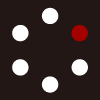
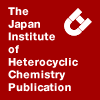
HETEROCYCLES
An International Journal for Reviews and Communications in Heterocyclic ChemistryWeb Edition ISSN: 1881-0942
Published online by The Japan Institute of Heterocyclic Chemistry
e-Journal
Full Text HTML
Received, 19th June, 2012, Accepted, 20th July, 2012, Published online, 24th July, 2012.
DOI: 10.3987/COM-12-S(N)43
■ Selective Deprotection of Methylene Acetal and MOM Ether in the Presence of Ketal-Type Protective Groups: Remarkable Effect of TBSOTf
Tomohiro Maegawa, Yasuyuki Koutani, Kento Senami, Kenzo Yahata, and Hiromichi Fujioka*
Graduate School of Pharmaceutical Science, Osaka University, 1-6 Yamadaoka, Suita, Osaka 560-0871, Japan
Abstract
A novel procedure for highly selective deprotection of methylene acetals and MOM (methoxymethyl) groups in the presence of ketal-type protective groups has been developed. The method, which utilizes a combination of TBSOTf and 2,2’-bipyridyl, displays a completely opposite selectivity compared with those of acid-mediated deprotection protocols.INTRODUCTION
In the area of organic synthesis, acetals and ketals are among the most widely employed protective groups for aldehydes and ketones.1 In addition, various acetal and ketal containing groups, such as MOM, BOM, acetonides and benzylidene acetals, have also been developed for protection of alcohols and diols. These protective groups are easily removed utilizing acidic conditions to produce the parent carbonyl and alcohol compounds. It is well-known that under these conditions ketals are deprotected more rapidly than acetals owing to the relative stabilities of the respective oxonium ion intermediates.2 The reactivities of acetal and ketal protective groups display the same trend, and methylene acetals are more robust diol protecting groups under acidic conditions than their counterparts. In fact, acetonides are preferentially cleaved under acidic conditions in the presence of methylene acetals3 (Scheme 1). In contrast, it is often difficult to cleave methylene acetals using mildly acidic conditions and, as a result, they are rarely used in organic synthesis because strong Lewis acids often need to be employed for their removal.1
In recent studies, we investigated the reactivity of stable cationic species, including pyridinium-type salts generated by the reaction of acetals with TESOTf and 2,4,6-collidine. These salts were found to readily react with various nucleophiles including water (Scheme 2, eq. 1).4
The results of this effort showed that the process, involving formation and hydrolysis of pyridinium salts, serves as a mild method for deprotection of acetal groups, THP and MOM ethers, as well as methylene acetals.5-7 In the cases of MOM and related ethers, the combination of TMSOTf (or TESOTf) and 2,2’-bipyridyl can be used for effective deprotection.6 In this process, 2,2’-bipyridylium salts are formed in situ and readily undergo hydrolysis to generate hemiacetals in routes to formation of parent alcohols (Scheme 2, eq. 2). The conditions employed are sufficiently mild to enable acid-labile groups, such as TBS and trityl (triphenylmethyl), to survive the reaction. It is noteworthy that, depending on the work-up conditions utilized to treat the intermediates, methylene acetals are also cleaved to afford the corresponding diols and their derivatives.7 For example, employment of acidic work-up (eg., 1N HCl) leads to formation of fully deprotected diols (Scheme 2, eq. 3), whereas basic work-up (satd. K2CO3 aq) can be employed to regioselectively generate monosilylated diols. Finally, methanolysis of cationic intermediates produces MOM protected diols in a regioselective manner.
The results of the studies described above indicate that TMSOTf (or TESOTf) would be capable of distinguishing between the different steric environments present in bis-acetals that contain the less crowded oxygen of methylene acetals. Initial work aimed at probing the selective deprotection process focused on the mixed ketal/acetal 1b, possessing a bulky benzophenone ketal and unhindered methylene acetal. Benzophenone ketals are known to be cleaved using weakly acidic conditions, such as AcOH or dilute HCl.1 In contrast, the results of this study showed that the methylene acetal moiety in 1b is selectively removed employing the TMSOTf based deprotection method (Scheme 3).7b
RESULTS AND DISCUSSION
In a more thorough investigation, summarized below, we explored the scope and limitations of the novel method for highly selective deprotection of acetals in the presence of ketals that takes advantage of the remarkable properties of TBSOTf. In the initial phase of this effort, we investigated reactions of 1c, which contains a methylene acetal and a cyclohexylidene ketal. An attempt to promote selective deprotection of 1c using TESOTf and 2,2’-bipyridyl followed by base treatment led to formation of 4a in a 28% yield. Moreover, enol ether 4a’ was produced as the major product (55%) in this process through β-elimination of the TESOTf activated cyclohexylidene acetal (Scheme 4).
