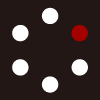
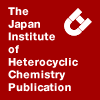
HETEROCYCLES
An International Journal for Reviews and Communications in Heterocyclic ChemistryWeb Edition ISSN: 1881-0942
Published online by The Japan Institute of Heterocyclic Chemistry
e-Journal
Full Text HTML
Received, 17th August, 2012, Accepted, 25th September, 2012, Published online, 12th October, 2012.
DOI: 10.3987/COM-12-S(N)108
■ Chemistry of Polyhalogenated Nitrobutadienes, 12: Synthesis of Novel, Highly Substituted Bi- and Tricyclic 5,6,7,8-Tetrahydro-4H-chromen-5-ones
Viktor A. Zapol'skii, Eva-Janina Vogt, Mimoza Gjikaj, and Dieter E. Kaufmann*
Institute of Organic Chemistry, Clausthal University of Technology, Leibnizstrasse 6, D-38678 Clausthal-Zellerfeld, Germany
Abstract
Knoevenagel condensation of 3,4-push-pull-substituted thiophene-2-carbaldehydes 4 with malononitrile gives the gem-dicyanovinyl-thiophenes 5, followed by a Michael addition of dimedone and a subsequent cyclization to 4-(2-thienyl)-5,6,7,8-tetrahydro-4H-chromen-5-ones 6. Protonolysis of the latter in methanol led to a mixture of four heterocycles 7-10, the main product being the novel tricyclic 9-(2-thienyl)-6,7-dihydro-5H-thieno[3,2-b]chromen-8(9H)-one derivative 7. A mechanism of the protonolysis reaction is proposed, the structures of the two main products have been confirmed by X-ray analysis.INTRODUCTION
Polyhalo-1,3-butadienes, carrying at least one nitro group, are valuable starting materials for the directed synthesis of highly functionalized heterocycles with a wide variety of synthetically and/or physiologically interesting properties,1-4 with 2-nitropentachloro-1,3-butadiene (1) being one of the most attractive members of this rather new class of synthetic building units. Starting from 1, the push-pull substituted thiophenes 3 are efficiently accessible in three steps via the aminodithiolanes 2 (Scheme 1).5 To interconnect these thiophenes, we have developed a new and unusual variant of the ipso-Vilsmeier reaction recently, in which a halogen is substituted by a formyl group under Vilsmeier-Haack conditions to give thiophene-2-carbaldehydes 4.6 This substitution reaction was first developed with electron-rich thiophenes such as 3, featuring a unique substitution pattern.
In the present paper, we mainly focus on recent progress in
a) the synthesis of substituted 2-thienyl-5,6,7,8-tetrahydro-4H-chromen-5-ones 6 by condensation of 4 with malononitrile and subsequent Michael addition/cyclization of dimedone with the Knoevenagel products 5, and
b) the protonolysis products of 6 in methanol as a solvent under sulfuric acid catalysis.
RESULTS AND DISCUSSION
Starting from 2-nitroperchlorobutadiene (1),7 4-nitrothiophenes 3a-c are accessible in very good yields (90-95%).5,6 Ipso-formylation of 3a-c under Vilsmeier-Haack conditions give the 4-nitrothiophene-2-carbaldehydes (4a-c) in good yields (70-79%).6
In a Knoevenagel condensation of 4a-c with malononitrile in ethanol at room temperature (rt) in the presence of catalytic amounts of sodium ethanolate 2-((4-nitro-3-(organylamino)-5-(vinylthio)thiophen-2-yl)methylene)malononitriles 5a-c were formed in 68-72%. Treatment of these vinylthiophenes 5a-c with dimedone led to the formation of the expected Michael adducts, followed by cyclization giving 6a-c. Carrying out the reaction with 10% excess of dimedone in ethanol in the presence of catalytic amounts of morpholine first at rt, followed by 35-40 °C (TLC monitoring), the 4H-chromene-3-carbonitriles (6) were formed in 75-91% yield. These results are depicted in Scheme 2. The formation of 2-amino-5-oxo-7,7-dimethyl-5,6,7,8-tetrahydro-4H-chromene-3-carbonitriles by Michael addition of malononitrile derivatives with dimedone has been well studied.8 These bicyclic heterocycles were synthesized from dimedone and the corresponding arylidenemalononitriles by a 6-exo-dig cyclization of δ-oxonitriles.9
2-Amino-5-oxo-5,6,7,8-tetrahydro-4H-chromene-3-carbonitriles are important synthetic building blocks with considerable pharmacological relevance. Their most important chemical transformations are (a) iodine-promoted domino reaction with various isocyanates to N-substituted 2-aminoquinoline-3-carbonitriles,10a (b) synthesis of substituted and annulated pyrano[2,3-d]pyrimidines consisting of an acylation of 2-amino-3-cyano-4H-pyrans with acetic anhydride,10b (c) simple and convenient approach to the Friedländer synthesis of pyrano[2,3-b]pyridines,10c-e d) reaction with phenylisothiocyanate and ethyl orthoformate with formation of the chromenopyrimidine and the formimidate, respectively,8f (e) synthesis of 4-isopropyl-7,7-dimethyl-2,5-dioxo-1,2,3,4,5,6,7,8-octahydroquinoline-3-carboxamide by treatment of the corresponding 4H-chromene-3-carbonitrile derivatives with a solution of bromine in aqueous methanol,10f (f) opening of a substituted 2-amino-3-cyano-4H-pyrane ring, resulting in formation of methyl acrylate derivatives,10g (g) synthesis of 4-amino-6-aryl-2-phenylpyrimidine-5-carbonitriles under the action of benzamidine hydrochloride in the presence of sodium ethanolate,10h (h) utility of 2-aminochromene-3-carbonitriles in the synthesis of chromeno[3,2-e][1,2,4]triazolo-[1,5-c]pyrimidine,10i (i) hydrolysis with hydrochloric acid under the formation of fused furo[2,3-b]furanones,10j (j) treatment of 2-aminochromene-3-carbonitriles with ammonium acetate, benzylidenemalononitrile, and ammonium thiocyanate/benzoyl chloride leads to 5-oxocyclohexanopyridin-3-carbonitriles, 3-azaacridin-4,6-diones and cyclohexano[g]-4H-pyrano[2,3-d]pyrimidin-7-ones, respectively.10k
We first tested the hydrolytic behavior of the 4H-chromene-3-carbonitriles 6a,b in aqueous methanol at rt in the presence of equimolar amounts of conc. sulfuric acid. The reaction led to the formation of a complex mixture of products, from which we could isolate four main compounds 7-10. While both thieno[3,2-b]chromen-8-ones 7a,b were obtained in 28-30% yield, a 2H-chromene-3-carboxylate 8a could only be isolated in case of the morpholino derivative 6a (10% yield). The trisubstituted thiophenes 9a,b resulted in 27-23% yield, and finally, the tetrahydro-2H-chromene 10 was formed in 29-23% (Scheme 3).
