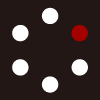
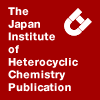
HETEROCYCLES
An International Journal for Reviews and Communications in Heterocyclic ChemistryWeb Edition ISSN: 1881-0942
Published online by The Japan Institute of Heterocyclic Chemistry
e-Journal
Full Text HTML
Received, 26th June, 2012, Accepted, 27th July, 2012, Published online, 30th July, 2012.
DOI: 10.3987/COM-12-S(N)58
■ Direct Catalytic Asymmetric Vinylogous Michael Reaction of α,β-Unsaturated γ-Butyrolactam under Dinuclear Nickel Schiff Base Catalysis
Hirooki Tanabe, Yingjie Xu, Bo Sun, Shigeki Matsunaga,* and Masakatsu Shibasaki*
Graduate School of Pharmaceutical Sciences, The University of Tokyo, 7-3-1 Hongo, Bunkyo-ku, Tokyo 113-0033, Japan
Abstract
Direct catalytic asymmetric vinylogous Michael reaction is described. 2.5 mol % of homobimetallic (S)-Ni2-Schiff base complex efficiently catalyzed the addition of α,β-unsaturated γ-butyrolactam to nitroalkenes under simple proton transfer conditions, giving vinylogous Michael products in 83-99% yield, 16:1->30:1 dr, and 96-99% ee.INTRODUCTION
Chiral butyrolactams are ubiquitous heterocyclic structural motifs found in many natural products and biologically active compounds, e.g. lactacystin,1 a proteasome inhibitor, and A-315675,2 an anti-influenza agent. The synthetic utility of these compounds has led to the development of various methods to synthesize chiral butyrolactams.3 Catalytic asymmetric vinylogous reactions of α,β-unsaturated γ-butyrolactams can be a very powerful and straightforward method for stereoselective synthesis of versatile building blocks towards functionalized chiral butyrolactams. In contrast to related γ-butenolides,4 however, catalytic asymmetric vinylogous reactions using α,β-unsaturated γ-butyrolactams remained unexplored until Suga and Kekehi‘s pioneering work in 2007.5 Suga and Kekehi used preformed 2-siloxypyrroles as latent enolates to establish a Lewis acid-catalyzed enantioselective vinylogous Mukaiyama-type Michael reaction. Casiraghi, Zanardi, and Curti et al. also recently reported efficient Lewis base- and Lewis acid-catalyzed highly enantioselective vinylogous reactions.6 In terms of atom-economy, however, a direct vinylogous addition of α,β-unsaturated γ-butyrolactams under simple proton transfer conditions would be more favorable. To address this issue, we and others recently reported bifunctional metal- and organo-catalyzed enantioselective vinylogous Michael and Mannich-type reactions.7,8 In this article, we describe the full details of our work on a direct catalytic asymmetric vinylogous Michael reaction of nitroalkenes under bimetallic Schiff base 1 catalysis (Figure 1).
RESULTS AND DISCUSSION
The direct vinylogous reaction with α,β-unsaturated γ-butyrolactam 2 first requires the chemoselective activation of 2 as a donor. Electrophilic activation of the α,β-unsaturated γ-butyrolactam unit in both 2 and the vinylogous Michael products leads to undesirable side reaction such as polymerization, and must therefore be avoided. Chemoselective deprotonation from pro-nucleophile 2 while suppressing undesirable deprotonation from vinylogous Michael products is also important because epimerization of the products decreases the diastereoselectivity. These points must be carefully considered, in addition to kinetic control of the enantioselectivity, diastereoselectivity, and α/γ-selectivity of the metal dienolate. Among acid/base bifunctional catalysts developed in our group,9 bimetallic Schiff base 1 catalysts,10-14 used for catalytic deprotonation of the α-proton in carbonyl donors, showed promising results in the present vinylogous Michael reactions. Optimization studies using α,β-unsaturated γ-butyrolactam 2 and nitroalkene 3a are summarized in Table 1 and Table 2.