This observation indicated that the steric bulkiness of TESOTf was insufficient to discriminate between the oxygens in the methylene acetal and the cyclohexylidene ketal moieties. Consequently, we next investigated the use of more bulky TBSOTf in this process and found that the selectively acetal-deprotected product 4b is generated exclusively in a 92% (Table 1, entry 1). In addition, the results showed that the methylene acetal group can be preferentially cleaved when other ketal protective groups are present. This selectivity is observed even in bis-ketal/acetals containing cyclopentylidene ketals, which are typically more highly susceptible to acid-catalyzed hydrolysis than cyclohexylidene ketals and acetonides8 (Table 1, entries 2 and 3). As expected, a contrastingly different reaction profile is observed when 1a is subjected to an acid-catalyzed deprotection reaction, which leads to formation of ketal deprotected product 2 (Scheme 1, Table 1, entry 4).
Additional studies showed that the TBSOTf based method can be employed in a diverse array of selective deprotection reactions of methylene acetals (Scheme 5). For example, basic work-up of the intermediate, 2,2’-bipyridilium salt, formed by treatment of 1a with the TBSOTf−2,2’-bipyridyl combination, affords the mono-TBS protected diol 4d in 95% yield. In addition, treatment of the salt with methanol and triethylamine results in regioselective generation of the MOM and TBS protected diol 5 in high yield (93%). Finally, conversions of the salt derived from 1a to diol 6 and mono-MOM protected diol 7 are achieved by using a one-pot protocol involving initial work-up with saturated K2CO3 aqueous solution and methanol, respectively, followed by treatment with TBAF/THF solution in one-pot.
Owing to the fact that MOM ethers also contain sterically unhindered acetal moieties, we presumed that they would be cleaved in preference to ketal protective groups. A method for selective deprotection of MOM ethers in the presence of acetonides and THP ethers, employing dimethylboron bromide and diphenylboron bromide at −78 ºC, has been described by Guindon and co-workers.9 However, these reagents are also capable of cleaving a variety of ethers and potentially can have effects on acid-sensitive functionality.
In studies probing the selective deprotection of MOM ethers 8a-c, which also contain ketals, we observed that the use of increased quantities of TBSOTf and 2,2’-bipyridyl are required (Table 2). Under these conditions, selective deprotection of the MOM ether moieties was found to take place in high yields without adverse effects on cyclohexylidene, cyclopentylidene and acetonide ketal groups (entries 1-3). In addition, more bulky sec- and tert-MOM ethers 8d and 8e undergo the selective deprotection process under the optimized conditions to give the corresponding alcohols 9d and 9e in 93% and 91% yields, respectively (entries 4 and 5). In these cases, acid-promoted hydrolysis results in preferential deprotection of the ketal protective groups, leaving the MOM ethers intact (Scheme 6).
A plausible mechanism for the selective deprotection process is depicted in Scheme 7. The initial and the most significant step in the pathway is Lewis acid coordination of TBSOTf to the acetal, which takes place in a highly discriminatory manner at the least sterically encumbered acetal. This conclusion is consistent with the observation that no selectivity is displayed in reactions promoted using the less sterically demanding TESOTf rather than TBSOTf. It is interesting to note that strict discrimination among four oxygen atoms is observed in these processes. Successive attack of 2,2’-bipyridyl on the complex then leads to formation of the salt intermediate in a chemo- and regioselective fashion. Finally, work-up with H2O affords the corresponding deprotected substances via hemi-acetal intermediate.
In conclusion, in the effort described above, we developed a novel, selective procedure for methylene acetal deprotection in substances that also contain ketal protective groups. This method displays a selectivity that is opposite to that of acid-mediated hydrolysis reactions because the reactivity differences arise from steric and not electronic effects. The new method is also applicable to MOM ether selective deprotection of substances in the presence of ketal-type protective groups. We believe that the current study has provided useful information about acetal deprotection reactions that will benefit those involved in the area of synthetic organic chemistry.
