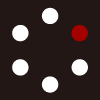
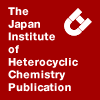
HETEROCYCLES
An International Journal for Reviews and Communications in Heterocyclic ChemistryWeb Edition ISSN: 1881-0942
Published online by The Japan Institute of Heterocyclic Chemistry
e-Journal
Full Text HTML
Received, 18th June, 2012, Accepted, 17th July, 2012, Published online, 7th August, 2012.
DOI: 10.3987/COM-12-S(N)37
■ SYNTHESIS AND BIOLOGICAL EVALUATION OF A NOVEL ACRONYCINE/DUOCARMYCIN HYBRID NATURAL PRODUCT
Lutz F. Tietze,* Arne Heins, Johannes R. Reiner, Svenia-C. Duefert, and Ingrid Schuberth
Institute of Organic and Biomolecular Chemistry, University of Göttingen, Tammannstr. 2, D-37077 Göttingen, Germany
Abstract
he design of novel natural product hybrids consisting of parts of two or more bioactive compounds may allow an access to new drugs. Here we describe a hybrid of the cytotoxic acronycine (2) and seco-duocarmycin 3, which was prepared via a selective bromination of 7 followed by the introduction of an alkyne moiety, which was further manipulated to give the epoxide 12. Cyclisation and chlorination of the formed primary hydroxy group yielded 3, which in situ would give the desired hybrid 4. The in vitro cytotoxicity test revealed a slightly higher bioactivity than acronycine (2).INTRODUCTION
Nature is an excellent source of lead structures for the fight against any type of disease. In fact, approximately 42% of the drugs that have been approved in the last 25 years are either natural products or derivatives and analogues thereof.1 For anti-cancer agents the percentage is even higher, 52% of the approved drugs are nature derived and nearly all immunosuppressive drugs needed in transplantations are natural products or their derivatives.1 Although the number of natural products being huge in the end it is limited, whereas millions of hybrids as combinations of parts of different natural products can be prepared. Recently, we and others have introduced the concept of natural product hybrids and shown its usefulness. Thus, these hybrids often exceed their parent compounds in biological activity or have other useful properties.2 One example for a natural hybrid is the indole alkaloid vincristine,3 a well known drug in cancer chemotherapy. It is a dimeric indole alkaloid consisting of vindoline – an alkaloid of the Aspidosperma subgroup – and catharanthine – a member of the Iboga subgroup of indole alkaloids. Interestingly, both monomers do not show any useful biological activity. In our approach for a selective treatment of cancer on the basis of the antibody directed enzyme prodrug therapy (ADEPT) we also used hybrids which consist of analogous of the antibiotic duocarmycin SA (1) and a sugar moiety (figure 1).4 Here a reduction of the cytotoxicity is the goal.
In our search for new anti cancer compounds with better tolerance we here describe the preparation of a new hybrid of duocarmycin SA (1) in its seco-form with the acridone alkaloid acronycine (2), which was first isolated from Acronychia baueri Schott (Rutaceae) in 1948 (Figure 1). Acronycine (2) shows a broad spectrum of activity against numerous cancer models and its cytotoxicity is based on an intercalation with double stranded DNA due to its planar aromatic system which is followed by an alkylation probably via the intermediate formation of an epoxide.5 However, clinical trials employing 2 and benzo[b]acronycine, respectively gave only poor results mainly because of their moderate potency and low water-solubility.6
On the other hand, duocarmycin SA (1), isolated in 1986 from Streptomyces sp. DO-113, shows a very high cytotoxicity with an IC50 = 10 pM,7 but has severe side effects such as myelotoxicity.8 The spirocyclopropylcyclohexadienone moiety together with the DNA-binding unit in duocarmycin SA (1) and similar compounds allows an irreversible intercalation into the minor grove of the DNA to induce apoptosis.4
For a combination of the positive effects of duocarmycin SA (1) and acronycine (2), we designed the novel acronycine/seco-duocarmycin hybrid 3, which would be transformed in situ by a Winstein cyclisation9 into the active hybrid natural product species 4, comprising the acridone structure of acronycine (2) and the spirocyclopropylhexadienone moiety of duocarmycin SA (1).
