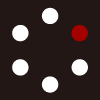
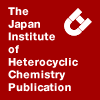
HETEROCYCLES
An International Journal for Reviews and Communications in Heterocyclic ChemistryWeb Edition ISSN: 1881-0942
Published online by The Japan Institute of Heterocyclic Chemistry
e-Journal
Full Text HTML
Received, 31st May, 2012, Accepted, 13th July, 2012, Published online, 2nd August, 2012.
DOI: 10.3987/COM-12-S(N)24
■ TRANSITION METALS IN ORGANIC SYNTHESIS, PART 104. IRON-MEDIATED TOTAL SYNTHESIS OF FUROCLAUSINE-A
Micha P. Krahl, Arndt W. Schmidt, and Hans-Joachim Knölker*
Institute of Organic Chemistry, Technical University Dresden, Bergstrasse 66, D-01069 Dresden, Germany
Abstract
Using iron-mediated coupling reactions a convergent seven-step synthesis of the furo[3,2-a]carbazole alkaloid furoclausine-A is achieved.INTRODUCTION
Over the past decades, many biologically active carbazole alkaloids with various structures have been isolated from different natural sources and a range of methodologies for their synthesis has been developed.2 Most of the carbazole alkaloids have been isolated from the taxonomically related higher plants of the genera Murraya, Glycosmis and Clausena of the family Rutaceae. Furocarbazoles represent a relatively young class of carbazole alkaloids.3 Till now, only four members of this class of carbazole alkaloids have been isolated from natural sources. In 1990, Furukawa et al. isolated the first furocarbazole alkaloids, furostifoline (1) and the isomeric eustifoline-D (2), from the acetone extract of the root bark of Murraya euchrestifolia Hayata collected in December in the central and southern parts of Taiwan (Figure 1).4 Furoclausine-A (3) and furoclausine-B (4) were isolated by Wu and co-workers in 1997 from the acetone extract of the root bark of Clausena excavata Burm. f., a shrub growing mainly in southern and south eastern Asia.5 In Taiwan, this shrub is known by the name “Sun Soak Tree”. The roots and the root bark of this tree are used in Chinese folk medicine for the treatment of several diseases such as cold and malaria.
Furostifoline (1), furoclausine-A (3) and furoclausine-B (4) feature a furo[3,2-a]carbazole skeleton, whereas eustifoline-D (2) has a furo[2,3-c]carbazole skeleton. It is believed that the central intermediate for the biosynthesis of carbazole alkaloids in higher plants is 3-methylcarbazole (5), which derives from anthranilic acid and prenyl pyrophosphate.2a,2f Diverse oxidation and oxygenation steps as well as other functionalisations like prenylation, geranylation and cyclisation reactions lead to the broad structural variety of the known carbazole alkaloids. Furoclausine-A (3) is proposed to be formed via the naturally occurring carbazole alkaloids clausine O (6), 7-hydroxyheptaphylline (7) and furoclausine-B (4), which were all isolated from the same natural source: Clausena excavata (Scheme 1).5,6
Oxygenation of 3-methylcarbazole (5) at C-2 and C-7 and oxidation of the methyl group at C-3 leads to clausine O (6). Prenylation at C-1 of clausine O (6) affords 7-hydroxyheptaphylline (7). Epoxidation of the prenyl side chain followed by intramolecular attack of the C-2 hydroxy group at the secondary carbon atom of the epoxide (path a) would provide furoclausine-B (4). Oxidation of the dihydrofuran ring with concomitant extrusion of acetone would lead to furoclausine-A (3).7 Clausine T (9) and clausine U (10), which were also isolated from Clausena excavata,5,6c probably derive from the same epoxide intermediate 8. Nucleophilic attack of the C-2 hydroxy group at the tertiary carbon atom of the epoxide (path b) would afford clausine T (9), while epoxide opening by attack of water would generate clausine U (10).
Many coumarins and carbazoles isolated from plants of the Rutaceae family show antiplatelet, antiplasmodial, antimicrobial, antitumour and anti-HIV activities.2 Recently, we described the anti-TB activity of several carbazole derivatives.8 Because of their unprecedented furan framework and the pharmacological potential, furocarbazoles have attracted a lot of interest from synthetic chemists over the last 15 years. Therefore, several strategies for the synthesis of the furocarbazole framework have been developed.3 Prior to the total synthesis of naturally occurring furocarbazole alkaloids, synthetic furocarbazoles were prepared using the Fischer indole synthesis, nitrene insertions, benzotriazole-based approaches and photocyclisation reactions.9
After the first total synthesis of furostifoline (1) reported by us in 1996,10a several alternative routes have been published.10 Using an approach which is analogous to our improved route to furostifoline (1),10b we have achieved the first total synthesis of furoclausine-A (3).11 Herein, we describe full experimental details of our approach.
RESULTS AND DISCUSSION
The retrosynthetic analysis of furoclausine-A (3) leads to the iron complex salt 11 and the arylamine 12 as precursors for an iron-mediated synthesis of this alkaloid (Scheme 2).
