3.5, 10.8 Hz), 3.67–3.76 (2H, m), 3.88 (1H, t, J = 8.4 Hz), 4.04 (1H, dd, J = 4.9, 8.4 Hz), 4.54 (1H, d, J = 12.4 Hz), 4.55 (1H, d, J = 12.4 Hz), 7.15–7.36 (10H, m). 13C-NMR (67.8 MHz, CDCl3): δ = 137.95, 137.80, 128.50, 128.19, 127.97, 127.39 (×2), 127.23, 72.96, 71.80, 63.45, 63.16, 60.25, 48.80, 28.14, 23.53. Anal. Calcd for C20H35NO2: C, 77.14; H, 8.09; N, 4.50. Found: C, 76.85; H, 8.18; N, 4.44. [α]D −50.2 (c 1.07, CHCl3).
(S)-Prolinol
A solution of 5 (0.313 g, 1.01 mmol) in MeOH (10 mL) was hydrogenated over 20% palladium hydroxide on carbon (30 mg) under H2 (3 atm) for 15 h at rt. The reaction mixture was then filtered through a small amount of Celite and the filtrate was evaporated under reduced pressure to give a residue, which was purified by bulb-to-bulb distillation (oven temperature 140 °C/7.0 mmHg) after washing with hexane to give (S)-prolinol as a yellowish viscous oil. Yield 74.1%. [α]D −30.1 (c 1.02, toluene). The spectral data and specific rotation were identical to those of commercially available (S)-prolinol.
(1S,1’R)-1-[(2’-Benzyloxy-1’-phenylethylamino)methyl]-3-[1,3]dioxolan-2-ylpropyl acetate (6)
To a stirred solution of aziridine 3 (1.04 g, 2.93 mmol) in anhydrous THF (20 mL) at 0 °C under a nitrogen atmosphere, acetyl chloride (0.345 g, 4.39 mmol) was added dropwise. After stirring the reaction mixture for 1 h at 0 °C, water (5 mL) was added and the mixture was stirred for 20 min at rt. The reaction mixture was made basic with saturated aqueous Na2CO3 (25 mL). The aqueous layer was extracted with Et2O (15 mL × 2). The combined extracts were washed with brine, dried over anhydrous Na2SO4, and the solvent was evaporated to give the crude product, which was purified using silica-gel flash chromatography with hexane/EtOAc (1:1) to afford pure product 6 as a yellowish viscous oil.
Yield 70.6%. IR (film): 3340, 3060, 1740, and 1240 cm−1. EI-MS: m/z 414 (M+). CI-MS: m/z 414 (M++1). 1H-NMR (CDCl3): δ 1.57–1.80 (4H, m), 2.00 (1H, br), 2.01 (3H, s), 2.52 (1H, dd, J = 4.8, 12.2 Hz), 2.63 (1H, dd, J = 7.3, 12.2 Hz), 3.45 (1H, t, J = 9.4 Hz), 3.52 (1H, dd, J = 3.9, 9.4 Hz), 3.78–3.97 (5H, m), 4.52 (1H, d, J = 11.9 Hz), 4.53 (1H, d, J = 11.9 Hz), 4.82 (1H, t, J = 4.1 Hz), 4.97 (1H, m), 7.22–7.37 (10H, m). 13C-NMR (67.8 MHz, CDCl3): δ = 170.56, 140.36, 137.96, 128.25 (×2), 127.51 (×2), 127.49, 127.38, 103.83, 75.34, 73.78, 73.00, 64.78 (×2), 62.87, 50.24, 29.36, 26.36, 20.97. Anal. Calcd for C24H31NO5: C, 69.71; H, 7.56; N, 3.39. Found: C, 69.58; H, 7.62; N, 3.37. [α]D –29.1 (c 1.07, CHCl3).