RESULTS AND DISCUSSION
Synthesis of a novel acronycine/duocarmycin hybrid natural product 4: Our approach for the synthesis of 4 was based on the formation of the chloride 3 from the hydroxy compound 13, which on the other hand should be accessible from 12 containing an aryl bromide and an epoxide moiety. Thus, halogen-metal exchange followed by a nucleophilic attack with opening of the epoxide would directly lead to 13. However, all attempts to transfer 8 into 12 in a straight forward approach using for instance the methanesulfonate of the epoxide of 1,1-dimethylallylic alcohol were not successful, since under the reaction conditions only the corresponding propenyloxirane was formed. Therefore, we used 3-chloro-3-methylbutyne for the alkylation of 8 with further manipulations to give 12. At this stage we did not try to develop an enantioselective synthesis, since we did not know which of the two enantiomers would be more active.
Commercially available 2-hydroxynaphthoic acid (5) was reacted with zinc chloride in aqueous, saturated ammonia solution at 190 °C for 4.5 d to give the amino acid 6 in 60% yield.10 Fusion with phloroglucinol (1,3,5-trihydroxybenzene) using catalytic amounts of tosylic acid in n-heptanol under reflux for 19 h led to the acridone 7 in 59% yield.11 Selective bromination at C-4 was achieved via a complexation of the vinylogous acid functionality with zinc chloride and subsequent addition of one equivalent of NBS in acetone at –78 °C for 2 h to give 8 in 59% yield. In addition, 15% of the 2-bromo derivative and 4% of the dibromo compound besides 18% of the starting material were obtained. The yield of 8 could be improved to 70% using 1.35 equivalents of NBS under otherwise identical reaction conditions with the disadvantage of the additional formation of 18% of the dibromo compound which was difficult to separate from 8. Other complexing agents as CuCl2 afforded lower yields of 8. Interestingly, reaction of 7 with 1.00 equivalent of NBS at –78 °C without the addition of ZnCl2 gave the unwanted 2-bromo compound as the main product with 43% yield. Monoalkylation with 3-chloro-3-methyl-1-butyne using catalytic amounts of copper(I) chloride in DMF at rt for 24 h yielded 9 in 68%. For this transformation the use of a Zn-salt as ZnBr2 as an additive was less appropriate, since only 6% of the desired 9 was obtained, the main product with 34% was a pyran which is probably formed from 9 by an electrophilic aromatic substitution under the reaction conditions. The alkyne 9 could easily be hydrogenated to give the alkene 10 using palladium on carbon poisoned with quinoline under a H2-atmosphere in acetone at rt for 2.5 h in 79%.12 The diol rac-11 was synthesised using the Upjohn conditions13 with catalytic amounts of potassium osmate(VI) dihydrate, DABCO and NMO in acetone at 0 °C for 3 d in 72%. Reaction of rac-11 with methanesulfonyl chloride in pyridine at 0 °C and subsequent nucleophilic substitution most probably via a primary formed methanesulfonate using potassium carbonate in methanol at rt for 3 h furnished the desired epoxide rac-12 in 48% yield.14 Lithium halogen exchange with n-BuLi in THF at −78 °C up to rt for 20 h yielded rac-13 in 62% yield.15 Transformation into the desired seco-drug rac-3 was performed using Appel conditions with triphenylphosphine and carbon tetrachloride in 1,2-dichloroethane at 50 °C for 18 h in 85% yield.16
At this stage we did not try to transform 3 into 4, since due to our experience with the seco-drugs of ducarmycin SA (1) analogues it seemed to be more appropriate to use the seco-drug in the in vitro assays. Thus, as already mentioned it could be expected that 3 is transformed in situ into 4 in the cell culture medium.
In-vitro-cytotoxicity tests: The in-vitro-cytotoxicity test of the new hybrid 3 was performed with adherent growing cells of the human bronchial carcinoma cell line A549 determining the colony forming ability; seco-drug rac-3 showed an IC50 value of 1040 nm. For comparison the same test was subjected to acronycine 2 which was synthesized according to a known procedure17 starting from phloroglucinol and anthranilic acid (2-aminobenzoic acid) in three steps showing an IC50 value of 1140 nm. For an evaluation of the advantage of the new hybrid natural product 3 as a drug candidate its in vivo bioactivity including its systemic toxicity will be studied. Moreover, compound 3 allows ample structural variations as the introduction of a tertiary amino functionality to improve water solubility by formation of a salt.