EXPERIMENTAL
General Information
Melting point (mp) was measured by Büchi B-545. Infrared spectra (IR) were recorded by Shimadzu FTIR 8400 using a diffuse reflectance measurement of samples dispersed in KBr powder. 1H NMR and 13C NMR spectra were recorded on a JEOL JNM-LA 500, JNM-ECS 400, JNM-AL 300 spectrometer in CDCl3 with tetramethylsilane as an internal standard. Data are reported as follows: chemical shift in ppm (δ), multiplicity (s = singlet, d = doublet, t = triplet, q = quartet, m = multiplet, brs = broad singlet), coupling constant (Hz) and integration. Mass spectra were obtained on a Shimadzu GCMS-QP 5000 instrument with ionization voltages of 70 eV. Column chromatography and TLC were carried out on Merck Silica gel 60 (230-400 mesh), Kanto Kagaku Silica gel 60N (40-50 µm, spherical, neutral), and Merck silica gel F254 plates (0.25 mm), respectively. The commercially available reagents were used without further purification. Compounds 9c9 is known compound.
Acidic hydrolysis of 1a (Scheme 1)
1a (39.5 mg, 0.145 mmol) was treated with CF3COOH (0.7 mL) and H2O (0.6 mL) for 1.5 h at room temperature. Then the mixture was extracted with CH2Cl2 (30 mL × 3) and the combined organic layers was dried over Na2SO4 and concentrated in vacuo. The residue was subjected to flash column chromatography (SiO2, hexanes/AcOEt = 10/1) affording 2 as colorless oil (29.1 mg, 87%).
9-(1,3-Dioxolan-4-yl)nonane-1,2-diol (2)
White solid (mp 39-42 °C); IR (KBr): 3395, 2932, 2361, 1466 cm-1; 1H NMR (400 MHz, CDCl3) δ: 1.21-1.41 (m, 13H), 1.53-1.68 (m, 1H), 2.01 (brs, 1H), 2.12 (brs, 1H), 3.35-3.45 (m, 2H), 3.59-3.74 (m, 2H), 3.89-4.02 (m, 2H), 4.84 (s, 1H), 4.99 (s, 1H); 13C NMR (100 MHz, CDCl3) δ: 25.5, 25.8, 29.43, 29.47, 29.5, 32.9, 33.1, 66.8, 69.6, 72.2, 76.2, 94.8; HRMS (FAB) calcd for C12H25O4 (M+H+) 233.1753, found 233.1743.
The Selective Deprotection of 1b (Scheme 3)
TMSOTf (73.1 μL, 0.404 mmol) was added to a solution of 1b (82.9 mg, 0.202 mmol) and 2,2’-bipyridyl (94.6 mg, 0.606 mmol) in CH2Cl2 (0.4 ml) at 0 °C under N2. The reaction mixture was stirred for 30 min at 0 °C. After disappearance of 1b on TLC, Et2O (2 ml) and 1N HCl (2 ml) was added to the reaction mixture. The resulting solution was stirred until disappearance of the pyridinium salt (highly polar compound). The mixture was extracted with CH2Cl2. The combined organic layer was dried over Na2SO4, filtered, and concentrated in vacuo. The residue was subjected to flash column chromatography (SiO2, hexanes/AcOEt = 1/1) to give a diol 3 (63.1 mg, 78%).
10-(2,2-Diphenyl-1,3-dioxolan-4-yl)decane-1,2-diol (3)
Colorless oil; IR (KBr): 3582, 2934, 2305 cm-1; 1H NMR (500 MHz, CDCl3) δ: 1.24-1.74 (m, 16H), 2.38 (brs, 2H), 3.39-3.42 (m, 1H), 3.61-3.69 (m, 3H), 4.08-4.16 (m, 2H), 7.24-7.34 (m, 6H), 7.48-7.52 (m, 4H); 13C NMR (125 MHz, CDCl3) δ:25.5, 25.7, 29.4, 29.47, 29.51, 33.1, 33.4, 66.8, 70.0, 72.3, 76.8, 109.3, 126.2, 127.8, 127.9, 128.1, 142.8; HRMS (EI) calcd for C25H34O4 (M+) 398.2457, found 398.2452.