The iron complex salt 11 was prepared via an azadiene-catalysed complexation of 1-methoxycyclohexa1,4-diene (13) with pentacarbonyliron (Scheme 3).12 Heating of 1-methoxycyclohexadiene (13) (technical grade) and pentacarbonyliron in the presence of catalytic amounts of the 1-azabuta-1,3-diene 14 afforded on a multigram scale a 1:1 mixture of the iron complexes 15a and 15b in 78% yield. Hydride abstraction with triphenylcarbenium tetrafluoroborate and subsequent hydrolytic separation of the iron complex salts 11 and 16 provided the desired iron complex salt 11.13
The arylamine 12 was already used as building block in our iron-mediated synthesis of furostifoline (1) and was prepared as described previously (Scheme 4).10b Bromoacetaldehyde diethyl acetal is a well-established C2-building block for the synthesis of benzofurans.14 Treatment of the commercially available nitrophenol 18 with 2-bromo-1,1-diethoxyethane in the presence of potassium carbonate in N,N-dimethylformamide at reflux provided the nitrobenzene 19 in 81% yield. Subsequent hydrogenation of the nitrobenzene 19 using palladium on activated carbon as catalyst at room temperature afforded the arylamine 12 almost quantitatively. Following this route, the arylamine 12 is accessible on a multigram scale.
Electrophilic substitution of the arylamine 12 by reaction with the iron complex salt 11 in acetonitrile at room temperature led to the iron complex 20 in 87% yield. Treatment of complex 20 with an excess of iodine in pyridine at 90 °C under air induced the oxidative cyclisation with concomitant aromatisation to afford the carbazole 21 in 71% yield. The oxidative cyclisation of an analogous iron complex lacking the methoxy group at the cyclohexadiene fragment has already been described in our improved total synthesis of furostifoline (1).10b In the present case, the yields are much better (71% versus 40%) which is ascribed to the more electron-rich iron–diene fragment of complex 20.15 The oxidative cyclisation of complex 20 using ferrocenium hexafluorophosphate failed and led exclusively to decomposition of starting material. Annulation of the furan ring was achieved by heating of compound 21 in chlorobenzene at 120 °C in the presence of catalytic amounts of amberlyst 15 to afford 7-methoxyfurostifoline (22) in 81% yield. The completion of the synthesis of furoclausine-A (3) required two final steps: Cleavage of the methyl ether and oxidation of the methyl group to a formyl group. Cleavage of the methyl ether of 7-methoxyfurostifoline (22) using boron tribromide in dichloromethane afforded the hydroxycarbazole 23 in 37% yield. However, all attempts to oxidise the methyl group using either selenium(IV) oxide, 2-iodoxybenzoic acid (IBX), 2,3-dichloro-5,6-dicyano-1,4-benzoquinone (DDQ) or manganese(IV) oxide failed and resulted exclusively in decomposition of the starting material. Presumably, the electron-rich 8-hydroxyfuro[3,2-a]carbazole framework is incompatible with oxidising agents and decomposition via quinone imine methide intermediates is faster than the desired benzylic oxidation. Therefore, we tried to conduct the two final steps in reverse order. First, the methyl group at C-4 was oxidised to a formyl group using DDQ in a mixture of methanol and water (4:1) to afford O-methylfuroclausine-A (24) in moderate yield. Subsequent cleavage of the methyl ether with boron tribromide under carefully controlled reaction conditions provided furoclausine-A (3) in seven steps and 7% overall yield based on the nitrophenol 18. The spectroscopic data of our synthetic furoclausine-A (3) (UV, IR, 1H NMR, 13C NMR and MS) are in good agreement with those reported by Wu et al. for the natural product.5 However, we obtained furoclausine-A (3) as light yellow crystals (decomposition at 110 °C), whereas the natural product was described as yellow oil.
Alternatively, the methyl group of 22 was oxidised with DDQ at –5 °C using water-free, degassed methanol as solvent (Scheme 6). These reaction conditions afforded the dimethyl acetal 25 in 39% yield. Subsequent selective cleavage of the acetal using boron tribromide in dichloromethane at 0 °C afforded O-methylfuroclausine-A (24) in 98% yield. However, this approach is not superior compared to the direct route described above (cf. Scheme 5).
CONCLUSION
We have achieved the total synthesis of the furo[3,2-a]carbazole alkaloid furoclausine-A (3) in seven steps and 7% overall yield based on the commercially available nitrophenol 18. The natural product has been obtained as light yellow crystals and full spectroscopic characterisation has confirmed the structural assignment. The iron-mediated route to the synthetically challenging oxygen-substituted furo[3,2-a]carbazole framework emphasises the utility of this method.
EXPERIMENTAL
General: All reactions were carried out in dry solvents under an inert gas atmosphere (argon), unless stated otherwise. All solvents were dried and degassed using an MBraun solvent purification system. Chemicals were used as received from commercial sources. Flash chromatography: Merck silica gel (0.040–0.063 mm). Melting points: Electrothermal IA9100. UV spectra: Varian CARY 3. IR spectra: Thermo Nicolet Avatar 360 FT-IR; ν in cm–1. NMR spectra: Bruker DRX 500 and AC 300 P; δ in ppm, J in Hz. Mass spectra: Finnigan MAT-95, ionisation potential: 70 eV. Elemental analyses: EuroVector EuroEA3000.