In addition to the standard NMR, MS and IR spectroscopic investigations, X-ray analyses proved the structures of the thieno[3,2-b]chromen-8(9H)-one 7a (see Figure 1) and the methyl hexahydro-2H-chromene-3-carboxylate 8a (Figure 2).11,12
Conceivable mechanisms for the formation of the compounds 7-10 are presented in Scheme 4 (thieno[3,2-b]chromen-8-ones 7), Scheme 5 (methyl 2H-chromene-3-carboxylate 8), and Scheme 6 (thiophenes 9 and chromene 10). In the cascade mechanism leading to 7 initially, the hemiaminal I is formed upon acid catalyzed addition of methanol to the push-pull substituted double bond in 6. The combination of a retro cyclization/Michael addition then leads to the formal Knoevenagel condensation product II of 4 with dimedone, which adds methanol to form the ether III by acid catalysis. The proposed intermediate II could not be isolated so far. Formally, it represents the diene part of a retro hetero-Diels-Alder reaction. Cycloreversion reactions of dihydropyrans are known, but occur at higher reaction temperatures.13 Subsequent acid-catalyzed retro Knoevenagel condensation of III leads to oxonium salt IV, which is stabilized by acetalization to V. Hetero-Diels-Alder reaction of the electron-rich acetal V and II leads to the thieno[3,2-b]chromen-8-one VI, which is protonated exclusively at the condensed thieno unit to the ammonium salt VII. Subsequent elimination of morpholine (or piperidine) from the sterically demanding intermediate VII results in the salt VIII, which is stabilized upon elimination of (methoxymethylidene)(methyl)oxonium to form the stable tricyclic heterocycle 7 (Scheme 4).
In the mechanism to 8 first the starting material 6a tautomerizes to the imine IX. Acid catalyzed hydrolysis of IX forms the acid X, then by esterification the stable methyl ester 8 is obtained (Scheme 5).
In the mechanism to 9 and 10 initially, the starting amines 6 were methylated with methyl sulfate, which forms in situ from sulfuric acid and methanol, to the methylamine derivative XI. This type of monomethylation of primary amines is known from the literature.14 Subsequent enamine-imine tautomerization to the imin XII and intramolecular addition leads to the tetracyclic intermediate XIII. Finally, [2 + 2] cycloreversion results in thiophenes 9 and chromene 10 (Scheme 6).
By treatment of tetrahydro-2H-chromene-3-carbonitrile (10) with lithium aluminium hydride in methanol at 0 °C to rt for 3 h only the carbonyl group in 10 is reduced by formation of 5-hydroxy-chromene 11 in 92% yield. The methylimino group in 10 remains unchanged under these reaction conditions (Scheme 7). To the best of our knowledge, tetrahydro-2H-chromene-3-carbonitriles of type 10 are very rare. Thus, 2-imino-7,7-dimethyl-4-methylsulfanyl-5-oxo-5,6,7,8-tetrahydro-2H-chromene-3-carbonitrile is formed in 85% yield by reaction of [bis(methylsulfanyl)methylidene]malononitrile with dimedone in refluxed 1,4-dioxane in the presence of equivalent amounts of KOH.15
The starting thiophenes 3 have a unique substitution pattern and cannot be synthesized on any other way, so far, despite wrong assumptions in the recent patent literature.16 They have proved to be interesting materials for varying applications, e.g. they can be used for prevention of retroviral infections due to their antiviral activity.16 Derivatives of 2-(thien-2-ylmethylene)malononitrile are nonlinear optical (NLO) chromophores,17a near-infrared fluorophores,17b show anti-Trypanosoma cruzi activity17c and are possible epidermal growth factor receptor tyrosine kinase inhibitors.17d Dicyanovinyl-oligothiophenes are interesting for application as donor in solar cells.17e 2-Amino-3-cyanochromenes and its derivatives are known to possess molluscicidal,8f neuroprotective,10e antibacterial,18a-b and antimicrobial activity.18c
CONCLUSION
By a Knoevenagel/Michael/cyclization sequence starting from 3-organylamino-4-nitro-5-(vinylthio)thiophene-2-carbaldehydes 4 potentially biologically active 2-amino-7,7-dimethyl-4-(3-organylamino-4-nitro-5-(vinylthio)thiophen-2-yl)-5-oxo-5,6,7,8-tetrahydro-4H-chromene-3-carbonitriles 6 were synthesized. Acidic hydrolysis of 6 in methanol at rt led to a mixture of heterocycles 7-10, mainly the novel 9-(thien-2-yl)-6,7-dihydro-5H-thieno[3,2-b]chromen-8(9H)-one derivatives 7 were formed via a reaction cascade with an unexpected hetero-Diels-Alder reaction with inverse electron demand.
EXPERIMENTAL
Melting points were determined with a Büchi apparatus 520 and are uncorrected. Thin layer chromatography (TLC) was performed on Merck TLC-plates (aluminum based) silica gel 60 F 254. FT-IR spectra were obtained in the range of 400 to 4000 cm-1 with a Bruker Vector 22 FT-IR spectrometer equipped with ALPHA's Platinum ATR single reflection diamond ATR module. Mass spectra were obtained on a Varian 320 MS Triple Quad GC/MS/MS instrument with a Varian 450-GC unit usually in direct mode with electron impact (70 eV). The elemental composition was confirmed by high-resolution EI and (+)-ESI mass spectrometry. All HRMS results were satisfactory in comparison to the calculated accurate masses of the molecular ions (±2 ppm, R ~ 10000). 1H NMR (600 MHz), 13C NMR (150 MHz): Avance III 600 MHz FT-NMR spectrometer (Bruker, Rheinstetten, Germany); 1H NMR (400 MHz), 13C NMR (100 MHz): Avance 400 FT-NMR spectrometer (also Bruker). 1H NMR (200 MHz), 13C NMR (50 MHz): DPX 200 FT-NMR spectrometer (also Bruker). 1H and 13C NMR spectra were referenced to the residual solvent peak: CDCl3: δ = 7.26 (1H), δ = 77.0 (13C) ppm; DMSO-d6: δ = 2.50 (1H), δ = 39.7 (13C) ppm. Chemical shifts δ are given in ppm. In most cases, peak assignments were accomplished by HSQC and HMBC NMR experiments. Separation of compounds (7-10) and purifications were carried out by means of column chromatography on silica gel 60 (Merck). Petroleum ether as eluent had the boiling range 60 – 70 °C.