The reaction of 1c with TESOTf and 2,2’-bipyridyl (Scheme 4)
TESOTf (94.5 μL, 0.42 mmol) was added dropwise to a solution of methylene acetal 1c (65.4 mg, 0.21 mmol) and 2,2′-bipyridyl (98.0 mg, 0.63 mmol) in CH2Cl2 (0.42 mL) at 0 ºC under N2. The reaction mixture was stirred at the same temperature. After checking the disappearance of 1c on TLC (1.5 h), satd. aq. K2CO3 (2 mL) and Et2O (2 mL) were added to the reaction mixture, which was then stirred vigorously. After disappearance of the high polar component, monitored by TLC, the mixture was extracted with CH2Cl (30 mL × 2). The combined organic layer was dried over Na2SO4, filtered, and concentrated in vacuo. The residue was subjected to flash column chromatography (SiO2, hexane/AcOEt = 8/1) to give 4a as yellow oil; 24.4 mg (28%) and 4a’ as colorless oil; 60.8 mg (55%).
9-(1,4-Dioxaspiro[4.5]decan-2-yl)-1-(triethylsilyloxy)nonan-2-ol (4a)
Colorless oil; IR (KBr): 2936, 2251, 1462 cm-1; 1H NMR (300 MHz, CDCl3) δ: 0.60 (q, J = 7.8 Hz, 6H), 0.94 (t, J = 7.8 Hz, 9H), 1.21-1.65 (m, 24H), 2.48 (brs, 1H), 3.30-3.37 (m, 1H), 3.44-3.49 (m, 1H), 3.57-3.66 (m, 2H), 3.97-4.06 (m, 2H); 13C NMR (100 MHz, CDCl3) δ: 4.3, 6.7, 23.9, 24.0, 25.2, 25.6, 25.7, 29.4, 29.58, 29.59, 32.7, 33.8, 35.3, 36.6, 66.9, 69.1, 71.8, 75.7, 109.1; HRMS (EI) calcd for C23H46O4Si (M+) 414.3165, found 414.3156.
11, 14-Bis(triethylsilyloxy)-13-(cyclohex-1’-enyloxy)-dodecan-2-ol (4a’)
Colorless oil, IR (KBr): 2936, 2251, 1664, 1456 cm-1; 1H NMR (500 MHz, CDCl3) δ: 0.53-0.63 (m, 12H), 0.89-0.97 (m, 18H), 1.23-1.65 (m, 18H), 1.97-2.04 (m, 4H), 2.44 (brs, 1H), 3.33-3.36 (m, 1H), 3.50-3.66 (m, 4H), 3.90-3.95 (m, 1H), 4.62-4.65 (m, 1H); 13C NMR (100 MHz, CDCl3) δ: 4.1, 4.3, 6.6, 6.7, 22.7, 23.0, 23.6, 25.4, 25.6, 28.1, 29.5, 29.7, 31.2, 32.7, 64.1, 66.9, 71.9, 75.8, 95.1, 153.3; HRMS (EI) calcd for C29H60O4Si2 (M+) 528.4030, found 528.4027.
Typical procedure for selective conversion of methylene acetals to mono-TBS protected diols 4 (Table 1). TBSOTf (91.9 μL, 0.40 mmol) was added dropwise to a solution of methylene acetal 1c (62.3 mg, 0.20 mmol) and 2,2′-bipyridyl (93.7 mg, 0.60 mmol) in CH2Cl2 (0.4 mL) at room temperature under N2. After observing the disappearance of 1c by using TLC (1.5 h), satd. aq. K2CO3 (2 mL) and Et2O (2 mL) were added to the mixture, which was then stirred vigorously. After disappearance of the high polar component, monitored by using TLC (8 h), the mixture was extracted with CH2Cl2 (20 mL × 3). The combined organic layer was dried over Na2SO4, filtered, and concentrated in vacuo. The residue was subjected to flash column chromatography (SiO2, hexanes/AcOEt = 5/1) to give the mono-TBS protected alcohol 4b (76.1 mg, 92%).
1-(tert-Butyldimethylsilyloxy)-9-(1,4-dioxaspiro[4.5]decan-2-yl)nonan-2-ol (4b): Colorless oil; IR (KBr): 2932, 1464 cm-1; 1H NMR (300 MHz, CDCl3) δ: 0.05 (s, 6H), 0.89 (s, 9H), 1.17-1.69 (m, 24H), 2.41 (brs, 1H), 3.32-3.39 (m, 1H), 3.44-3.49 (m, 1H), 3.57-3.61 (m, 2H), 3.99-4.06 (m, 2H); 13C NMR (100 MHz, CDCl3) δ: -5.39, -5.44, 18.2, 23.8, 24.0, 25.2, 25.5, 25.7, 25.8, 29.4, 29.5, 29.6, 32.7, 33.7, 35.3, 36.6, 67.2, 69.1, 71.8, 76.7, 109.0; HRMS (FAB) calcd for C23H47O4Si (M++H) 415.3244, found 415.3250.