Tricarbonyl[(1–4-η)-1-methoxycyclohexa-1,3-diene]iron (15a) and tricarbonyl[(1–4-η)-2-methoxycyclohexa-1,3-diene]iron (15b)
A dried two-neck flask with argon inlet and condenser with gas bubbler was charged under argon atmosphere with 4-methoxy-N-(3-phenylallylidene)aniline (14) (4.66 g, 19.6 mmol) and 1,4-dioxane (200 mL). Pentacarbonyliron (28.3 g, 18.8 mL, 144 mmol) was added and the solution was stirred for 30 min at room temperature. 1-Methoxycyclohexa-1,4-diene (13) (22.6 g, 205 mmol; technical grade) and 1,4-dioxane (300 mL) were added and the mixture was heated at reflux for 5 days. During the reaction a light flow of argon was directed through the apparatus. After cooling, the mixture was filtered through Celite. The Celite was then washed with hexane and the combined filtrates were evaporated to afford the iron complexes 15a and 15b (28.0 g, 78%; ratio 15a:15b = 1:1, assigned by 1H NMR spectrum); yellow oil. The mixture of 15a and 15b was used for the next step without further purification. For spectral data, see ref.12e,13
Tricarbonyl[(1–5-η)-2-methoxycyclohexadienylium]iron tetrafluoroborate (11) and tricarbonyl[(1–5-η)-1-methoxycyclohexadienylium]iron tetrafluoroborate (16)
A solution of a 1:1 mixture of the iron complexes 15a and 15b (28.0 g, 112 mmol) in dichloromethane (140 mL) was added at room temperature to a suspension of triphenylcarbenium tetrafluoroborate (46.9 g, 142 mmol) in dichloromethane (140 mL) and the reaction mixture was stirred at room temperature for 90 min. The mixture was poured into diethyl ether (1.40 L) and the precipitate was collected. The filtrate was concentrated and additional precipitate was collected. The combined precipitates were dried in vacuum to give a mixture of regioisomeric iron complex salts 11 and 16 (37.6 g, 100%; ratio of 11:16 was not determined); yellow crystals. The mixture of 11 and 16 was used for the next step without further purification.
Tricarbonyl[(1–5-η)-2-methoxycyclohexadienylium]iron tetrafluoroborate (11) and tricarbonyl- [(2–5-η)-cyclohexa-2,4-dienone]iron (17)
A mixture of the iron complex salts 11 and 16 (5.00 g, 14.9 mmol) was added to degassed water (100 mL) and the mixture was heated at reflux for 2 h. The aqueous layer was extracted several times with Et2O until the organic layer remained almost colourless. The organic layer was dried over magnesium sulfate and the solvent was evaporated to provide the iron complex 17 (1.57 g, 45%); yellow solid. For spectral data, see ref.13 The aqueous layer was concentrated to dryness and the resulting solid was dissolved in a mixture of MeCN (50 mL) and water (10 mL). Et2O (700 mL) was added and the precipitate was collected. The resulting crystals were dried in vacuum to give the iron complex salt 11 (1.84 g, 37%); yellow solid. For spectral data, see ref.13
Tricarbonyl[(1–4-η)-5-(2-amino-4-(2,2-diethoxyethoxy)-5-methylphenyl)-2-methoxycyclohexa-1,3-diene]iron (20)
A solution of the arylamine 12 (287 mg, 1.20 mmol) and the iron complex salt 11 (200 mg, 0.596 mmol) in MeCN (20 mL) was stirred at room temperature for 23 h. The solvent was removed and the residue was purified by flash chromatography (hexane–EtOAc, 2:1) on silica gel to afford the iron complex 20 (254 mg, 87%); light yellow crystals; mp 73–74 °C; UV (MeOH): λ = 205, 298 nm. IR (ATR): υ = 3364, 2976, 2932, 2038, 1953, 1622, 1510, 1484, 1458, 1423, 1375, 1309, 1226, 1113, 1065, 1020, 885, 825, 754, 680, 620, 608, 572 cm–1. 1H NMR (500 MHz, CDCl3): δ = 1.229 (t, J = 7.1 Hz, 3 H), 1.232 (t, J = 7.0 Hz, 3 H), 1.67 (ddd, J = 14.7, 3.4, 2.3 Hz, 1 H), 2.11 (s, 3 H), 2.36 (ddd, J = 14.7, 11.1, 3.7 Hz, 1 H), 2.73 (dd, J = 6.5, 3.4 Hz, 1 H), 3.12 (dt, J = 11.1, 3.4 Hz, 1 H), 3.42 (m, 3 H), 3.59–3.66 (m, 2 H), 3.68 (s, 3 H), 3.72–3.78 (m, 2 H), 3.90 (d, J = 5.2 Hz, 2 H), 4.80 (t, J = 5.2 Hz, 1 H), 5.24 (dd, J = 6.5, 2.2 Hz, 1 H), 6.12 (s, 1 H), 6.78 (s, 1 H). 13C NMR and DEPT (125 MHz, CDCl3): δ = 15.34 (2 CH3), 15.57 (CH3), 32.62 (CH2), 37.58 (CH), 53.10 (CH), 54.01 (CH), 54.39 (CH3), 62.70 (CH2), 62.73 (CH2), 66.98 (CH), 68.99 (CH2), 100.14 (CH), 100.73 (CH), 116.93 (C), 122.74 (C), 128.50 (CH), 139.93 (C), 142.10 (C), 155.53 (C), 211.25 (3 CO). MS (EI): m/z (%) = 487 (5) [M+], 431 (62), 403 (100), 347 (36), 345 (26), 329 (58), 315 (35), 299 (16), 287 (88), 285 (74), 223 (70), 179 (70), 103 (70). HRMS: m/z calcd for C23H29FeNO7 [M+]: 487.1293; found: 487.1258. Anal. Calcd for C23H29FeNO7: C, 56.69; H, 6.00; N, 2.87. Found: C, 56.77; H, 6.12; N, 2.91.