To select a suitable catalyst for the vinylogous reaction of 2, we screened several metals as shown in Table 1. We first utilized dinuclear Co2-110 and Mn2-111 complexes, which have been used for Michael reactions to nitroalkenes with β-keto esters and N-Boc oxindoles as donors. Use of the Co2-1 and Mn2-1 complexes (entries 1-2) resulted in good diastereoselectivity, but the yield and enantioselectivity were only moderate. On the other hand, the use of a dinuclear Ni2-1 complex12 gave promising results, affording product 4a in 64% yield, 30:1 dr, and 91% ee in THF at 25 °C (entry 3). No α-adduct was detected in entry 3. Other bimetallic catalysts, such as Zn2-1, and Cu2-1 complexes resulted in poor reactivity (entries 4-5).
To further improve the reactivity and enantioselectivity, we further optimized the reaction conditions using Ni2-1 catalysts, as summarized in Table 2. Among the solvents screened (entries 1-7), 1,4-dioxane gave the best diastereoselectivity and enantioselectivity (entry 3). Other solvents resulted in less satisfactory reactivity and/or selectivity. Increasing the amount of donor 2 improved the yield to some extent (entries 8-9). With 2 equiv of donor 2, catalyst loading was reduced to 2.5 mol %, giving the product 4a in 85% yield, >30:1 dr, and 95% ee after 20 h (entry 10). To accelerate the reaction, molecular sieves were added in entries 11-12,15 but this resulted in less satisfactory reactivity and selectivity. Raising the reaction temperature to 50 °C improved the reactivity, and product 4a was obtained in quantitative conversion after 13 h (entry 13, 98% isolated yield). It is noteworthy that high enantioselectivity was maintained even at an elevated temperature (entry 9: 95% ee vs entry 13: 97% ee). When catalyst loading was further reduced to 1.0 mol %, the reaction did not complete even when the reaction time was prolonged (36 h), and the stereoselectivity was slightly decreased (entry 14). Thus, we selected the conditions in entry 13 as optimum.
The substrate scope of the reaction under optimized reaction conditions with homodinuclear (S)-Ni2-1 is summarized in Table 3. β-Aryl-substituted nitroalkenes with either an electron-withdrawing group or an electron-donating group gave the products in high yield with high stereoselectivity (entries 2-4). Notably, sterically-hindered 2-bromo-substituted nitroalkene 3c also showed good reactivity and enantioselectivity, although the diastereoselectivity was somewhat decreased (entry 3, 16:1 dr). β-Heteroaryl-substituted nitroalkenes 3e and 3f also gave comparably high reactivity and selectivity (entries 5-6). As for β-alkyl-substituted nitroalkenes, both linear and branched nitroalkenes 3g and 3h gave the products in good yield and with high selectivity, although a slightly longer reaction time (24 h) was required (entries 7-8). Nitrodiene 3i was also applicable, giving predominantly the 1,4-adduct in 83% yield, 25:1 dr, and 99% ee (entry 9). The absolute and relative configurations of product 4b were unequivocally determined by X-ray crystallographic analysis (Figure 2),16 and those of other products were assigned by analogy.
The postulated catalytic cycle of the reaction under dinuclear nickel catalysis is shown in Figure 3. Based on previous studies of dinuclear Ni-catalysis,12 we speculate that one of the Ni-O bonds in the outer O2O2 cavity works as a Brønsted base to deprotonate 2 and generate Ni-vinylogous enolate in situ. The other Ni in the inner N2O2 cavity functions as a Lewis acid to control the position of nitroalkenes 3, similar to conventional metal-salen Lewis acid catalysis. The C-C bond-formation, followed by protonation, affords product 4 and regenerates the Ni2-1 catalyst. The high diastereoselectivity observed in Table 2 suggests that negligible undesired epimerization of the products occurred via deprotonation. A control experiment using γ-Me-substituted α,β-unsaturated γ-butyrolactam under the present Ni2-1 catalysis did not proceed, suggesting that the steric hindrance suppressed the epimerization by the Ni2-1 catalyst.
In summary, we developed a highly enantio- and diastereoselective catalytic asymmetric vinylogous Michael reaction of α,β-unsaturated γ-butyrolactam to nitroalkenes. A homodinuclear Ni2-Schiff base 1 complex was suitable for the reaction and vinylogous Michael adducts were obtained in 83-99% yield, 16:1->30:1 dr, and 96-99% ee.