1-(tert-Butyldimethylsilyloxy)-9-(1,4-dioxaspiro[4.4]nonan-2-yl)nonan-2-ol (4c): Colorless oil; IR (KBr): 3574, 2929, 2247, 1462 cm-1; 1H NMR (400 MHz, CDCl3) δ: 0.03 (s, 6H), 0.86 (s, 9H), 1.20-1.47 (m, 13H), 1.58-1.79 (m, 9H), 2.43 (brs, 1H), 3.32-3.37 (m, 1H), 3.42 (t, J = 6.8 Hz,1H), 3.56-3.62 (m, 2H), 3.92-3.99 (m, 2H); 13C NMR (100 MHz, CDCl3) δ: -5.45, -5.38, 18.2, 23.3, 23.6, 25.5, 25.7, 25.8, 29.4, 29.53, 29.56, 32.7, 33.6, 36.3, 36.7, 67.2, 69.2, 71.7, 75.9, 118.5; HRMS (EI) calcd for C22H44O4Si (M+) 400.3009, found 400.3011.
1-(tert-Butyldimethylsilyloxy)-9-(2,2-dimethyl-1,3-dioxolan-4-yl)nonan-2-ol (4d): Colorless oil; IR (KBr): 3572, 2930, 2249, 1464 cm-1; 1H NMR (500 MHz, CDCl3) δ: 0.05 (s, 6H), 0.88 (s, 9H), 1.23-1.66 (m, 20H), 2.40 (brs, 1H), 3.36 (m, 1H), 3.46-3.49 (m, 1H), 3.58-3.61 (m, 2H), 4.00-4.06 (m, 2H); 13C NMR (75 MHz, CDCl3) δ: -5.44, -5.37, 18.3, 25.5, 25.72, 25.83, 26.9, 29.38, 29.54, 29.56, 32.7, 33.5, 67.2, 69.5, 71.7, 76.1, 108.5; HRMS (FAB) calcd for C20H43O4Si (M+ +H) 375.2931, found 375.2948.
Typical procedure for the selective deprotection of methylene acetal 1a (Scheme 5)
Conversion of 1a to mono-TBS protected diol 4d. See the procedure for Table 1.
Conversion of 1a to MOM and TBS-protected diol 5. TBSOTf (114.0 μL, 0.49 mmol) was added dropwise to a solution of methylene acetal 1a (64.4 mg, 0.24 mmol) and 2,2′-bipyridyl (116.0 mg, 0.74 mmol) in CH2Cl2 (0.5 mL) at room temperature under N2. After observing the disappearance of 1a by using TLC (1.5 h), Et3N (98.0 μL, 0.71 mmol) and MeOH (2 mL) were added to the mixture, which was then stirred vigorously. After disappearance of the high polar component, monitored by using TLC (12 h), the mixture was extracted with AcOEt (20 mL × 3). The combined organic layers was washed with 3.5% aq. HCl (20 mL), H2O (20 mL) and satd. aq. NaHCO3 (20 mL). The organic layer was dried over Na2SO4, filtered and concentrated in vacuo. The residue was subjected to flash column chromatography (SiO2, hexanes/AcOEt = 10/1) affording product 5 (91.5 mg, 93%).
5-[7-(2,2-Dimethyl-1,3-dioxolan-4-yl)heptyl]-8,8,9,9-tetramethyl-2,4,7-trioxa-8-siladecane (5): Yellow oil; IR (KBr): 2929, 2249, 1464 cm-1; 1H NMR (400 MHz, CDCl3) δ: 0.02 (s, 6H), 0.85 (s, 9H), 1.19-1.64 (m, 20H), 3.34 (s, 3H), 3.45 (t, J = 6.8 Hz, 1H), 3.52-3.58 (m, 3H), 3.97-4.03 (m, 2H), 4.61 (d, J = 6.8 Hz, 1H), 4.74 (d, J = 6.8 Hz, 1H); 13C NMR (100 MHz, CDCl3) δ: -5.46, -5.42, 18.2, 25.3, 25.7, 25.9, 26.9, 29.4, 29.57, 29.59, 31.6, 33.5, 55.4, 65.8, 69.5, 76.1, 78.1, 96.2, 108.5; HRMS (FAB) calcd for C22H46O5SiNa (M++Na) 441.3012, found 441.3029.