(1’R,3S)-1-(2’-Benzyloxy-1’-phenylethyl)piperidin-3-ol (7)
A solution of 6 (0.310 g, 0.750 mmol) in 10% aqueous solution of HCl (24 mL) was stirred at rt for 20 h. Then NaBH3CN [0.149 g (95%), 2.25 mmol] was added to the reaction mixture over a period of 5 min and stirred for 30 min at rt. After the reaction mixture had been made basic with a saturated aqueous solution of Na2CO3, the aqueous layer was extracted with CH2Cl2 (15 mL × 2). The combined organic layers were washed with brine, dried over anhydrous Na2SO4, and the solvent was evaporated to give the crude product, which was purified using silica-gel flash chromatography with hexane/EtOAc (1:1) to afford pure product 7 as a yellowish viscous oil.
Yield 86.8%. IR (film): 3410 cm−1. EI-MS: m/z 311 (M+). 1H-NMR (CDCl3): δ 1.40–1.52 (3H, m), 1.69–1.81 (1H, m), 2.27–2.35 (1H, m), 2.45–2.73 (3H, m), 3.00 (1H, br), 3.65–3.80 (3H, m), 3.85 (1H, dd, J = 5.7, 8.3 Hz), 4.52 (2H, s), 7.20–7.32 (10H, m). 13C-NMR (67.8 MHz, CDCl3): δ = 138.43, 137.93, 128.15 (×2), 127.95, 127.45, 127.39, 127.13, 72.95, 70.77, 68.33, 65.94, 56.80, 51.08, 31.71, 21.69. Anal. Calcd for C20H25NO2·1/10 water: C, 76.69; H, 8.11; N, 4.47. Found: C, 76.72; H, 8.24; N, 4.43. [α]D −33.5 (c 1.10, CHCl3).
(S)-Piperidin-3-ol
A solution of 7 (0.191 g, 0.613 mmol) in MeOH (10 mL) was hydrogenated over 20% palladium hydroxide on carbon (20 mg) under H2 (3 atm) for 15 h at room temperature. The reaction mixture was then filtered through a small amount of Celite, and the filtrate was evaporated under reduced pressure to give a residue, which was recrystallized from Et2O/hexane to afford (S)-piperidin-3-ol as colorless crystals.
Yield 70.2%. mp 58-60 °C. 1H-NMR (CDCl3): δ 1.39–1.61 (2H, m), 1.72–1.86 (2H, m), 2.08 (2H, s), 2.65–2.81 (3H, m), 2.96 (1H, dd, J = 2.6, 11.9 Hz), 3.69–3.76 (1H, m). [α]D −9.03 (c 2.02, MeOH); lit.6 [α]D24 −8.94 (c 4.7, MeOH). The spectral data and specific rotation were identical to those previously reported.
(3R,6S)-3-Phenylhexahydrooxazolo[3,2-a]pyridin-6-yl acetate (8)
To a solution of 6 (0.51 g, 1.23 mmol) in anhydrous CH2Cl2 (25 mL) at rt under a nitrogen atmosphere, iodotrimethylsilane (5.00 g, 25.0 mmol) was added dropwise. After stirring the reaction mixture for 20 h at rt, a saturated aqueous solution of Na2CO3 (20 mL) was added and the layers were separated. The organic layer was washed with brine, dried over anhydrous Na2SO4, and the solvent was evaporated to give the crude product, which was subjected to column chromatography on silica gel with hexane/EtOAc (1:2). The bicyclic compound 8 was obtained as a diastereomeric mixture (8:1), which was unstable and used without further purification.
Major component: 1H-NMR (CDCl3): δ 1.55–1.68 (1H, m), 1.82–2.09 (3H, m), 2.09 (3H, s), 2.27 (1H, dd, J = 2.1, 12.4 Hz), 3.01 (1H, dd, J = 2.3, 12.4 Hz), 3.60 (1H, t, J = 6.8 Hz), 3.66 (1H, dd, J = 6.7, 6.8 Hz), 3.84 (1H, dd, J = 3.5, 8.7 Hz), 4.19 (1H, t, J = 6.8 Hz), 4.91–4.94 (1H, m), 7.26–7.40 (5H, m).