2-(2,2-Diethoxyethoxy)-7-methoxy-3-methyl-9H-carbazole (21)
Iodine (1.18 g, 4.65 mmol) was added to a solution of the iron complex 20 (713 mg, 1.46 mmol) in anhydrous pyridine (20 mL) at 90 °C and the mixture was stirred at the same temperature for 6 h under air. The reaction mixture was cooled to room temperature, a solution of sodium thiosulfate (2.37 g) and citric acid (1.25 g) in water (24 mL) was added, and the resulting mixture was extracted with Et2O several times until the organic layer remained almost colourless. The combined organic layers were washed with water three times and dried over magnesium sulfate. The solvent was evaporated and the residue was purified by flash chromatography (hexane–EtOAc, 1:1) on silica gel to provide the carbazole 21 (356 mg, 71%); colourless crystals; mp 195–196 °C; UV (MeOH): λ = 210, 236, 262, 310, 318 nm. IR (ATR): υ = 3385, 2974, 2930, 2872, 1618, 1578, 1459, 1371, 1346, 1308, 1270, 1233, 1189, 1133, 1069, 1025, 942, 886, 857, 822, 807, 745, 726, 672, 632 cm–1. 1H NMR (500 MHz, CDCl3): δ = 1.27 (t, J = 7.1 Hz, 6 H), 2.36 (s, 3 H), 3.66–3.72 (m, 2 H), 3.78–3.84 (m, 2 H), 3.87 (s, 3 H), 4.06 (d, J = 5.2 Hz, 2 H), 4.91 (t, J = 5.2 Hz, 1 H), 6.80 (dd, J = 8.4, 2.2 Hz, 1 H), 6.81 (s, 1 H), 6.85 (d, J = 2.2 Hz, 1 H), 7.68 (s, 1 H), 7.79 (d, J = 8.4 Hz, 1 H), 7.80 (br s, 1 H). 13C NMR and DEPT (125 MHz, CDCl3): δ = 15.39 (2 CH3), 16.68 (CH3), 55.62 (CH3), 62.92 (2 CH2), 69.34 (CH2), 93.80 (CH), 94.88 (CH), 100.90 (CH), 107.67 (CH), 116.73 (C), 117.38 (C), 119.34 (C), 119.97 (CH), 120.81 (CH), 138.88 (C), 140.55 (C), 155.32 (C), 157.93 (C). MS (EI): m/z (%) = 343 (100) [M+], 297 (30), 251 (15), 227 (60), 178 (69), 161 (34). HRMS: m/z calcd for C20H25NO4 [M+]: 343.1784; found: 343.1780. Anal. Calcd for C20H25NO4: C, 69.95; H, 7.34; N, 4.08. Found: C, 70.03; H, 7.48; N, 4.15.
8-Methoxy-4-methyl-10H-furo[3,2-a]carbazole (7-methoxyfurostifoline) (22)
A mixture of carbazole 21 (1.00 g, 2.92 mmol) and amberlyst 15 (411 mg, 41 wt%) in chlorobenzene (90 mL) was stirred at 120 °C for 20 h. After cooling to room temperature the solvent was evaporated and the residue was purified by flash chromatography (hexane–EtOAc, 3:1) on silica gel to provide 8-methoxy-4-methyl-10H-furo[3,2-a]carbazole (22) (592 mg, 81%); colourless crystals; mp 200–201 °C; UV (MeOH): λ = 214 (sh), 229 (sh), 239, 244, 271, 277 (sh), 306, 329 nm. IR (ATR): υ = 3429, 3151, 3122, 2967, 2928, 2828, 1721, 1619, 1579, 1486, 1446, 1354, 1316, 1272, 1252, 1192, 1153, 1042, 1017, 989, 946, 878, 824, 805, 771, 749, 734, 676 cm–1. 1H NMR (500 MHz, CDCl3): δ = 2.65 (s, 3 H), 3.90 (s, 3 H), 6.87 (dd, J = 8.5, 2.2 Hz, 1 H), 6.94 (d, J = 2.2 Hz, 1 H), 6.96 (d, J = 2.2 Hz, 1 H), 7.68 (s, 1 H), 7.70 (d, J = 2.2 Hz, 1H), 7.90 (d, J = 8.5 Hz, 1 H), 8.13 (br s, 1 H). 13C NMR and DEPT (125 MHz, CDCl3): δ = 15.42 (CH3), 55.67 (CH3), 95.18 (CH), 103.54 (CH), 108.12 (CH), 111.37 (C), 114.11 (C), 116.06 (CH), 117.94 (C), 118.00 (C), 120.16 (CH), 130.69 (C), 140.01 (C), 143.72 (CH), 153.35 (C), 157.96 (C). MS (EI): m/z (%) = 251 (100) [M+], 236 (63), 208 (22), 178 (38), 161 (15). HRMS: m/z calcd for C16H13NO2 [M+]: 251.0946; found: 251.0962. Anal. Calcd for C16H13NO2: C, 76.48; H, 5.21; N, 5.57. Found: C, 76.27; H, 5.31; N, 5.74.