Conversion of 1a to diol 6. TBSOTf (107.0 μL, 0.47 mmol) was added dropwise to a solution of methylene acetal 1a (63.5 mg, 0.23 mmol) and 2,2′-bipyridyl (109.0 mg, 0.70 mmol) in CH2Cl2 (1.2 mL) at room temperature under N2. After observing the disappearance of 1a on TLC (1 h), satd. aq. K2CO3 (0.5 mL) and Et2O (0.5 mL) were added to the mixture, which was then stirred vigorously. After disappearance of the high polar component, monitored by using TLC (17 h), TBAF (1.0 M THF solution, 1.16 mL, 1.16 mmol) was added to the mixture, which was then stirred for 9 h. The mixture was extracted with CH2Cl2 (20 mL × 3) and the combined organic layer was dried over Na2SO4, filtered, and concentrated in vacuo. The residue was subjected to flash column chromatography (SiO2, AcOEt only) affording product 6 (56.2 mg, 93%).
9-(2,2-Dimethyl-1,3-dioxolan-4-yl)nonane-1,2-diol (6): Colorless oil; IR (KBr): 3402, 2931, 2250, 1464 cm-1; 1H NMR (400 MHz, CDCl3) δ: 1.23-1.50 (m, 19H), 1.56-1.66 (m, 1H), 1.99 (brs, 2H), 3.39-3.49 (m, 2H), 3.62-3.70 (m, 2H), 3.99-4.07 (m, 2H); 13C NMR (75 MHz, CDCl3) δ: 25.5, 25.68, 25.71, 26.9, 29.3, 29.47, 29.51, 33.1, 33.5, 66.8, 69.5, 72.2, 76.1, 108.6; HRMS (FAB) calcd for C14H29O4 (M++H) 261.2066, found 261.2090.
Conversion of 1a to mono-MOM protected diol 7. TBSOTf (127.0 μL, 0.55 mmol) was added dropwise to a solution of methylene acetal 1a (73.9 mg, 0.27 mmol) and 2,2′-bipyridyl (130.0 mg, 0.83 mmol) in CH2Cl2 (0.55 mL) at room temperature under N2. After observing the disappearance of 1a by using TLC (1.5 h), MeOH (0.5 mL) and Et3N (112 μL, 0.81 mmol) were added to the mixture, which was then stirred vigorously. After disappearance of high polar component, monitored by using TLC (4 h), TBAF (1.0 M THF solution, 1.35 mL, 1.35 mmol) was added to the mixture, which was stirred for 24 h. The mixture was extracted with AcOEt (20 mL × 3) and the combined organic layers was washed with 3.5% aq. HCl (20 mL), H2O (20 mL) and satd. aq. NaHCO3 (20 mL). The organic layer was dried over Na2SO4, filtered, and concentrated in vacuo. The residue was subjected to flash column chromatography (SiO2, hexanes/AcOEt = 2/1) affording product 7 (58.5 mg, 71%).
9-(2,2-Dimethyl-1,3-dioxolan-4-yl)-2-(methoxymethoxy)nonan-1-ol (7): Colorless oil; IR (KBr): 3450, 2931, 2249, 1456 cm-1; 1H NMR (400 MHz, CDCl3) δ: 1.21-1.57 (m, 20H), 3.10 (brs, 1H), 3.39 (s, 3H), 3.42-3.54 (m, 4H), 3.97-4.04 (m, 2H), 4.64 (d, J = 6.8 Hz, 1H), 4.70 (d, J = 6.8 Hz, 1H); 13C NMR (100 MHz, CDCl3) δ: 25.4, 25.68, 25.69, 26.9, 29.3, 29.46, 29.51, 31.6, 33.5, 55.6, 65.6, 69.4, 76.1, 82.2, 96.9, 108.5; HRMS (FAB) calcd for C16H33O5 (M++H) 305.2328, found 305.2326.
Typical procedure for selective deprotection of MOM ether 8 (Table 2). TBSOTf (170.9 μL, 0.74 mmol) was added dropwise to a solution of MOM ether 8a (61.2 mg, 0.19 mmol) and 2,2′-bipyridyl (174.3 mg, 1.12 mmol) in CH2Cl2 (0.93 mL) at room temperature under N2. After observing the disappearance of 8a by using TLC (7 h), satd. aq. K2CO3 (2 mL) and Et2O (2 mL) were added to the mixture, which was then stirred vigorously. After disappearance of high polar component, monitored by using TLC (12 h), the mixture was extracted with AcOEt (20 mL × 3). The combined organic layer was washed with 3.5% aq. HCl (20 mL), H2O (20 mL) and satd. aq. NaHCO3 (20 mL). The organic layer was dried over Na2SO4, filtered, and concentrated in vacuo. The residue was subjected to flash column chromatography (SiO2, hexanes/AcOEt = 8/1) affording product 9a (52.1 mg, 98%).