Starting Material. 2-Nitropentachlorobuta-1,3-diene (1) was synthesized according to the literature19 from 2H-pentachlorobuta-1,3-diene with a 10:1 solution of 63% HNO3 and 98% H2SO4 in 53% yield (bp 69–71 °C, 1 mbar). Dithiolanes 2 and thiophenes 3 were prepared by previously reported procedure.5 Thiophene-2-carbaldehydes 4 were synthesized according to the literature.6 All other chemicals used in this study were commercially available.
Typical Procedure for the Preparation of Products 5a-c.
2-((3-Morpholino-4-nitro-5-(vinylthio)thien-2-yl)methylene)malononitrile (5a).
To a suspension of thiophene-2-carbaldehyde 4a (3.00 g, 10.0 mmol) and malononitrile (0.99 g, 15 mmol) in 80 mL EtOH at 0 °C was added EtONa (0.068 g, 1.0 mmol) and the resulting mixture was stirred for 2 h at 0 °C and 18 h at rt. Subsequently, the supernatant liquid was concentrated in vacuo to a volume of about 30 mL, cooled to 10 °C and treated with 2 mL conc. HCl. The precipitate was isolated, washed with H2O (2 x 20 mL), cold EtOH (1 x 5 mL), and finally dried under reduced pressure. Purification if necessary was carried out by means of column chromatography, eluent petroleum ether : EtOAc = 3 : 1. Yield 2.37 g (68%); red solid; mp 167-169 °C; IR 3107, 2960, 2923, 2869, 2214 (CN), 1546, 1522 (NO2), 1440, 1327 (NO2), 1185, 1113, 994, 949, 864, 776, 642, 602 cm-1; 1H NMR (200 MHz, CDCl3) δ 3.16-3.34 (m, 4H), 3.74-3.93 (m, 4H), 6.05 (d, J = 9.1 Hz, 1H), 6.06 (d, J = 16.4 Hz, 1H), 6.66 (dd, J = 16.4, 9.1 Hz, 1H), 7.88 (s, 1H); 13C NMR (CDCl3) δ 52.09 (NCH2), 67.05 (OCH2), 75.57 (CCN), 113.43 (CN), 114.20 (CN), 120.66 (SC), 124.76 (SCH), 130.04 (CH2), 136.52 (CNO2), 146.32 (CH), 152.88, 158.17 (SCS); MS m/z 347 (M+-H, 25), 330 (M+-H2O, 9), 271 (M+-CH=C(CN)2), 15); HRMS (EI) calcd for C14H12N4O3S2 348.0351, found: m/z 348.0349.
2-((3-Piperidino-4-nitro-5-(vinylthio)thien-2-yl)methylene)malononitrile (5b). Reaction time: 15 h at rt. Yield 72%; reddish brown solid; mp 133-134 °C; IR 2942, 2885, 2215 (CN), 1540, 1525 (NO2), 1444, 1379, 1332 (NO2), 1257, 1190, 1101, 991, 956, 858, 774, 643, 601 cm-1; 1H NMR (200 MHz, CDCl3) δ 1.55-1.88 (m, 6H), 3.06-3.38 (m, 4H), 6.01 (d, J = 8.9 Hz, 1H), 6.03 (d, J = 16.3 Hz, 1H), 6.65 (dd, J = 16.3, 8.9 Hz, 1H), 7.75 (s, 1H); 13C NMR (CDCl3) δ 23.53 (CH2), 26.41 (2CH2), 54.08 (NCH2), 73.06 (CCN), 113.88 (CN), 114.75 (CN), 117.70 (SC), 124.79 (SCH), 129.50 (CH2), 137.92 (CNO2), 146.53 (CH), 154.73, 157.84 (SCS); MS m/z 346 (M+, 45), 330 (M+-OH, 68), 312 (38), 298 (M+-HNO2, 25), 285 (30); HRMS (EI) calcd for C15H14N4O3S2 346.0558, found: m/z 346.0551.
Ethyl 4-(2-(2,2-dicyanovinyl)-4-nitro-5-(vinylthio)thien-3-yl)piperazine-1-carboxylate (5c). Reaction time: 12 h at rt. Yield 71%; red solid; mp 151-152 °C; IR 2861, 2221 (CN), 1698 (CO), 1572, 1520 (NO2), 1470, 1434, 1386, 1333 (NO2), 1243, 1128, 1116, 990, 957, 837, 763, 611 cm-1; 1H NMR (400 MHz, DMSO-d6) δ 1.21 (t, J = 7.1 Hz, 3H), 3.27-3.37 (m, 4H), 3.51-3.59 (m, 4H), 4.08 (q, J = 7.1 Hz, 2H), 6.15 (d, J = 9.0 Hz, 1H), 6.16 (d, J = 16.3 Hz, 1H), 6.88 (dd, J = 16.3, 9.1 Hz, 1H), 8.29 (s, 1H); 13C NMR (DMSO-d6) δ 14.75 (Me), 43.85 (NCH2), 52.03 (NCH2), 61.10 (OCH2), 72.64 (CCN), 114.71 (CN), 115.13 (CN), 117.62 (SC), 125.27 (SCH), 130.88 (CH2), 137.50 (CNO2), 148.86 (CH), 153.34 (CO), 154.87, 157.77 (SCS); MS m/z 419 (M+, 95), 403 (M+-O, 27), 374 (M+-OEt, 18), 275 (48), 259 (52); HRMS (EI) calcd for C17H17N5O4S2 419.0722, found: m/z 419.0722.
Typical Procedure for the Preparation of Products 6a-c.