Minor component: 1H-NMR (CDCl3): δ 1.43–2.10 (4H, m), 2.02 (3H, s), 2.58 (1H, dd, J = 12.0, 7.1 Hz), 2.94 (1H, dd, J = 12.0, 4.8 Hz), 3.89 (1H, dd, J = 4.8, 7.5 Hz), 4.26 (1H, dd, J = 4.8, 7.5 Hz), 4.38 (1H, t, J = 7.5 Hz), 4.47–4.50 (1H, m), 4.93–4.99 (1H, m), 7.26–7.40 (5H, m).
(1’R,3S,6S)-1-(2’-Hydroxy-1’-phenylethyl)-6-propylpiperidin-3-ol (9)
To a stirred solution of propylmagnesium bromide, prepared from 1-bromopropane (0.76 g, 6.2 mmol) and Mg (153.2 mg, 6.3 mmol), in dry THF (10 mL), a solution of 8 was added dropwise at 0 °C in dry THF (10 mL) under nitrogen over a 10-min period. After stirring the reaction mixture at rt for 20 h, it was quenched with water (0.5 mL) and filtered through a small amount of Celite. The filtrate was dried over anhydrous Na2SO4 and the solvent was evaporated to give the crude product as a diastereomeric mixture (7:1), which was subjected to column chromatography on silica gel with hexane/EtOAc (1:5) to afford pure (1’R,3S,6S)-trans-9 and (1’R,3S,6R)-cis-9 as a colorless viscous oil.
(1’R,3S,6S)-trans-9 (Major product): Yield 50.7%. IR (film): 3340 cm−1. EI-MS: m/z 263 (M+). 1H-NMR (CDCl3): δ 0.90 (3H, t, J = 7.1 Hz), 1.14–1.76 (8H, m), 2.02 (1H, m), 2.61 (1H, dd, J = 12.5, 3.2 Hz), 2.63 (2H, br), 2.66 (1H, dd, J = 12.5, 2.1 Hz), 2.94 (1H, m), 3.73 (1H, dd, J = 13.2, 8.4 Hz), 3.64–3.77 (2H, m), 7.23–7.36 (5H, m). 13C-NMR (67.8 MHz, CDCl3): δ = 140.44, 128.47, 128.28, 127.54, 67.24, 65.31, 62.48, 57.00, 48.97, 27.12, 26.96, 22.56, 20.14, 14.25. Anal. Calcd for C16H25NO2: C, 72.96; H, 9.57; N, 5.32. Found: C, 73.21; H, 9.71; N, 5.29. [α]D +28.9 (c 1.10, EtOH).
(1’R,3S,6R)-cis-9 (Minor product): Yield 7.2%. 1H-NMR (CDCl3): δ 0.95 (3H, t, J = 7.2 Hz), 1.26–1.75 (8H, m), 2.12 (1H, dd, J = 12.0, 2.0 Hz), 2.34 (2H, br), 2.39–2.47 (1H, m), 2.91 (1H, dd, J = 4.0, 12.0 Hz), 3.69 (1H, dd, J = 5.3, 10.0 Hz), 3.83 (1H, br), 3.97 (1H, t, J = 10.0 Hz), 4.31 (1H, dd, J = 5.3, 10.0 Hz), 7.19–7.38 (5H, m).
(3S,6S)-6-Propylpiperidin-3-ol (pseudoconhydrine)
A solution of trans-9 (0.26 g, 0.987 mmol) in MeOH (10 mL) was hydrogenated over 20% palladium hydroxide on carbon (20 mg) under H2 (3 atm) for 15 h at rt. The reaction mixture was then filtered through a small amount of Celite and the filtrate was evaporated under reduced pressure to give a residue, which was recrystallized from hexane to afford (3S,6S)-pseudoconhydrine as colorless crystals.
Yield 76.8%. mp 102-104 °C. 1H-NMR (CDCl3): δ 0.91 (3H, m), 1.06–1.39 (5H, m), 1.72–1.78 (4H, m), 2.02–2.17 (1H, m), 2.42 (1H, m), 2.46 (1H, dd, J = 10.0, 11.2 Hz), 3.23 (1H, ddd, J = 2.1, 4.6, 11.2 Hz), 3.64 (1H, m). [α]D +12.6 (c 1.01, EtOH); lit.,8 [α]D +10.3 (c 0.17, EtOH). The spectral data and specific rotation were identical to those previously reported.