EXPERIMENTAL
General: Infrared (IR) spectra were recorded on a JASCO FT/IR 410 Fourier transform infrared spectrophotometer. NMR spectra were recorded on JEOL ECX500 spectrometers, operating at 500 MHz for 1H NMR and 125.65 MHz for 13C NMR. Chemical shifts in CDCl3 were reported in the scale relative to tetramethylsilane (0 ppm) for 1H NMR. For 13C NMR, chemical shifts were reported in the scale relative to CHCl3 (77.0 ppm) as an internal reference. Column chromatography was performed with silica gel Merck 60 (230-400 mesh ASTM). Optical rotations were measured on a JASCO P-1010 polarimeter. ESI mass spectra were measured on Waters micromass ZQ (for LRMS) and ESI mass spectra for HRMS were measured on a JEOL JMS-T100LC AccuTOF spectrometer. The enantiomeric excess (ee) was determined by HPLC analysis. HPLC was performed on JASCO HPLC systems consisting of the following: pump, PU-2080 plus; detector, UV-2075 plus, measured at 254 nm; column, DAICEL CHIRALPAK IC, IB or AD-H; mobile phase, hexane-iPrOH.
General Procedure for Direct Catalytic Asymmetric Vinylogous Michael Reaction of α,β-Unsaturated γ-Butyrolactam 2:
To a stirred solution of (S)-Ni2-Schiff base 1 catalyst (4.8 mg, 7.5 µmol, 2.5 mol %) in 1,4-dioxane (2.0 mL) at room temperature were added successively α,β-unsaturated γ-butylolactam 2 (0.6 mmol) and nitroalkene 3 (0.3 mmol). The resulting mixture was stirred at 50 ºC for indicated time in Table 3. After cooling down to room temperature, silica gel (ca. 30 mg) suspension in EtOAc was added. The resulting suspension was stirred at room temperature for 15 min. Then, the mixture was filtrated to remove silica gel and the catalyst. The filtrate was concentrated under reduced pressure, and the residue was purified by silica gel flash column chromatography (hexane/ethyl acetate = 10/1 to 2/1) to give product 4.
(S)-tert-Butyl 2-((S)-2-nitro-1-phenylethyl)-5-oxo-2,5-dihydro-1H-pyrrole-1-carboxylate (4a): Colorless solid; IR (KBr) ν 3097, 2979, 1775, 1696, 1556, 1362, 1274 cm-1; 1H NMR (CDCl3) δ 1.66 (s, 9H), 4.48-4.56 (m, 1H), 4.64-4.74 (m, 2H), 4.87-4.90 (m, 1H), 6.18 (dd, J = 1.9, 6.1 Hz 1H), 7.03 (dd, J = 2.2, 6.1 Hz 1H), 7.27-7.45 (m, 5H); 13C NMR (CDCl3) δ 28.0, 43.8, 65.0, 73.2, 84.1, 127.6, 128.5, 128.6, 129.2, 134.8, 146.1, 149.2, 168.2; LRMS (ESI): m/z 355 [M+Na]+; HRMS (ESI): m/z calculated for C17H20N2O5Na+ [M+Na]+: 355.1264, found: 355.1258; [α]D24.0 –155 (c 0.93, CHCl3); HPLC (DAICEL CHIRALPAK IC, EtOH, flow rate: 0.7 mL/min, detection at 254 nm) tR 9.4 min (minor) and 13.8 min (major).
(S)-tert-Butyl 2-((S)-1-(4-bromophenyl)-2-nitroethyl)-5-oxo-2,5-dihydro-1H-pyrrole-1-carboxylate (4b): Colorless solid; IR (KBr) ν 3103, 2979, 2933, 1759, 1555, 1489, 1368, 1298, 1253, 1157, 1048, 1009, 822 cm-1; 1H NMR (CDCl3, 500 MHz) δ 1.63 (s, 9H), 4.52 (dd, J = 4.7, 12.6 Hz, 1H), 4.56-4.60 (m, 1H), 4.66 (dd, J = 9.5, 12.6 Hz, 1H), 4.83-4.86 (m, 1H), 6.16 (dd, J = 1.7, 6.2 Hz, 1H), 7.00 (dd, J = 2.1, 6.2 Hz, 1H), 7.12-7.15 (m, 2H), 7.51-7.54 (m, 2H); 13C NMR (CDCl3, 125 MHz) δ 28.1, 43.5, 64.6, 73.3, 84.4, 122.6, 128.9, 129.3, 132.5, 133.8, 145.8, 149.5, 167.8; LRMS (ESI): m/z 433, 435 [M+Na]+; HRMS (FAB): m/z calculated for C17H19Br81N2O5Na+ [M+Na]+: 435.0355, found: 435.0350; HPLC (chiral column: DAICEL CHIRALPAK IC, ethanol, flow rate: 0.4 mL/min, detection: at 254 nm): tR = 15.1 min (R,R) and 24.1 min (S,S); [α]D29.7 = –114 (c 0.50, CHCl3).