2-Amino-7,7-dimethyl-4-(3-morpholino-4-nitro-5-(vinylthio)thien-2-yl)-5-oxo-5,6,7,8-tetrahydro-4H-chromene-3-carbonitrile (6a). To a suspension of thiophene 5a (3.48 g, 10.0 mmol) in 80 mL EtOH was added dimedone (1.54 g, 11.0 mmol) and morpholine (0.09 g, 1.0 mmol) at rt. The resulting mixture was stirred 8 h at rt and 6 h at 35-40 °C. Subsequently, the supernatant liquid was concentrated in vacuo to a volume of about 30 mL, cooled to 10 °C and treated with 2 mL conc. HCl. The precipitate was isolated, washed with H2O (2 x 20 mL), cold EtOH (1 x 5 mL), and finally dried under reduced pressure. Purification if necessary was carried out by means of column chromatography, eluent petroleum ether: EtOAc = 3 :1. Yield 4.45 g (91%); yellow solid; mp 203-205 °C; IR 3378, 3326, 3191, 2943, 2857, 2194 (CN), 1678, 1650, 1546 (NO2), 1495, 1365 (NO2), 1322, 1218, 1104, 977, 853, 657, 561 cm-1; 1H NMR (200 MHz, DMSO-d6) δ 1.02 (s, 3H, Me), 1.05 (s, 3H, Me), 2.15 (d, J = 16.1 Hz, 1H, CH2), 2.30 (d, J = 16.1 Hz, 1H, CH2), 2.40-2.60 (m, 2H, CH2), 3.03-3.17 (m, 2H, NCH2), 3.18-3.33 (m, 2H, NCH2), 3.60-3.86 (m, 4H, OCH2), 5.03 (s, 1H, CH), 5.89 (d, J = 9.2 Hz, 1H), 5.93 (d, J = 16.3 Hz, 1H), 6.78 (dd, J = 16.3, 9.2 Hz, 1H), 7.23 (br. s, 2H, NH2); 13C NMR (DMSO-d6) δ 26.99 (CH), 28.63 (Me), 28.68 (Me), 32.01 (Cq), 39.61 (CH2), 49.60 (NCH2), 50.07 (CH2), 57.09 (CCN), 67.09 (OCH2), 111.28 (Cq), 119.49 (CN), 127.08 (CH2), 127.15 (SCH), 139.15 (SC), 139.48 (CNO2), 143.74 (Cq), 144.26 (Cq), 158.61 (Cq), 164.01 (Cq), 195.97 (CO); MS m/z 488 (M+, 17), 471 (M+-OH, 14), 271 (thienyl unit, 10), 217 (chromene unit, 100) ; HRMS (EI) calcd for C22H24N4O5S2 488.1188, found: m/z 488.1187.
2-Amino-7,7-dimethyl-4-(3-piperidino-4-nitro-5-(vinylthio)thien-2-yl)-5-oxo-5,6,7,8-tetrahydro-4H-chromene-3-carbonitrile (6b). Reaction time: 5 h at 35-40 °C. Yield 89%; dark orange solid; mp 157-159 °C; IR 3331, 3171, 2937, 2850, 2200 (CN), 1681, 1654, 1542 (NO2), 1500, 1364 (NO2), 1330, 1215, 1142, 1037, 962, 855, 723, 560 cm-1; 1H NMR (200 MHz, DMSO-d6) δ 1.03 (s, 3H, Me), 1.05 (s, 3H, Me), 1.40-1.72 (m, 6H), 2.14 (d, J = 16.1 Hz, 1H, CH2), 2.30 (d, J = 16.1 Hz, 1H, CH2), 2.46 (d, J = 16.4 Hz, 1H, CH2), 2.57 (d, J = 16.4 Hz, 1H, CH2), 2.91-3.07 (m, 2H, NCH2), 3.11-3.26 (m, 2H, NCH2), 4.94 (s, 1H, CH), 5.85 (d, J = 9.0 Hz, 1H), 5.87 (d, J = 16.6 Hz, 1H), 6.75 (dd, J = 16.6, 9.0 Hz, 1H), 7.20 (br. s, 2H, NH2); 13C NMR (DMSO-d6) δ 23.91 (CH2), 26.30 (2CH2), 26.95 (CH), 28.63 (Me), 28.64 (Me), 31.95 (Cq), 39.65 (CH2), 50.10 (CH2), 50.51 (NCH2), 57.29 (CCN), 111.43 (Cq), 119.18 (CN), 126.28 (CH2), 127.37 (SCH), 140.17 (SC), 140.70 (Cq), 142.32 (CNO2), 142.74 (Cq), 158.65 (Cq), 163.85 (Cq), 195.83 (CO); MS m/z 486 (M+, 12), 469 (M+-OH, 12), 269 (thienyl unit, 3), 217 (chromene unit, 18); HRMS (EI) calcd for C23H26N4O4S2 486.1396, found: m/z 486.1398.
Ethyl 4-(2-(2-amino-3-cyano-7,7-dimethyl-5-oxo-5,6,7,8-tetrahydro-4H-chromen-4-yl)-4-nitro-5-(vinylthio)thien-3-yl)piperazine-1-carboxylate (6c). Reaction time: 3 h at 35-40 °C. Yield 75%; a yellow solid; mp 189-190 °C; IR 3392, 3326, 3195, 2959, 2193 (CN), 1673 (CO), 1607, 1549 (NO2), 1504, 1486, 1362 (NO2), 1330, 1246, 1215, 1142, 1071, 992, 720 cm-1; 1H NMR (200 MHz, DMSO-d6) δ 1.03 (s, 3H, Me), 1.05 (s, 3H, Me), 1.20 (t, J = 7.1 Hz, 3H, Me), 2.16 (d, J = 16.1 Hz, 1H, CH2), 2.29 (d, J = 16.1 Hz, 1H, CH2), 2.49 (d, J = 17.6 Hz, 1H, CH2), 2.56 (d, J = 17.6 Hz, 1H, CH2), 2.90-3.08 (m, 2H, NCH2), 3.15-3.26 (m, 2H, NCH2), 3.33-3.40 (m, 1H, NCH2), 3.40-3.48 (m, 1H, NCH2), 3.48-3.70 (m, 2H, NCH2), 4.06 (q, J = 7.1 Hz, 2H, OCH2), 5.01 (s, 1H, CH), 5.89 (d, J = 9.2 Hz, 1H), 5.93 (d, J = 16.4 Hz, 1H), 6.77 (dd, J = 16.4, 9.2 Hz, 1H), 7.24 (br s, 2H, NH2); 13C NMR (DMSO-d6) δ 14.7 (Me), 27.02 (CH), 28.64 (Me), 28.70 (Me), 32.05 (Cq), 39.83 (CH2), 44.32 (CH2), 49.21 (CH2), 50.05 (CH2), 57.12 (CCN), 61.04 (OCH2), 111.32 (Cq), 119.48 (CN), 127.09 (SCH), 127.24 (CH2), 139.15 (SC), 139.30 (CNO2), 143.55 (Cq), 144.54 (Cq), 154.90 (CO), 158.58 (Cq), 163.91 (Cq), 195.88 (CO); MS m/z 559 (M+, 20), 542 (M+-OH, 8), 342 (thienyl unit, 10), 217 (chromene unit, 100); HRMS (ESI) calcd for C25H30N5O6S2 560.1638 (M++H), found: m/z 560.1615.