ACKNOWLEDGMENTS
This work was supported in part by a Grant-in-Aid for Scientific Research from the Ministry of Education, Culture, Sports, Science and Technology of Japan, and by the Promotion and Mutual Aid Corporation for Private Schools of Japan.
References
1. For reviews, see: M. D’hooghe and N. De Kimpe, Tetrahedron, 2008, 64, 3275; CrossRef G. S. Singh, M. D’hooghe, and N. De Kimpe, Chem. Rev., 2007, 107, 2080; CrossRef X. E. Hu, Tetrahedron, 2004, 60, 2701; CrossRef J. B. Sweeney, Chem. Soc. Rev., 2002, 31, 247; CrossRef W. McCoull and F. Davis, Synthesis, 2000, 1347; CrossRef D. Tanner, Angew. Chem., Int. Ed. Engl., 1994, 33, 599. CrossRef
2. For reviews, see: S. Stankovic, M. D’hooghe, S. Catak, H. Eum, M. Waroquier, V. Van Speybroeck, N. De Kimpe, and H.-J. Ha, Chem. Soc. Rev., 2012, 41, 643; CrossRef for some recent examples, see: T. Katagiri, Y. Katayama, M. Taeda, T. Ohshima, N. Iguchi, and K. Uneyama, J. Org. Chem., 2011, 7, 9305; CrossRef M. A. Lowe, M. Ostovar, S. Ferrini, C. C. Chen, P. G. Lawrence, F. Fontana, A. A. Calabrese, and V. K. Aggarwal, Angew. Chem. Int. Ed., 2011, 50, 6370; CrossRef D. A. Ryan and D. Y. Gin, J. Am. Chem. Soc., 2008, 130, 15228; CrossRef H. Liu, V. R. Pattabiraman, and J. C. Vederas, Org. Lett., 2007, 9, 4211; CrossRef D. Savoia, G. Alvaro, R. D. Fabio, A. Gualandi, and C. Fiorelli, J. Org. Chem., 2006, 71, 9373; CrossRef M. K. Ghorai, K. Ghosh, and K. Das, Tetrahedron Lett., 2006, 47, 5399; CrossRef M. D’hooghe, A. Waterinckx, and N. De Kimpe, J. Org. Chem., 2005, 70, 227; CrossRef P. D. Pohlhaus, R. K. Bowman, and J. S. Johnson, J. Am. Chem. Soc., 2004, 126, 2294; CrossRef A. W. Miller and S. T. Nguyen, Org. Lett., 2004, 6, 2301; CrossRef A. B. Smith and D. S. Kim, Org. Lett., 2004, 6, 1493. CrossRef
3. K. Higashiyama, M. Matsumura, H. Kojima, and T. Yamauchi, Heterocycles, 2009, 78, 471. CrossRef
4. K. Higashiyama, M. Matsumura, A. Shiogama, T. Yamauchi, and S. Ohmiya, Heterocycles, 2002, 58, 85. CrossRef
5. A. I. Meyers, G. S. Poindexter, and Z. Brich, J. Org, Chem., 1978, 43, 892. CrossRef
6. N. Huh and C. M. Thompson, Tetrahedron, 1995, 51, 5935. CrossRef
7. A. Castro, J. Ramírez, J. Juárez, J. L. Terán, L. Orea, A. Galindo, and D. Gnecco, Heterocycles, 2007, 71, 2699; CrossRef H. Poerwono, K. Higashiyama, T. Yamauchi, H. Kubo, S. Ohmiya, and H. Takahashi, Tetrahedron, 1998, 54, 13955; CrossRef D. Enders and U. Reinhold, Tetrahedron: Asymmetry, 1997, 8, 1895. CrossRef
8. M. A. Wijdeven, F. L. van Delft, and F. P. J. T. Rutjes, Tetrahedron, 2010, 66, 5623. CrossRef