(S)-tert-Butyl 2-((S)-1-(2-bromophenyl)-2-nitroethyl)-5-oxo-2,5-dihydro-1H-pyrrole-1-carboxylate (4c): Colorless solid; IR (KBr) ν 3105, 2982, 2949, 1766, 1558, 1474, 1368, 1288, 1159, 1046, 829, 772, 729 cm--1; 1H NMR (CDCl3) δ 1.65 (s, 9H), 4.66-4.75 (m, 2H), 5.10-5.16 (m, 2H), 6.10 (dd, J =1.6, 6.3 Hz, 1H), 6.98-7.01(m, 1 H), 7.10 (dd, J = 3.0, 6.3 Hz, 1H), 7.19-7.23 (m, 1H), 7.33-7.37 (m, 1H), 7.63-7.67(m,1H); 13C NMR (CDCl3) δ 28.1, 43.1, 62.1, 74.0, 84.4, 125.4, 128.0, 128.3, 130.0, 133.7, 134.1, 146.7, 149.7, 168.3; LRMS (ESI): m/z 433, 435 [M+Na]+; HRMS (FAB): m/z calculated for C17H19Br81N2O5Na+ [M+Na]+: 435.0355, found: 435.0349; [α]D24.0 –127 (c 0.99, CHCl3), HPLC (DAICEL CHIRALPAK IC, ethanol, flow rate: 0.4 mL/min, detection at 254 nm) tR 18.6 min (minor) and 27.0 min (major).
(S)-tert-Butyl 2-((S)-1-(4-methoxyphenyl)-2-nitroethyl)-5-oxo-2,5-dihydro-1H-pyrrole-1-carboxylate (4d): Colorless oil; IR (neat) ν 2979, 2839, 1783, 1612, 1557, 1514, 1368, 1255, 1157, 1103, 1034, 824, 753 cm-1; 1H NMR (CDCl3, 500 MHz) δ 1.65 (s, 9H), 3.80 (s, 3H), 4.49 (dd, J = 4.3, 12.5 Hz, 1H), 4.56-4.60 (m, 1H), 4.64 (dd, J = 9.6, 12.5 Hz, 1H), 4.83-4.86 (m, 1H), 6.16 (dd, J = 1.9, 6.1 Hz, 1H), 6.90-6.93 (m, 2H), 7.04 (dd, J = 2.1, 6.1 Hz, 1H), 7.15-7.19 (m, 2H); 13C NMR (CDCl3, 125 MHz) δ 28.1, 43.3, 55.3, 65.2, 73.7, 84.2, 144.7, 126.5, 128.6, 128.8, 146.4, 149.5, 159.6, 168.2; LRMS (ESI): m/z 385 [M+Na]+; HRMS (FAB): m/z calculated for C18H22NaN2O6+ [M+Na]+: 385.1370, found: 385.1364; HPLC (chiral column: DAICEL CHIRALPAK IC, ethanol, flow rate: 0.4 mL/min, detection: at 254 nm): tR = 20.2 min (minor) and 29.7 min (major); [α]D24.9 = –94.7 (c 0.90, CHCl3).
(S)-tert-Butyl 2-((S)-1-(furan-2-yl)-2-nitroethyl)-5-oxo-2,5-dihydro-1H-pyrrole-1-carboxylate (4e): Colorless solid; IR (KBr) 2969, 1789, 1698, 1552, 1369, 1288, 1254, 1162, 1051, 916, 848, 795, 755 cm-1; 1H NMR (CDCl3) δ 1.62 (s, 9H), 4.34 (dd, J = 4.3, 13.5 Hz, 1H), 4.54 (dd, J = 9.8, 13.5 Hz ,1H), 4.76 (ddd, J = 4.3, 4.3, 9.8 Hz, 1H), 4.97 (ddd, J = 1.7, 2.1, 4.3 Hz, 1H), 6.22 (dd, J = 1.7, 6.2 Hz, 1H), 6.31 (brd, J = 3.4 Hz, 1H), 6.38 (dd, J = 1.9, 3.4 Hz, 1H), 7.19 (dd, J = 2.1, 6.2 Hz, 1H), 7.44 (dd, J = 0.7, 1.9 Hz, 1H); 13C NMR (CDCl3) δ 28.0, 38.1, 63.4, 72.5, 84.2, 108.8, 110.7, 128.6, 143.1, 146.8, 148.9, 149.0, 168.1; LRMS (ESI): m/z 345 [M+Na]+; HRMS (ESI): m/z calculated for C15H18N2O6Na+ [M+Na]+: 345.1057, found: 345.1053; [α]D24.0 –179 (c 1.11, CHCl3), HPLC (DAICEL CHIRALPAK IC, ethanol, flow rate: 0.7 mL/min, detection at 254 nm) tR 8.6 min (minor) and 10.4 min (major).