Typical Procedure for Protonolysis of 2-Amino-4H-chromene-3-carbonitriles (6a,b) to Compounds 7-10.
Protonolysis of Chromene (6a) to 7a-9a and 10.
To a suspension of chromene 6a (2.44 g, 5.0 mmol) in 150 mL MeOH at rt was added 0.49 g (5.0 mmol) conc. H2SO4 and the resulting mixture was stirred at this temperature. After disappearance of starting material (about 7 d, TLC control) the supernatant liquid was concentrated in vacuo at rt to a volume of about 30 mL. The precipitated solid was filtered, washed with cold MeOH (1 x 5 mL), H2O (2 x 10 mL), Et2O (1 x 5 mL), and finally dried under reduced pressure to afford 7a. To the combined mother liquor and wash fractions cold H2O (200 mL) was added at 0 to 5 °C under vigorous stirring. The resulting mixture was extracted with CHCl3 (3 x 70 mL), and then the organic phase was washed with H2O (2 x 100 mL) und dried with CaCl2. After evaporation of the solvent and separation via column chromatography the products 8a, 9a, 10 were obtained (first petroleum ether : EtOAc mixture 5:1 was used as eluent for separation of 9a and 10, then petroleum ether : EtOAc 1:1 for isolation of 8a).
6,6-Dimethyl-9-(3-morpholino-4-nitro-5-(vinylthio)thien-2-yl)-3-nitro-2-(vinylthio)-6,7-dihydro-5H-thieno[3,2-b]chromen-8(9H)-one (7a). Rf (petroleum ether : EtOAc = 1: 1) = 0.85; Yield 0.91 g (30%) of 7a; yellow solid; mp 183-184 °C; IR 2951, 2859, 1639, 1575, 1543 (NO2), 1508 (NO2), 1363 (NO2), 1325 (NO2), 1262, 1209, 1112, 1038, 995, 859, 774, 659, 525 cm-1; 1H NMR (200 MHz, CDCl3) δ 1.17 (s, 3H, Me), 1.19 (s, 3H, Me), 2.32 (s, 2H, CH2), 2.66 (d, J = 17.8 Hz, 1H, CH2), 2.77 (d, J = 17.8 Hz, 1H, CH2), 3.05-3.26 (m, 2H, NCH2), 3.40-3.58 (m, 2H, NCH2), 3.81-3.98 (m, 4H, OCH2), 5.71 (s, 1H, CH), 5.78 (d, J = 9.0 Hz, 1H), 5.84 (d, J = 16.2 Hz, 1H), 5.91 (d, J = 9.1 Hz, 1H), 5.96 (d, J = 16.4 Hz, 1H), 6.48 (dd, J = 16.4, 9.1 Hz, 1H), 6.52 (dd, J = 16.2, 9.0 Hz, 1H); 13C NMR (CDCl3) δ 27.74 (Me), 28.85 (Me), 29.29 (CH), 32.22 (Cq), 41.36 (CH2), 49.88 (NCH2), 50.77 (CH2), 67.78 (OCH2), 109.47 (Cq), 116.51 (Cq), 125.51 (SCH), 126.21 (CH2), 126.98 (SCH), 128.30 (CH2), 138.41 (Cq), 139.38 (Cq), 139.92 (Cq), 141.69 (Cq), 143.55 (Cq), 145.20 (Cq), 147.43 (Cq), 166.05 (Cq), 196.33 (CO); MS m/z 606 (M+-H, 8), 589 (M+-H2O, 8), 335 (thienochromenone unit-H, 100), 271 (thiophene unit, 4) ; HRMS (ESI) calcd for C25H26N3O7S4 608.0654 (M++H), found: m/z 608.0648.
Methyl 2-imino-7,7-dimethyl-4-(3-morpholino-4-nitro-5-(vinylthio)thiophen-2-yl)-5-oxo-3,4,5,6,7,8-hexahydro-2H-chromene-3-carboxylate (8a). Rf (petroleum ether : EtOAc = 1: 1) = 0.38; Yield 0.26 g (10%); orange solid; mp 220-222 °C; IR 2957, 2873, 1744 (CO), 1717 (CO), 1652, 1629, 1539 (NO2), 1500, 1370, 1328 (NO2), 1261, 1169, 1103, 987, 847, 664, 555, 530 cm-1; 1H NMR (400 MHz, CDCl3) δ 1.12 (s, 3H, Me), 1.14 (s, 3H, Me), 2.27 (d, J = 16.4 Hz, 1H, CH2), 2.33 (d, J = 16.4 Hz, 1H, CH2), 2.40 (d, J = 17.3 Hz, 1H, CH2), 2.50 (d, J = 17.3 Hz, 1H, CH2), 3.01-3.18 (m, 2H, NCH2), 3.26-3.38 (m, 2H, NCH2), 3.66 (br s, 1H, CHCO2), 3.80 (s, 3H, OMe), 3.80-3.94 (m, 4H, OCH2), 5.26 (br s, 1H, CH), 5.73 (d, J = 9.2 Hz, 1H), 5.81 (d, J = 16.5 Hz, 1H), 6.48 (dd, J = 16.5, 9.2 Hz, 1H), 8.49 (br s, 1H, NH); 13C NMR (CDCl3) δ 28.03 (Me), 28.55 (Me), 32.12 (CH), 33.04 (Cq), 40.88 (CH2), 49.86 (NCH2), 50.23 (CH2), 53.4 (OMe), 54.2 (CHCO2), 67.77 (OCH2), 111.57 (Cq), 125.23 (CH2), 127.29 (SCH), 136.87 (Cq), 140.60 (Cq), 141.02 (Cq), 142.78 (CNO2), 151.82 (C=NH), 166.37 (Cq), 167.65 (Cq), 194.44 (CO); MS m/z 520 (M+-H, 1), 504 (M+-OH, 4), 462 (M+-CO2Me, 3), 272 (thiophene unit+H, 5), 250 (chromenone unit, 8); HRMS (ESI) calcd for C23H28N3O7S2 522.1387 (M++H), found: m/z 522.1363.