4-Methyl-10H-furo[3,2-a]carbazol-8-ol (23)
A 1 M solution of boron tribromide in CH2Cl2 (400 µL, 0.400 mmol) was added at –78 °C to a solution of the carbazole 22 (51.0 mg, 0.203 mmol) in CH2Cl2 (10 mL). The solution was stirred for 2 h at –78 °C, for 1 h at –10 °C and for 3 h at room temperature. Methanol (4 mL) was added and the mixture was washed with brine. The aqueous layer was extracted with CH2Cl2 three times and the combined organic layers were dried over magnesium sulfate. The solvent was removed in vacuum and the residue was purified by flash chromatography (hexane–EtOAc, 1:1) on silica gel to afford the furo[3,2-a]carbazole 23 (17.9 mg, 37%); light yellow crystals; mp 210 °C (decomp.); UV (MeOH): λ = 212 (sh), 228 (sh), 238, 242 (sh), 271, 287 (sh), 308, 316 (sh), 333 nm. IR (ATR): υ = 3408, 2920, 1621, 1487, 1441, 1353, 1318, 1282, 1208, 1154, 1043, 955, 730 cm–1. 1H NMR (300 MHz, acetone-d6): δ = 2.63 (s, 3 H), 6.79 (dd, J = 8.4, 2.1 Hz, 1 H), 7.00 (d, J = 2.1 Hz, 1 H), 7.18 (d, J = 2.1 Hz, 1 H), 7.74 (s, 1 H), 7.89 (m, 2 H), 8.21 (s, 1 H), 10.45 (br s, 1 H). 13C NMR and DEPT (75 MHz, acetone-d6): δ = 15.46 (CH3), 97.82 (CH), 105.05 (CH), 109.50 (CH), 112.64 (C), 113.61 (C), 116.69 (CH), 118.04 (C), 119.02 (C), 120.76 (CH), 132.22 (C), 141.94 (C), 144.67 (CH), 154.02 (C), 156.45 (C). MS (EI): m/z (%) = 237 (100) [M+], 208 (7), 178 (4), 152 (4), 118 (6).
8-Methoxy-10H-furo[3,2-a]carbazole-4-carbaldehyde (24)
Method A: DDQ (211 mg, 0.929 mmol) was added at room temperature to a solution of the carbazole 22 (101 mg, 0.402 mmol) in a mixture of MeOH (40 mL) and water (10 mL) and the solution was stirred for 55 min. Et2O was added and the organic layer was washed with 2 N aqueous sodium hydroxide and brine. Then, most of the solvent was evaporated (not to dryness). Purification of the residue by flash chromatography (hexane–EtOAc, 3:4; 1% triethylamine) on silica gel afforded the aldehyde 24 (45.0 mg, 42%); light yellow crystals; mp 204–205 °C; UV (MeOH): λ = 221, 236, 284 (sh), 299, 342 nm. IR (ATR): υ = 3298, 3121, 2926, 2833, 2728, 2458, 1669, 1627, 1581, 1532, 1468, 1398, 1347, 1272, 1228, 1205, 1178, 1156, 1092, 1033, 995, 848, 774, 708, 689 cm–1. 1H NMR (500 MHz, acetone-d6): δ = 3.93 (s, 3 H), 6.98 (dd, J = 8.6, 2.2 Hz, 1 H), 7.18 (d, J = 2.2 Hz, 1 H), 7.33 (d, J = 2.2 Hz, 1 H), 8.05 (d, J = 2.2 Hz, 1 H), 8.16 (d, J = 8.6 Hz, 1 H), 8.53 (s, 1 H), 10.47 (s, 1 H), 11.28 (br s, 1 H). 13C NMR and DEPT (125 MHz, acetone-d6): δ = 55.82 (CH3), 96.40 (CH), 104.73 (CH), 110.14 (CH), 113.56 (C), 116.30 (C), 118.31 (C), 119.11 (C), 120.11 (CH), 121.58 (CH), 137.80 (C), 142.31 (C), 146.06 (CH), 154.11 (C), 159.99 (C), 187.79 (CHO). MS (EI): m/z (%) = 265 (100) [M+], 251 (38), 250 (72), 222 (42), 193 (10). HRMS: m/z calcd for C16H11NO3 [M+]: 265.0739; found: 265.0706. Anal. Calcd for C16H11NO3: C, 72.45; H, 4.18; N, 5.28. Found: C, 72.31; H, 4.20; N, 5.49.