(S)-tert-Butyl 2-((S)-2-nitro-1-(thiophen-2-yl)ethyl)-5-oxo-2,5-dihydro-1H-pyrrole-1-carboxylate (4f): Colorless solid; IR (KBr) ν 3099, 2981, 1782, 1693, 1556, 1358. 1277, 1152, 1107, 1032, 835, 714 cm-1; 1H NMR (CDCl3) δ 1.64 (s, 9H), 4.50 (dd, J = 4.8, 13.4 Hz, 1H), 4.57 (dd, J = 9.5, 13.4 Hz, 1H), 4.89 (ddd, J = 4.8, 4.8, 9.5, 1H), 4.94-4.96 (m, 1H), 6.20 (dd, J = 1.5, 6.1 Hz, 1H), 6.97 (d, J = 3.4 Hz, 1H), 7.01 (dd, J = 3.4, 5.2 Hz, 1H), 7.16 (dd, J = 2.1, 6.1 Hz, 1H), 7.30 (d, J = 5.2 Hz, 1H); 13C NMR (CDCl3) δ 28.0, 39.8, 64.8, 74.9, 84.3, 125.7, 126.3, 127.4, 128.8, 137.2, 146.3, 149.1, 168.1; LRMS (ESI): m/z 361 [M+Na]+; HRMS (ESI): m/z calculated for C15H18N2O5SNa+ [M+Na]+: 361.0829, found: 362.0826; [α]D24.0 –184 (c 1.03, CHCl3), HPLC (DAICEL CHIRALPAK IC, ethanol, flow rate: 0.4 mL/min, detection at 254 nm) tR 18.3 min (minor) and 27.1 min (major).
(S)-tert-Butyl 2-((S)-1-nitro-4-phenylbutan-2-yl)-5-oxo-2,5-dihydro-1H-pyrrole-1-carboxylate (4g): Colorless oil; IR (neat) ν 3022, 2980, 2932, 1778, 1555, 1456, 1370, 1314, 1158, 1105, 1049, 825, 753, 700 cm-1; 1H NMR (CDCl3, 500 MHz) δ 1.49 (s, 9H), 1.77-1.82 (m, 2H), 2.72-2.75 (m, 2H), 3.27-3.33 (m, 1H), 4.04 (dd, J = 7.3, 13.1 Hz, 1H), 4.14 (dd, J = 5.2, 13.1 Hz, 1H), 4.78-4.80 (m, 1H), 6.17 (dd, J = 1.5, 6.2 Hz, 1H), 7.06 (dd, J = 1.7, 6.2 Hz, 1H), 7.13-7.27 (m, 5H); 13C NMR (CDCl3, 125 MHz) δ 27.9, 31.8, 33.3, 37.9, 63.1, 75.1, 84.0, 126.5, 128.1, 128.7, 129.2, 140.1, 145.8, 149.2, 168.2; LRMS (ESI): m/z 383 [M+Na]+; HRMS (FAB): m/z calculated for C19H24NaN2O5+ [M+Cs]+: 383.1577, found: 383.1574; HPLC (chiral column: DAICEL CHIRALPAK AD-H, solvent: hexane/2-propanol = 9/1, flow rate: 0.5 mL/min, detection: at 254 nm): tR = 31.9 min (minor) and 36.5 min (major); [α]D 29.7 = –113.6 (c = 0.70, CHCl3).