9-(1,4-Dioxaspiro[4.5]decan-2-yl)nonan-1-ol (9a): Colorless oil; IR (KBr): 2934, 2253, 1464 cm-1; 1H NMR (400 MHz, CDCl3) δ: 1.23-1.65 (m, 26H), 3.45-3.49 (m, 1H), 3.62 (t, J = 6.6 Hz, 2H), 3.98-4.07 (m, 2H); 13C NMR (100 MHz, CDCl3) δ: 23.8, 23.9, 25.1, 25.7, 29.31, 29.35, 29.4, 29.5, 32.7, 33.7, 35.2, 36.5, 62.8, 69.0, 75.7, 109.0; HRMS (FAB) calcd for C17H33O3 (M++H) 285.2430, found 285.2430.
9-(1,4-Dioxaspiro[4.4]nonan-2-yl)nonan-1-ol (9b): Yellow oil; IR (KBr): 3404, 2929, 2250, 1466 cm-1; 1H NMR (400 MHz, CDCl3) δ: 1.27-1.80 (m, 24H), 3.43-3.46 (m, 1H), 3.62 (t, J = 6.8 Hz, 2H), 3.94-3.99 (m, 2H); 13C NMR (100 MHz, CDCl3) δ: 23.3, 23.5, 25.6, 29.3, 29.36, 29.41, 29.5, 32.7, 33.5, 36.3, 36.7, 62.9, 69.2, 75.9, 118.5; HRMS (EI) calcd for C16H30O3 (M+) 270.2195, found 270.2208.
9-(2,2-Dimethyl-1,3-dioxolan-4-yl)nonan-1-ol (9c)9: Colorless oil; 1H NMR (300 MHz, CDCl3) δ: 1.17-1.65 (m, 22H), 3.40-3.47 (m, 1H), 3.57 (t, J = 6.5 Hz, 2H), 3.94-4.05 (m, 2H).
10-(2,2-Dimethyl-1,3-dioxolan-4-yl)decan-2-ol (9d): Colorless oil; IR (KBr): 3616, 3465, 3155, 2931, 2250, 1456 cm-1; 1H NMR (500 MHz, CDCl3) δ: 1.15-1.49 (m, 23H), 1.59-1.65 (m, 2H), 3.46-3.49 (m, 1H), 3.74-3.77 (m, 1H), 3.99-4.07 (m, 2H); 13C NMR (125 MHz, CDCl3) δ: 23.4, 25.73, 29.75, 26.9, 29.41, 29.47, 29.58, 29.61, 33.5, 39.3, 68.1, 69.5, 76.2, 108.6; HRMS (FAB) calcd for C15H31O3 (M++H) 259.2273, found 259.2258.
10-(2,2-Dimethyl-1,3-dioxolan-4-yl)-2-methyldecan-2-ol (9e): Colorless oil; IR (KBr): 3417, 2931, 2249, 1714, 1456 cm-1; 1H NMR (400 MHz, CDCl3) δ: 1.11-1.68 (m, 28H), 3.43-3.48 (m, 1H), 3.97-4.06 (m, 2H); 13C NMR (75 MHz, CDCl3) δ: 24.3, 26.0, 26.9, 29.1, 29.41, 29.45, 29.47, 29.55, 29.60, 30.1, 33.5, 43.9, 69.5, 71.0, 76.1, 108.5; HRMS (FAB) calcd for C16H32O3Na (M++Na) 295.2249, found 295.2250.
Acidic hydrolysis of 8a (Scheme 6)
8a (39.7 mg, 0.121 mmol) was treated with AcOH (1.2 mL) and H2O (0.3 mL) at 50 °C and stirred for 3 h. Satd. aq. NaHCO3 was added to the reaction mixture and the mixture was extracted with AcOEt (30 mL × 3). The combined organic layer was dried over Na2SO4, filtered and concentrated in vacuo. The residue was subjected to flash column chromatography (SiO2, hexanes/AcOEt = 10/1) affording 10 (21.9 mg, 73%).