8-Hydroxy-10H-furo[3,2-a]carbazole-4-carbaldehyde (furoclausine-A) (3)
A 1 M solution of boron tribromide in CH2Cl2 (0.75 mL, 0.75 mmol) was added dropwise to a stirred solution of the aldehyde 24 (90 mg, 0.34 mmol) in CH2Cl2 (25 mL) at –78 °C and the mixture was stirred for 3.5 d at –20 °C. MeOH (25 mL) and degassed brine were added and the layers were separated. The aqueous layer was extracted with CH2Cl2, the organic layers were combined and the solvent was evaporated. Purification of the residue by flash chromatography (hexane–EtOAc, 3:4) on silica gel provided 8-hydroxy-10H-furo[3,2-a]carbazole-4-carbaldehyde (furoclausine-A) (3) (35 mg, 41%); light yellow crystals; mp 110 °C (decomp.); UV (MeOH): λ = 220, 235, 287 (sh), 300, 345 nm. IR (ATR): υ = 3302, 1703, 1670, 1619, 1590, 1444, 1350, 1325, 1302, 1276, 1258, 1228, 1165, 1149, 1114, 1070, 1040, 993, 956, 856, 830, 810, 799, 775, 750, 730, 686, 629, 591, 549 cm–1. 1H NMR (500 MHz, acetone-d6): δ = 6.92 (dd, J = 8.4, 2.1 Hz, 1 H), 7.09 (d, J = 2.1 Hz, 1 H), 7.32 (d, J = 2.2 Hz, 1 H), 8.05 (d, J = 2.2 Hz, 1 H), 8.10 (d, J = 8.4 Hz, 1 H), 8.51 (s, 1 H), 8.54 (s, 1 H), 10.47 (s, 1 H), 11.16 (br s, 1 H). 13C NMR and DEPT (125 MHz, acetone-d6): δ = 98.22 (CH), 104.67 (CH), 110.73 (CH), 113.43 (C), 116.16 (C), 117.64 (C), 119.36 (C), 119.85 (CH), 121.62 (CH), 137.75 (C), 142.57 (C), 146.02 (CH), 154.09 (C), 157.49 (C), 187.75 (CHO). MS (EI): m/z (%) = 251 (100) [M+], 250 (74), 222 (26), 194 (11), 139 (3). HRMS: m/z calcd for C15H9NO3 [M+]: 251.0582; found: 251.0568.
4-(Dimethoxymethyl)-8-methoxy-10H-furo[3,2-a]carbazole (25)
DDQ (102 mg, 0.45 mmol) was added portionwise to a solution of carbazole 22 (50.3 mg, 0.200 mmol) in dry MeOH (25 mL) at –5 °C and the mixture was stirred at the same temperature for 1 h. After consumption of the starting material, Et2O (25 mL) was added. The solution was washed twice with aqueous 2 N sodium hydroxide and with brine. The combined organic layers were dried over magnesium sulfate and the solvent was removed in vacuo. Purification by flash chromatography (hexane–EtOAc, 1:1; 1% triethylamine) on silica gel afforded 4-(dimethoxymethyl)-8-methoxy-10H-furo[3,2-a]carbazole (25) (24.1 mg, 39%); colourless crystals; mp 124–125 °C; UV (MeOH): λ = 212 (sh), 230 (sh), 238, 243, 264 (sh), 278, 305, 314 (sh), 327 nm. IR (ATR): υ = 3416, 3134, 3112, 2926, 2542, 2216, 2068, 1734, 1617, 1530, 1486, 1445, 1342, 1274, 1250, 1203, 1159, 1122, 1031, 808, 751, 732 cm–1. 1H NMR (500 MHz, acetone-d6): δ = 3.416 (s, 3 H), 3.418 (s, 3 H), 3.91 (s, 3 H), 6.01 (s, 1 H), 6.90 (dd, J = 8.5, 2.2 Hz, 1 H), 7.13 (d, J = 2.2 Hz, 1 H), 7.23 (d, J = 2.1 Hz, 1 H), 7.92 (d, J = 2.1 Hz, 1 H), 8.06 (d, J = 8.5 Hz, 1 H), 8.11 (s, 1 H), 10.79 (br s, 1 H). 13C NMR and DEPT (125 MHz, acetone-d6): δ = 53.12 (CH3), 55.73 (2 CH3), 95.83 (CH), 100.97 (CH), 104.78 (CH), 109.09 (CH), 112.93 (C), 114.76 (CH), 115.53 (C), 118.00 (C), 118.64 (C), 120.98 (CH), 133.66 (C), 141.73 (C), 144.96 (CH), 152.51 (C), 159.25 (C). MS (EI): m/z (%) = 311 (51) [M+], 281 (60), 280 (100), 265 (52), 250 (46), 236 (11), 222 (14), 140 (17). HRMS: m/z calcd for C18H17NO4 [M+]: 311.1158; found: 311.1134. Anal. Calcd for C18H17NO4: C, 69.44; H, 5.50; N, 4.50. Found: C, 69.31; H, 5.52; N, 4.53.
8-Methoxy-10H-furo[3,2-a]carbazole-4-carbaldehyde (24)
Method B: A 1 M solution of boron tribromide in CH2Cl2 (192 μL, 0.192 mmol) was added dropwise to a solution of carbazole 25 (20.0 mg, 0.064 mmol) in CH2Cl2 (6 mL) at –78 °C. After stirring for 2 h at the same temperature, the mixture was warmed to 0 °C and stirred for additional 60 min. MeOH (6 mL) and brine (15 mL) were added and the mixture was extracted with CH2Cl2 (3 × 30 mL). The combined organic layers were dried over magnesium sulfate and the solvent was evaporated. Purification of the residue by flash chromatography (hexane–EtOAc, 1:1; 1% triethylamine) on silica gel provided 8-methoxy-10H-furo[3,2-a]carbazole-4-carbaldehyde (24) (16.6 mg, 98%). For spectral data, see above.