(S)-tert-Butyl 2-((S)-3-methyl-1-nitrobutan-2-yl)-5-oxo-2,5-dihydro-1H-pyrrole-1-carboxylate (4h): Colorless solid; IR (KBr) ν 3090, 2973, 1787, 1708, 1552, 1474, 1366, 1281, 1162, 1047, 848, 825, 752 cm-1; 1H NMR (CDCl3, 500 MHz) δ 1.07 (d, J = 6.7 Hz, 3H), 1.15 (d, J = 6.7 Hz, 3H), 1.57 (s, 9H), 1.77-1.84 (m, 1H), 3.11-3.16 (m, 1H), 3.92 (dd, J = 4.4, 13.8 Hz, 1H), 4.13 (dd, J = 6.9, 13.8 Hz, 1H), 4.93-4.94 (m, 1H), 6.21 (dd, J = 1.4, 6.2 Hz, 1H), 7.10 (dd, J = 1.9, 6.2 Hz, 1H); 13C NMR (CDCl3, 125 MHz) δ 20.5, 20.6, 28.0, 29.5, 43.9, 62.0, 73.6, 83.9, 129.3, 146.1, 149.0, 168.2; LRMS (ESI): m/z 321 [M+Na]+; HRMS (ESI): m/z calculated for C14H22NaN2O5+ [M+Na]+: 321.1421, found: 321.1427; HPLC (chiral column: DAICEL CHIRALPAK IC, solvent: ethanol, flow rate: 0.4 mL/min, detection: at 254 nm): tR = 14.8 min (minor) and 21.9 min (major); [α]D29.7 = –177 (c = 1.0, CHCl3).
(S)-tert-Butyl 2-((S,E)-1-nitro-4-phenylbut-3-en-2-yl)-5-oxo-2,5-dihydro-1H-pyrrole-1-carboxylate (4i): Colorless oil; IR (neat) ν 2980, 1780, 1742, 1555, 1369, 1315, 1157, 1105, 1049, 969, 824, 751, 693 cm-1; 1H NMR (CDCl3, 500 MHz) δ 1.53 (s, 9H), 4.00-4.05 (m, 1H), 4.20 (dd, J = 9.9, 12.9 Hz, 1H), 4.27 (dd, J = 4.6, 12.9 Hz, 1H), 4.77-4.79 (m, 1H), 5.95 (dd, J = 8.6, 15.9 Hz, 1H), 6.13 (dd, J = 1.3, 6.1 Hz, 1H), 6.58 (d, J = 15.9 Hz, 1H), 7.12 (dd, J = 1.2, 6.1 Hz, 1H), 7.17-7.28 (m, 5H); 13C NMR (CDCl3, 125 MHz) δ 28.0, 42.5, 64.1, 74.9, 84.3, 122.2, 126.6, 128.6, 128.7, 128.8, 135.5, 136.3, 146.4, 149.5, 168.1; LRMS (ESI): m/z 381 [M+Na]+; HRMS (ESI): m/z calculated for C19H22NaN2O5+ [M+Na]+: 381.1421, found: 381.1419; HPLC (chiral column: DAICEL CHIRALPAK IB, solvent: hexane/2-propanol = 4/1, flow rate: 1.0 mL/min, detection: at 254 nm): tR = 75.2 min (minor) and 89.5 min (major); [α]D29.7 = –169 (c = 0.90, CHCl3).
ACKNOWLEDGEMENTS
This work was supported in part by Scientific Research on Innovative Areas “Molecular Activation Directed toward Straightforward Synthesis” from MEXT, Grant-in-aid for Young Scientist (A) from JSPS, the Naito Foundation, Takeda Science Foundation, and the Noguchi Institute. We thank prof. M. Kanai at the University of Tokyo for his fruitful discussion and unlimited access to analytical facilities in his lab.
References
1. S. Omura, T. Fujimoto, K. Otoguro, K. Matsuzaki, R. Moriguchi, H. Tanaka, and Y. Sasaki, J. Antibiot., 1991, 44, 113. CrossRef
2. D. A. DeGoey, H.-J. Chen, W. J. Flosi, D. J. Grampovnik, C. M. Yeung, L. L. Klein, and D. J. Kempf, J. Org. Chem., 2002, 67, 5445; CrossRef D. M. Barnes, L. Bhagavatula, J. DeMattei, A. Gupta, D. R. Hill, S. Manna, M. A. McLaughlin, P. Nichols, R. Premchandran, M. W. Rasmussen, Z. Tian, and S. J. Wittenberger, Tetrahedron: Asymmetry, 2003, 14, 3541; CrossRef For other synthetic applications and stereoselective transformation of α,β-unsaturated γ-butyrolactam unit, see reference 3.