4-(4-Nitro-5-(vinylthio)thiophen-3-yl)morpholine (9a). Rf (petroleum ether : EtOAc = 5 : 1) = 0.14; Yield 0.37 g (27%); orange solid; mp 122-123 °C; IR 2973, 2923, 2862, 1536 (NO2), 1475, 1380, 1301 (NO2), 1184, 1110, 996, 920, 858, 765, 661, 609, 588 cm-1; 1H NMR (600 MHz, CDCl3) δ 2.96-2.98 (m, 4 H), 3.84-3.86 (m, 4 H), 5.81 (d, J = 9.2 Hz, 1 H), 5.90 (d, J = 16.4 Hz, 1 H), 6.37 (s, 1 H), 6.62 (dd, J = 16.4, 9.2 Hz, 1 H); 13C NMR (CDCl3) δ 52.62 (2 CH2), 66.60 (2 CH2), 104.58 (CH), 125.71 (CH2), 127.28 (SCH), 137.02 (CNO2), 147.48 (Cq), 148.59 (Cq); MS m/z 272 (M+, 74), 255 (M+-OH, 27), 238 (30), 227 (16); HRMS (EI) calcd for C10H12N2O3S2 272.0289 found: m/z 272.0290.
7,7-Dimethyl-2-(methylimino)-5-oxo-5,6,7,8-tetrahydro-2H-chromene-3-carbonitrile (10). Rf (petroleum ether : EtOAc = 5: 1) = 0.28; Yield 0.33 g (29%); a white solid; mp 125-126 °C; IR 3050, 2947, 2871, 2230 (CN), 1689 (CO), 1591, 1558, 1482, 1429, 1400, 1319, 1236, 1121, 1042, 978, 770, 565, 458 cm-1; 1H NMR (400 MHz, CDCl3) δ 1.10 (s, 6H, Me), 2.51 (s, 2H, CH2), 2.95 (s, 2H, CH2), 4.41 (s, 3H, NMe), 8.43 (s, 1H, CH); 13C NMR (CDCl3) δ 28.22 (2 Me), 32.78 (Cq), 46.54 (CH2), 51.40 (CH2), 55.23 (NMe), 95.88 (CCN), 114.52 (CN), 121.57 (Cq), 142.11 (CH), 165.54 (C=N), 166.41 (Cq), 195.12 (C=O); MS m/z 230 (M+, 70), 215 (M+-Me, 10), 201 (M+-CO-H, 12), 174 (M+-(MeN=C-O)+H, 100); HRMS (EI) calcd for C13H14N2O2 230.1055 found: m/z 230.1054.
Protonolysis of Chromene (6b) to Compounds 7b, 9b and 10. Reaction time: 7 d at rt (TLC control) with using of 0.49 g (1.0 mmol) of 6b and 0.10 g (1.0 mmol) conc. H2SO4 in 30 mL MeOH. Workup see protonolysis of 6a.
6,6-Dimethyl-3-nitro-9-(4-nitro-3-piperidino-5-(vinylthio)thien-2-yl)-2-(vinylthio)-6,7-dihydro-5H-thieno[3,2-b]chromen-8(9H)-one (7b). Rf (petroleum ether : EtOAc = 5: 1) = 0.42; Yield 0.17 g (28%); orange solid; mp 191-193 °C; IR 3030, 2938, 2850, 1711, 1665, 1643, 1574, 1544 (NO2), 1510 (NO2), 1362 (NO2), 1325 (NO2), 1273, 1209, 1180, 1115, 1036, 997, 966, 855, 773, 656, 538 cm-1; 1H NMR (200 MHz, CDCl3) δ 1.17 (s, 3H, Me), 1.20 (s, 3H, Me), 1.55-1.78 (m, 6H, CH2), 2.34 (br s, 2H, CH2), 2.64 (d, J = 17.9 Hz, 1H, CH2), 2.76 (d, J = 17.9 Hz, 1H, CH2), 2.89-3.24 (m, 2H, NCH2), 3.28-3.41 (m, 2H, NCH2), 5.62 (s, 1H, CH), 5.66 (d, J = 9.0 Hz, 1H), 5.72 (d, J = 16.4 Hz, 1H), 5.89 (d, J = 9.0 Hz, 1H), 5.95 (d, J = 16.4 Hz, 1H), 6.46 (dd, J = 16.4, 9.0 Hz, 1H), 6.53 (dd, J = 16.4, 9.0 Hz, 1H); 13C NMR (CDCl3) δ 23.92 (CH2), 26.71 (2CH2), 27.79 (Me), 28.87 (Me), 29.27 (CH), 32.21 (Cq), 41.41 (CH2), 50.70 (CH2), 50.80 (NCH2), 109.45 (Cq), 116.84 (Cq), 125.20 (CH2), 125.66 (SCH), 127.41 (SCH), 128.06 (CH2), 138.28 (Cq), 140.86 (Cq), 141.05 (Cq), 142.82 (Cq), 144.12 (Cq), 146.25 (Cq), 147.57 (Cq), 166.13 (Cq), 196.29 (CO); MS m/z 605 (M+, 22), 588 (M+-OH, 20), 336 (thienochromenone unit-H, 100), 269 (thiophene unit, 10) ; HRMS (ESI) calcd for C26H28N3O6S4 606.0861 (M++H), found: m/z 606.0855.
1-(4-Nitro-5-(vinylthio)thien-3-yl)piperidine (9b). Rf (petroleum ether : EtOAc = 5 : 1) = 0.55; Yield 0.062 g (23%); orange solid; mp 82-83 °C; IR 3114, 2928, 2807, 1541 (NO2), 1472, 1385, 1305 (NO2), 1191, 1112, 991, 955, 921, 857, 799, 667, 619, 589 cm-1; 1H NMR (200 MHz, CDCl3) δ 1.47-1.60 (m, 2H, CH2), 1.70-1.82 (m, 4H, CH2), 2.84-2.94 (m, 4H, NCH2), 5.79 (d, J = 9.2 Hz, 1H), 5.88 (d, J = 16.4 Hz, 1H), 6.35 (s, 1H, CH), 6.64 (dd, J = 16.4, 9.2 Hz, 1H); 13C NMR (CDCl3) δ 23.85 (CH2), 25.65 (2 CH2), 53.68 (NCH2), 104.06 (CH), 125.15 (CH2), 127.56 (SCH), 137.05 (CNO2), 147.66 (Cq), 148.40 (Cq); MS m/z 270 (M+, 77), 253 (M+-OH, 95), 236 (72), 222 (10); HRMS (EI) calcd for C11H14N2O2S2 270.0497, found: m/z 270.0505.