11-(Methoxymethoxy)undecane-1,2-diol (10): white solid (mp 40-41 °C); IR (KBr): 3366, 2929, 2251, 1465 cm-1; 1H NMR (400 MHz, CDCl3) δ: 1.26-1.40 (m, 14H), 1.52-1.59 (m, 2H), 2.20 (t, J = 5.6 Hz, 1H), 2.28 (t, J = 4.0 Hz, 1H), 3.33 (s, 3H), 3.37-3.42 (m, 1H), 3.49 (t, J = 6.8 Hz, 2H), 3.60-3.68 (m, 2H), 4.59 (s, 2H); 13C NMR (100 MHz, CDCl3) δ: 25.5, 26.1, 29.42, 29.45, 29.5, 29.6, 29.7, 33.1, 55.1, 66.8, 67.8, 72.3, 96.3; HRMS (FAB) calcd for C13H29O4 (M+H+) 249.2066, found 249.2061.
ACKNOWLEDGEMENTS
This work was financially supported by the Hoansha Foundation, the Uehara Memorial Foundation, and Granted-in-aid for scientific research from the Ministry of Education, Culture, Sports, Sciences, and Technology of Japan.
References
1. P. G. M. Wuts and T. W. Greene, 'Greene’s Protective Groups in Organic Synthesis,' 4th ed.; John Wiley & Sons, Inc.: Hoboken, NJ, 2006. CrossRef
2. (a) C. A. Bunton and R. H. De Wolfe, J. Org. Chem., 1965, 30, 1371; CrossRef (b) D. P. N. Satchell and R. S. Satchell, Chem. Soc. Rev., 1990, 19, 55; CrossRef (c) P. Deslongchamps, Y. L. Dory, and S. Li, Tetrahedron, 2000, 56, 3533. CrossRef
3. The reaction was carried out in our hands.
4. (a) H. Fujioka, Y. Sawama, N. Murata, T. Okitsu, O. Kubo, S. Matsuda, and Y. Kita, J. Am. Chem. Soc., 2004, 126, 11800; CrossRef (b) H. Fujioka, T. Okitsu, Y. Sawama, N. Murata, R. Li, and Y. Kita, J. Am. Chem. Soc., 2006, 128, 5930. CrossRef
5. (a) H. Fujioka, T. Okitsu, T. Ohnaka, Y. Sawama, O. Kubo, K. Okamoto, and Y. Kita, Adv. Synth. Catal., 2007, 349, 636; CrossRef (b) H. Fujioka, O. Kubo, K. Okamoto, K. Senami, T. Okitsu, T. Ohnaka, Y. Sawama, and Y. Kita, Heterocycles, 2009, 77, 1089. CrossRef
6. (a) H. Fujioka, O. Kubo, K. Senami, Y. Minamitsuji, and T. Maegawa, Chem. Commun., 2009, 4429; CrossRef (b) H. Fujioka, Y. Minamitsuji, O. Kubo, K. Senami, and T. Maegawa, Tetrahedron, 2011, 67, 2949. CrossRef
7. (a) H. Fujioka, K. Senami, O. Kubo, K. Yahata, Y. Minamitsuji, and T. Maegawa, Org. Lett., 2009, 11, 5138; CrossRef (b) H. Fujioka, K. Senami, O. Kubo, K. Yahata, Y. Minamitsuji, and T. Maegawa, Chem. Pharm. Bull., 2010, 58, 426. CrossRef
8. (a) M. S. Newman and R. J. Harper, Jr., J. Am. Chem. Soc., 1958, 80, 6350; CrossRef (b) W. A. R. Van Heeswijk, J. B. Goedhart, and J. F. G. Vliegenthart, Carbohydr. Res., 1977, 58, 337; CrossRef (c) J. M. T. Tronchet, G. Zosimo-Landolfo, F. Villedon-Denaide, M. Balkadjian, D. Cabrini, and F. Barbalat-Rey, J. Carbohydr. Chem., 1990, 9, 823; CrossRef (d) J. D. White, J. H. Cammack, K. Sakuma, G. W. Rewcastle, and R. K. Widener, J. Org. Chem., 1995, 60, 3600. CrossRef
9. Y. Guindon, C. Yoakim, and H. E. Morton, J. Org. Chem., 1984, 49, 3912. CrossRef
10. D. Landini, F. Montanari, and F. Rolla, Synthesis, 1979, 134. CrossRef