ACKNOWLEDGEMENTS
We thank the BASF SE, Ludwigshafen, for a gift of pentacarbonyliron.
References
1. Part 103: C. Börger, O. Kataeva, and H.-J. Knölker, Org. Biomol. Chem., 2012, 10, 7269. CrossRef
2. (a) A. W. Schmidt, K. R. Reddy, and H.-J. Knölker, Chem. Rev., 2012, 112, 3193; CrossRef (b) I. Bauer and H.-J. Knölker, Top. Curr. Chem., 2012, 309, 203; CrossRef (c) R. Forke, K. K. Gruner, K. E. Knott, S. Auschill, S. Agarwal, R. Martin, M. Böhl, S. Richter, G. Tsiavaliaris, R. Fedorov, D. J. Manstein, H. O. Gutzeit, and H.-J. Knölker, Pure Appl. Chem., 2010, 82, 1975; CrossRef (d) N. Kongkathip and B. Kongkathip, Heterocycles, 2009, 79, 121; CrossRef (e) H.-J. Knölker, Chem. Lett., 2009, 38, 8; CrossRef (f) H.-J. Knölker and K. R. Reddy, in The Alkaloids, ed. by G. A. Cordell, Academic Press, Amsterdam, 2008, vol. 65, p. 1; (g) H.-J. Knölker, Top. Curr. Chem., 2005, 244, 115; CrossRef (h) H.-J. Knölker, Curr. Org. Synth., 2004, 1, 309; CrossRef (i) D. P. Chakraborty and S. Roy, in Progress in the Chemistry of Organic Natural Products, ed. by W. Herz, H. Grisebach, G. W. Kirby, W. Steglich, and C. Tamm, Springer-Verlag, Wien, 2003, vol. 85, p. 125; (j) H.-J. Knölker and K. R. Reddy, Chem. Rev., 2002, 102, 4303; CrossRef (k) P. T. Gallagher, in Science of Synthesis, ed. by E. J. Thomas, Thieme, Stuttgart, 2001, p. 693; (l) C. J. Moody, Synlett, 1994, 681; CrossRef (m) D. P. Chakraborty, in The Alkaloids, ed. by G. A. Cordell, Academic Press, New York, 1993, vol. 44, p. 257; (n) D. P. Chakraborty and S. Roy, in Progress in the Chemistry of Organic Natural Products, ed. by W. Herz, H. Grisebach, G. W. Kirby, W. Steglich, and C. Tamm, Springer-Verlag, Wien, 1991, vol. 57, p. 71; (o) J. Bergmann and B. Pelcman, Pure Appl. Chem., 1990, 62, 1967; CrossRef (p) R. S. Kapil, in The Alkaloids, ed. by R. H. F. Manske, Academic Press, New York, 1971, vol. 13, p. 273.
3. (a) W. Fröhner, M. P. Krahl, K. R. Reddy, and H.-J. Knölker, Heterocycles, 2004, 63, 2393; CrossRef (b) H.-J. Knölker and K. R. Reddy, in Selected Methods for Synthesis and Modification of Heterocycles: The Chemistry and Biological Activity of Natural Indole Systems, Part 1, ed. by V. G. Kartsev, ICSPF Press, Moscow, 2005, vol. 4, p. 166.
4. C. Ito and H. Furukawa, Chem. Pharm. Bull., 1990, 38, 1548. CrossRef
5. T.-S. Wu, S.-C. Huang, and P.-L. Wu, Heterocycles, 1997, 45, 969. CrossRef
6. (a) V. Kumar, K. Vallipuram, A. C. Adebajo, and J. Reisch, Phytochemistry, 1995, 40, 1563; CrossRef (b) Z.-Q. Xin, J.-J. Lu, C.-Q. Ke, C.-X. Hu, L.-P. Lin, and Y. Ye, Chem. Pharm. Bull., 2008, 56, 827; CrossRef (c) T.-S. Wu, S.-C. Huang, P.-L. Wu, and C.-S. Kuoh, Phytochemistry, 1999, 52, 523. CrossRef
7. P. M. Dewick, Medicinal Natural Products: A Biosynthetic Approach, 3rd Ed., John Wiley & Sons Ltd., Chichester, 2009, p. 163.