3. Reviews: G. Casiraghi, L. Battistini, C. Curti, G. Rassu, and F. Zanardi, Chem. Rev., 2011, 111, 3076; CrossRef G. Casiraghi, F. Zanardi, L. Battistini, and G. Rassu, Synlett, 2009, 1525; CrossRef A. Gheorghe, M. Schulte, and O. Reiser, J. Org. Chem., 2006, 71, 2173. CrossRef
4. For selected examples of direct catalytic asymmetric carbon-carbon bond-forming reaction of γ-butenolides under proton transfer conditions, see: A. Yamaguchi, S. Matsunaga, and M. Shibasaki, Org. Lett., 2008, 10, 2319; CrossRef B. M. Trost and J. Hitce, J. Am. Chem. Soc., 2009, 131, 4572; CrossRef H.-L.Cui, J.-R. Huang, J. Lei, Z.-F. Wang, S. Chen, L. Wu, and Y.-C. Chen, Org. Lett., 2010, 12, 720; CrossRef H. Ube, N. Shimada, and M. Terada, Angew. Chem. Int. Ed., 2010, 49, 1858; CrossRef Y. Zhang, C. Yu, Y. Ji, and W. Wang, Chem. Asian J., 2010, 5, 1303; CrossRef J. Wang, C. Qi, Z. Ge, T. Cheng, and R. Li, Chem. Commun., 2010, 46, 2124; CrossRef Y. Yang, K. Zheng, J. Zhao, J. Shi, L. Lin, X. Liu, and X. Feng, J. Org. Chem., 2010, 75, 5382; CrossRef H. Huang, F. Yu, Z. Jin, W. Li, W. Wu, X. Liang, and J. Ye, Chem. Commun., 2010, 46, 5957; CrossRef M. Terada and K. Ando, Org. Lett., 2011, 13, 2026; CrossRef L. Zhou, L. Lin, J. Ji, M. Xie, X. Liu, and X. Feng, Org. Lett., 2011, 13, 3056. CrossRef
5. H. Suga, H. Takemoto, and A. Kakehi, Heterocycles, 2007, 71, 361. CrossRef
6. C. Curti, A. Sartori, L. Battistini, G. Rassu, F. Zanardi, and G. Casiraghi, Tetrahedron Lett., 2009, 50, 3428; CrossRef C. Curti, B. Ranieri, L. Battistini, G. Rassu, V. Zambrano, G. Pelosi, G. Casiraghi, and F. Zanardi, Adv. Synth. Catal., 2010, 352, 2011; CrossRef C. Curti, L. Battistini, B. Ranieri, G. Pelosi, G. Rassu, G. Casiraghi, and F. Zanardi, J. Org. Chem., 2011, 76, 2248; CrossRef B. Ranieri, C. Curti, L. Battistini, A. Sartori, L. Pinna, G. Casiraghi, and F. Zanardi, J. Org. Chem., 2011, 76, 10291. CrossRef
7. A part of results in this article was communicated previously, see: N. E. Shepherd, H. Tanabe, Y. Xu, S. Matsunaga, and M. Shibasaki, J. Am. Chem. Soc., 2010, 132, 3666. CrossRef
8. X. Feng, H.-L. Cui, S. Xu, L. Wu, and Y.-C. Chen, Chem. Eur. J., 2010, 16, 10309; CrossRef Y. Zhang, Y.-L. Shao, H.-S. Xu, and W. Wang, J. Org. Chem., 2011, 76, 1472; CrossRef H. Huang, Z. Jin, K. Zhu, X. Liang, and J. Ye, Angew. Chem. Int. Ed., 2011, 50, 3232; CrossRef L. Lin, J. Zhang, X. Ma, X. Fu, and R. Wang, Org. Lett., 2011, 13, 6410; CrossRef Y. Yang, S. Dong, X. Liu, L. Lin, and X. Feng, Chem. Commun., 2012, 48, 5040. CrossRef