7,7-Dimethyl-2-(methylimino)-5-oxo-5,6,7,8-tetrahydro-2H-chromene-3-carbonitrile (10). Yield 0.053 g (23%).
5-Hydroxy-7,7-dimethyl-2-(methylimino)-5,6,7,8-tetrahydro-2H-chromene-3-carbonitrile (11). To a solution of 0.23 g (1.0 mmol) of chromen-5-one 10 in 10 mL MeOH was added at 0 °C 0.076 g (2.0 mmol) LiAlH4 and the resulting mixture was stirred 1 h at 0 °C and 2 h at rt. The supernatant liquid was concentrated in vacuo at rt to a volume of about 1 mL, cooled down to 0 °C, and then treated with 5 % aqueous HCl (10 mL). After 20 min stirring, the precipitate was filtered off, washed with H2O (2 x 5 mL), and then dried under reduced pressure to give 0.214 g (92%) of alcohol 11; bright yellow solid; mp 104-105 °C; IR 3428 (OH), 2951, 2920, 2226 (CN), 1596, 1564, 1475, 1422, 1395, 1308, 1254, 1109, 1072, 978, 762, 562, 462 cm-1; 1H NMR (400 MHz, CDCl3) δ 0.97 (s, 3H, Me), 1.12 (s, 3H, Me), 1.53 (dd, J = 12.8, 13.0 Hz, 1H, CH2), 2.02 (ddd, J = 12.8, 6.3, 2.0 Hz, 1H, CH2), 2.04 (br s, 1H, OH), 2.61 (dd, J = 18.1, 2.0 Hz, 1H, CH2), 2.70 (d, J = 18.1 Hz, 1H, CH2), 4.00 (s, 3H, NMe), 4.77 (dd, J = 13.0, 6.3 Hz, 1H, CH(OH)), 8.06 (s, 1H, =CH); 13C NMR (CDCl3) δ 26.12 (Me), 30.83 (Cq), 31.01 (Me), 45.74 (CH2), 46.52 (CH2), 54.28 (NMe), 66.13 (CH(OH)), 94.27 (CCN), 115.68 (CN), 126.59 (Cq), 142.56 (=CH), 159.38 (Cq), 162.57 (Cq); MS m/z 232 (M+, 78), 217 (M+-Me, 34), 214 (M+-H2O, 42), 199 (M+-H2O-Me, 95), 176 (M+-(MeN=C-O)+H, 100); HRMS (EI) calcd for C13H16N2O2 232.1211, found: m/z 232.1212.
ACKNOWLEDGEMENTS
We thank Prof. I. Erden, San Francisco State University, San Francisco, CA (USA), for helpful mechanistic discussions, Dr. G. Dräger, Leibniz University, Hannover (Germany), and Dr. H. Frauendorf, Georg-August University, Göttingen (Germany) for HRMS measurements.
References
1. V. A. Zapol’skii, J. C. Namyslo, A. de Meijere, and D. E. Kaufmann, Beilstein J. Org. Chem., 2012, 8, 621. CrossRef
2. E. Nutz, V. A. Zapol’skii, and D. E. Kaufmann, Synthesis, 2009, 2719. CrossRef
3. V. A. Zapol’skii, R. Fischer, J. C. Namyslo, and D. E. Kaufmann, Bioorg. Med. Chem., 2009, 17, 4206. CrossRef
4. V. A. Zapol’skii, J. C. Namyslo, C. Altug, M. Gjikaj, and D. E. Kaufmann, Synthesis, 2008, 304. CrossRef
5. V. A. Zapol’skii, J. C. Namyslo, A. E. W. Adam, and D. E. Kaufmann, Heterocycles, 2004, 63, 1281. CrossRef
6. E.-J. Vogt, V. A. Zapol’skii, E. Nutz, and D. E. Kaufmann, Z. Naturforsch., 2012, 67b, 285.
7. R. V. Kaberdin, V. I. Potkin, and V. A. Zapol’skii, Russ. Chem. Rev., 1997, 66, 827. CrossRef
8. (a) H. Junek and H. Aigner, Z. Naturforsch. B: Anorg. Chem., Org. Chem., Biochem., Biophys., Biol., 1970, 25, 1423; (b) Yu. A. Sharanin, J. Org. Chem. USSR (Engl. Transl.), 1980, 16, 1866; (c) N. M. Abd El-Rahman, A. A. El-Kateb, and M. F. Mady, Synth. Commun., 2007, 3961; CrossRef (d) A. Shaabani, M. Mohammadpour Amini, S. Ghasemi, R. Ghadari, A. H. Rezayan, Y. Fazaeli, and S. Feizi, Chem. Pharm. Bull., 2010, 58, 270; CrossRef (e) M. Seifi and H. Sheibani, Catal. Lett., 2008, 126, 275; CrossRef (f) F. M. Abdelrazek, P. Metz, O. Kataeva, A. Jäger, and S. F. El-Mahrouky, Arch. Pharm. Chem. Life Sci., 2007, 340, 543. CrossRef
9. M. Suárez, E. Salfrán, Y. Verdecia, E. Ochoa, L. Alba, N. Martín, R. Martínez-Alvarez, M. Quinteiro, C. Seoane, H. Novoa, N. Blaton, O. Peeters, and C. De Ranter, Tetrahedron, 2002, 58, 953. CrossRef
10. (a) B. Jiang, C. Li, S.-J. Tu, and F. Shi, J. Comb. Chem., 2010, 12, 482; CrossRef (b) Yu. M. Litvinov, and A. M. Shestopalov, Russ. Chem. Bull., 2008, 57, 2223; CrossRef (c) N. P. Selvam, T. H. Babu, and P. T. Perumal, Tetrahedron, 2009, 65, 8524; CrossRef (d) R. Leon, A. G. Garcia, and J. Marco-Contelles, J. Chem. Res., 2006, 536; CrossRef (e) J. Marco-Contelles, R. Leó, C. de los Ríos, A. G. García, M. G. López, and M. Villarroya, Bioorg. Med. Chem., 2006, 14, 8176; CrossRef (f) V. D. Dyachenko and A. N. Chernega, Russ. J. Gen. Chem., 2005, 75, 952; CrossRef (g) V. D. Dyachenko, Russ. J. Gen. Chem., 2004, 74, 1463; CrossRef (h) E. Salfrán, M. Suárez, Y. Verdecia, A. Alvarez, E. Ochoa, R. Martínez-Alvarez, C. Seoane, and N. Martín, J. Heterocycl. Chem., 2004, 41, 509; CrossRef (i) A. A. Hassanien, M. A. Zahran, M. S. A. El-Gaby, and M. M. Ghorab, J. Ind. Chem. Soc., 1999, 76, 350; (j) Y. Okamoto, Y. Kaneda, T. Yamasaki, T. Okawara, and M. Furukawa, J. Chem. Soc., Perkin Trans. 1, 1997, 1323; CrossRef (k) M. I. Elnagdi, F. A. M. A. Aal, and Y. M. Yassin, J. Prakt. Chemie, 1989, 331, 971. CrossRef
11. X-Ray structure analysis for 7a and 8a: Suitable single crystals of the title compounds were selected under a polarization microscope and mounted in a glass capillary (d = 0.3 mm). The crystal structures were determined by X-ray diffraction analysis using graphite monochromated Mo-Kα radiation (0.71073 Å) [T = 223(2) K], whereas the scattering intensities were collected with a single crystal diffractometer (STOE IPDS II). The crystal structures were solved by Direct Methods using SHELXS-97 and refined using alternating cycles of least squares refinements against F2 (SHELXL-97). All non-H atoms were located in Difference Fourier maps and were refined with anisotropic displacement parameters. In 7a the H positions were determined by a final Difference Fourier Synthesis, but the hydrogen positions in C24 and C25 were calculated using the riding model. In compound 8a the hydrogen position of N1 couldn’t be located.