8. (a) T. A. Choi, R. Czerwonka, W. Fröhner, M. P. Krahl, K. R. Reddy, S. G. Franzblau, and H.-J. Knölker, ChemMedChem, 2006, 1, 812; CrossRef (b) T. A. Choi, R. Czerwonka, R. Forke, A. Jäger, J. Knöll, M. P. Krahl, T. Krause, K. R. Reddy, S. G. Franzblau, and H.-J. Knölker, Med. Chem. Res., 2008, 17, 374. CrossRef
9. (a) E. Y. Murugova, O. B. Romanova, A. S. Sokolova, I. S. Nikolaeva, T. V. Pushkina, A. N. Fomina, and V. G. Granik, Khim. Farm. Zh., 1990, 24, 44; (b) D. Joseph, L. Martarello, and G. Kirsch, J. Chem. Res., Synop., 1995, 350; (c) D. Joseph, L. Martarello, and G. Kirsch, J. Chem. Res., Synop., 1995, 448; (d) G. Jones and W. H. McKinley, J. Chem. Soc., Perkin Trans. 1, 1979, 599; CrossRef (e) G. Jones and W. H. McKinley, Tetrahedron Lett., 1977, 18, 2457; CrossRef (f) E. M. Beccalli, A. Marchesini, and T. Pilati, Tetrahedron, 1993, 49, 4741; CrossRef (g) A. R. Katritzky and L. Xie, J. Org. Chem., 1995, 60, 3707. CrossRef
10. (a) H.-J. Knölker and W. Fröhner, Tetrahedron Lett., 1996, 37, 9183; CrossRef (b) H.-J. Knölker and W. Fröhner, Synthesis, 2000, 2131; CrossRef (c) H. Hagiwara, T. Choshi, H. Fujimoto, E. Sugino, and S. Hibino, Chem. Pharm. Bull., 1998, 46, 1948; CrossRef (d) H. Hagiwara, T. Choshi, J. Nobuhiro, H. Fujimoto, and S. Hibino, Chem. Pharm. Bull., 2001, 49, 881; CrossRef (e) E. M. Beccalli, F. Clerici, and A. Marchesini, Tetrahedron, 1998, 54, 11675; CrossRef (f) T. Soós, G. Timári, and G. Hajós, Tetrahedron Lett., 1999, 40, 8607; CrossRef (g) A. Yasuhara, N. Suzuki, and T. Sakamoto, Chem. Pharm. Bull., 2002, 50, 143. CrossRef
11. H.-J. Knölker and M. P. Krahl, Synlett, 2004, 528. CrossRef
12. (a) H.-J. Knölker and P. Gonser, Synlett, 1992, 517; CrossRef (b) H.-J. Knölker, P. Gonser, and P. G. Jones, Synlett, 1994, 405; CrossRef (c) H.-J. Knölker, G. Baum, N. Foitzik, H. Goesmann, P. Gonser, P. G. Jones, and H. Röttele, Eur. J. Inorg. Chem., 1998, 993; CrossRef (d) H.-J. Knölker, E. Baum, P. Gonser, G. Rohde, and H. Röttele, Organometallics, 1998, 17, 3916; CrossRef (e) H.-J. Knölker, B. Ahrens, P. Gonser, M. Heininger, and P. G. Jones, Tetrahedron, 2000, 56, 2259; CrossRef (f) H.-J. Knölker, Chem. Rev., 2000, 100, 2941; CrossRef (g) H.-J. Knölker, in Transition Metals for Organic Synthesis – Building Blocks and Fine Chemicals, Second Edition, ed. by M. Beller and C. Bolm, Wiley-VCH, Weinheim, 2004, p. 585.
13. (a) A. J. Birch, P. E. Cross, J. Lewis, D. A. White, and S. B. Wild, J. Chem. Soc. A, 1968, 332; CrossRef (b) A. J. Birch, K. B. Chamberlain, M. A. Haas, and D. J. Thompson, J. Chem. Soc., Perkin Trans. 1, 1973, 1882; CrossRef (c) R. E. Ireland, G. G. Brown, Jr., R. H. Stanford, Jr., and T. C. McKenzie, J. Org. Chem., 1974, 39, 51; CrossRef (d) A. J. Birch and K. B. Chamberlain, Org. Synth., 1977, 57, 107.
14. R. Röhrkasten and M. Konrad, in Methoden der Organischen Chemie (Houben-Weyl), ed. by R. P. Kreher, Thieme Verlag, 1994, vol. E6b, p. 33.
15. For reviews on the iron-mediated carbazole synthesis, see: (a) H.-J. Knölker, Synlett, 1992, 371; CrossRef (b) H.-J. Knölker, Chem. Soc. Rev., 1999, 28, 151; CrossRef for further applications, see: (c) H.-J. Knölker, M. Bauermeister, and J.-B. Pannek, Chem. Ber., 1992, 125, 2783; CrossRef (d) H.-J. Knölker and M. Bauermeister, Helv. Chim. Acta, 1993, 76, 2500; CrossRef (e) H.-J. Knölker and W. Fröhner, Tetrahedron Lett., 1997, 38, 1535; CrossRef (f) H.-J. Knölker, W. Fröhner, and K. R. Reddy, Eur. J. Org. Chem., 2003, 740; CrossRef (g) O. Kataeva, M. P. Krahl, and H.-J. Knölker, Org. Biomol. Chem., 2005, 3, 3099; CrossRef (h) R. Czerwonka, K. R. Reddy, E. Baum, and H.-J. Knölker, Chem. Commun., 2006, 711; CrossRef (i) K. E. Knott, S. Auschill, A. Jäger, and H.-J. Knölker, Chem. Commun., 2009, 1467; CrossRef (j) K. K. Gruner, T. Hopfmann, K. Matsumoto, A. Jäger, T. Katsuki, and H.-J. Knölker, Org. Biomol. Chem., 2011, 9, 2057; CrossRef (k) C. Thomas, O. Kataeva, and H.-J. Knölker, Synlett, 2011, 2663. CrossRef