9. For selected recent examples, see: H. Mihara, Y. Xu, N. E. Shepherd, S. Matsunaga, and M. Shibasaki, J. Am. Chem. Soc., 2009, 131, 8384; CrossRef T. Yoshino, H. Morimoto, G. Lu, S. Matsunaga, and M. Shibasaki, J. Am. Chem. Soc., 2009, 131, 17082; CrossRef S. Handa, V. Gnanadesikan, S. Matsunaga, and M. Shibasaki, J. Am. Chem. Soc., 2010, 132, 4925; CrossRef Y. Xu, L. Lin, M. Kanai, S. Matsunaga, and M. Shibasaki, J. Am. Chem. Soc., 2011, 133, 5791; CrossRef G. Lu, T. Yoshino, H. Morimoto, S. Matsunaga, and M. Shibasaki, Angew. Chem. Int. Ed., 2011, 50, 4382; CrossRef S. Kato, T. Yoshino, M. Shibasaki, M. Kanai, and S. Matsunaga, Angew. Chem. Int. Ed., 2012, 51, Early View. CrossRef
10. A dinuclear Co-catalyst for Michael reaction to nitroalkenes: Z. Chen, M. Furutachi, Y. Kato, S. Matsunaga, and M. Shibasaki, Angew. Chem. Int. Ed., 2009, 48, 2218; CrossRef M. Furutachi, Z. Chen, S. Matsunaga, and M. Shibasaki, Molecules, 2010, 15, 532. CrossRef
11. Dinuclear Mn-catalysts for Michael reaction to nitroalkenes: Y. Kato, M. Furutachi, Z. Chen, H. Mitsunuma, S. Matsunaga, and M. Shibasaki, J. Am. Chem. Soc., 2009, 131, 9168; CrossRef H. Mitsunuma, M. Shibasaki, M. Kanai, and S. Matsunaga, Angew. Chem. Int. Ed., 2012, 51, 5217. CrossRef
12. Dinuclear Ni-catalysts for Michael reaction to nitroalkenes: Y. Xu, S. Matsunaga, and M. Shibasaki, Org. Lett., 2010, 12, 3246; CrossRef H. Mitsunuma, and S. Matsunaga, Chem. Commun., 2011, 47, 469; CrossRef For the utility of Ni2-catalyst in other reactions, see: Z. Chen, H. Morimoto, S. Matsunaga, and M. Shibasaki, J. Am. Chem. Soc., 2008, 130, 2170; CrossRef Y. Xu, G. Lu, S. Matsunaga, and M. Shibasaki, Angew. Chem. Int. Ed., 2009, 48, 3353; CrossRef S. Mouri, Z. Chen, S. Matsunaga, and M. Shibasaki, Chem. Commun., 2009, 45, 5138; CrossRef S. Mouri, Z. Chen, H. Mitsunuma, M. Furutachi, S. Matsunaga, and M. Shibasaki, J. Am. Chem. Soc., 2010, 132, 1255. CrossRef
13. A heterodinuclear Ni/La-catalyst for Michael reaction to nitroalkenes: M. Furutachi, S. Mouri, S. Matsunaga, and M. Shibasaki, Chem. Asian J., 2010, 15, 2351. CrossRef
14. For selected examples of related bifunctional bimetallic Schiff base complexes in asymmetric catalysis, see V. Annamalai, E. F. DiMauro, P. J. Carroll, and M. C. Kozlowski, J. Org. Chem., 2003, 68, 1973; CrossRef and references therein; M. Yang, C. Zhu, F. Yuan, Y. Huang, and Y. Pan, Org. Lett., 2005, 7, 1927; CrossRef W. Hirahata, R. M. Thomas, E. B. Lobkovsky, and G. W. Coates, J. Am. Chem. Soc., 2008, 130, 17658; CrossRef C. Mazet and E. N. Jacobsen, Angew. Chem. Int. Ed., 2008, 47, 1762; CrossRef B. Wu, J. C. Gallucci, J. R. Parquette, and T. V. RajanBabu, Angew. Chem. Int. Ed., 2009, 48, 1126; CrossRef B. Wu, J. R. Parquette, and T. V. RajanBabu, Science, 2009, 326, 1662. CrossRef
15. Molecular sieves were effective in some previously reported reactions using the dinuclear Ni2-1 catalyst. See, reference 12. In contrast, DRIERITE (CaSO4) was not effective to accelerate the reaction.
16. CCDC 786778 contains the supplementary crystallographic data for 4b. The data can be obtained free of charge from The Cambridge Crystallographic Data Centre via www.ccdc.cam.ac.uk/data_request/cif.