7a crystallized in the monoclinic space group C2/c (no. 15), lattice parameters a = 22.069(7), b = 11.584(2), c = 26.900(6) Å, β = 101.60(2)°, V = 6737(3) Å3, Z = 4, dcalc. = 1.370 g cm–3, F(000) = 2912 using 6040 independent reflections and 521 parameters. R1 = 0.0537, wR2 = 0.1068 [I > 2σ(I)], goodness of fit on F2 = 0.974, residual electron density = 0.353 and –0.289 e Å–3. 8a crystallized in the triclinic space group P1 (no. 2), lattice parameters a = 9.959(3), b = 10.455(3), c = 13.185(4) Å, α = 69.76(2), β = 89.34(2), γ = 75.91(2) °, V = 1245.3(6) Å3, Z = 1, dcalc. = 1.388 g cm–3, F(000) = 546 using 4446 independent reflections and 410 parameters. R1 = 0.0804, wR2 = 0.1815 [I > 2σ(I)], goodness of fit on F2 = 1.034, residual electron density = 0.472 and –0.4990 e Å–3. Further details of the crystal structure investigations have been deposited with the Cambridge Crystallographic Data Center, CCDC 886707 for 7a and CCDC 886706 for 8a. Copies of this information may be obtained free of charge from The Director, CCDC, 12 Union Road, Cambridge, CB2 1EZ, UK (Fax: +44(1223)-336 033; e-mail: fileserv@ccdc.ac.uk or http://www.ccdc.cam.ac.uk)..
12. G. M. Sheldrick, SHELXS 97 and SHELXL 97, Program for the Solution and Refinement of Crystal Structures; University of Göttingen: Göttingen, Germany, 1997.
13. (a) N. Ota, E. Okada, D. Shibata, S. Adachi, and S. Saikawa, Heterocycles, 2010, 80, 515; CrossRef (b) D. Garcia and P. M. Keehn, J. Am. Chem. Soc., 1978, 100, 6111; CrossRef (c) J. Svetly, R. Lukas, S. Pokornj, and M. Kolinskj, Makromol. Chem., Rapid Commun., 1981, 2, 149; CrossRef (d) G. J. Besseris, J. H. Kiefer, Q. Zhang, J. A. Walker, and W. Tsang, Int. J. Chem. Kinetics, 1995, 27, 691. CrossRef
14. (a) A. K. Bhattacharyya and D. K. Nandi, Ind. Eng. Chem., Prod. Res. Div., 1976, 15, 201; CrossRef (b) E. Fretschner, H. R. Merkle, and J. Schröder, Pat. DE 19509361 A1, 1996; (c) A. G. Sukhanova, G. V. Sakovich, and G. T. Sukhanov, Chem. Heterocycl. Comp., 2008, 44, 1368; CrossRef (d) M. Sada and S. Hiroshi, Pat. JP 56-016499, 1981; (e) T. Yamamoto and M. Muraoka, Org. Prep. Proced. Int., 1984, 16, 130. CrossRef
15. G. E. H. Elgemeie, A. H. Elghandour, A. M. Elzanate, and A. M. Hussein, J. Chem. Res. (S), 1997, 7, 256. CrossRef
16. A. R. H. Guenther and J. R. Szewczyk, PCT Int. Appl. Patent 2011, WO 2011113060.
17. (a) Q. Li, C. Lu, J. Zhu, E. Fu, C. Zhong, S. Li, Y. Cui, J. Qin, and Z. Li, J. Phys. Chem. B, 2008, 112, 4545; CrossRef (b) S. T. Meek, E. E. Nesterov, and T. M. Swager, Org. Lett., 2008, 10, 2991; CrossRef (c) S. Muelas-Serrano, A. Le-Senne, C. Fernandez-Portillo, J. J. Nogal, C. Ochoa, and A. Gomez-Barrio, Memorias do Instituto Oswaldo Cruz, 2002, 97, 553; CrossRef (d) V. G Brunton, L. R. Kelland, M. J. Lear, G. J. Montgomery, J. H. Robertson, D. J. Robins, J. Queen, and P. Workman, Anti-Cancer Drug Design, 1996, 11, 265; (e) M. S. Wrackmeyer, M. Hein, A. Petrich, J. Meiss, M. Hummert, M. K. Riede, and K. Leo, Solar Energy Materials & Solar Cells, 2011, 95, 3171. CrossRef
18. (a) G. Zhang, Y. Zhang, J. Yan, R. Chen, S. Wang, Y. Ma, and R. Wang, J. Org. Chem., 2012, 77, 878; CrossRef (b) D. Kumar, V. B. Reddy, S. Sharad, U. Dube, and S. Kapur, Eur. J. Med. Chem., 2009, 44, 3805; CrossRef (c) N. J. Thumar and M. P. Patel, ARKIVOC, 2009, xiii, 363.
19. V. I. Potkin, V. A. Zapol’skii, and R. V. Kaberdin, Izv. Akad. Nauk Bel., Ser. Khim. Nauk 1996, 40, 68.