CONCLUSION
We have designed and biologically evaluated the novel acronycine/seco-duocarmycin hybrid natural product 3 by combining parts of acronycine (2) with a chloromethyl heterocyclic moiety condensed to a phenol as found in seco-duocarmycin. This could form compound 4 in situ with the bioactive spirocyclopropyldienenone moiety located in 1. The new hybrid shows a slightly higher cytotoxicity than acronycine (2), although it does not contain a double bond which could be transformed in situ into an epoxide as anticipated for the mode of action of 2. Thus, we have developed a new anticancer agent on the basis of the hybrid concept, which might be of interest for further development.
EXPERIMENTAL
General: All reactions were performed under argon in flame-dried flasks. All solvents were dried and distilled prior to use by usual laboratory methods. All reagents obtained from commercial sources were used without further purification. Thin-layer chromatography (TLC) was performed on precoated silica gel plates (TLC silica gel 60 F254, Merck) and silica gel 60 (0.032–0.063 mm, Merck) was used for column chromatography. Phosphomolybdic acid in MeOH (PMA) or vanillin in methanolic sulphuric acid were used as staining reagents for TLC. UV spectra were recorded in MCN or MeOH with a Perkin–Elmer Lambda 2 or a JASCO V-630 spectrometer. IR spectra were recorded with a JASCO FT/IR-4100 spectrometer. All substances were applied neat on an ATR unit. 1H and 13C NMR spectra were recorded on a Varian Mercury-300, Unity-300, Inova-500 and Inova-600 spectrometer and a Bruker AMX-300 spectrometer in CDCl3. Chemical shifts are given in ppm relative to tetramethylsilane (TMS), coupling constants J in Hertz. The solvent signals were used as references and the chemical shifts converted to the TMS scale (CHCl3: δH = 7.24 ppm, δC = 77.36 ppm). Multiplicities of 13C NMR peaks were determined with the APT pulse sequence. Mass spectra were measured with a Finnigan MAT 95, TSQ 7000 and LCQ instrument. HRMS was performed with a 7 T FTICR-MS APEX IV (Bruker). The following abbreviations are used in the text: EtOAc = ethyl acetate, PE = petroleum ether (bp 35–60 °C).
4-Bromo-3-(2,3-dihydroxy-1,1-dimethylpropoxy)-1-hydroxy-5H-benzo[b]acridin-12-one (rac-11): To a solution of alkene 10 (224 mg, 529 µmol, 1.00 equiv.) in acetone (10 mL) and H2O (2 mL) was added NMO (92.9 mg, 793 µmol, 1.50 equiv.), DABCO (89.0 mg, 793 µmol, 1.50 equiv.) and K2OsO4•2H2O (19.5 mg, 52.9 µmol, 0.10 equiv.). The reaction mixture was stirred at 0 °C for 3 d, sat. aq. NaHSO3-solution (2 mL) was added, the solution was further stirred for 10 min, then the volatile compounds were evaporated under reduced pressure. The remaining suspension was diluted in 1 n HCl (2 mL) and extracted with EtOAc (4 x 20 mL), the organic layer was dried over MgSO4 and silica gel (1 g) was added. Removal of the solvents under reduced pressure and purification on silica gel (PE/EtOAc = 1:1 to 100% EtOAc) gave rac-11 (174 mg, 380 µmol, 72%) as a dark orange solid. Rf = 0.17 (PE/EtOAc = 1:1); 1H-NMR (301 MHz, DMSO): δ = 1.43, 1.47 (s, 6 H, 2 x 1'-CH3), 3.47 (ddd, J = 11.0, 8.0, 5.3 Hz, 1 H, 3'-Ha), 3.74 (ddd, J = 8.1, 5.2, 2.9 Hz, 1 H, 2'-H), 3.86 (ddd, J = 11.0, 6.0, 2.8 Hz, 1 H, 3'-Hb), 4.49 (t, J = 5.7 Hz, 1 H, 3'-OH), 5.11 (d, J = 5.2 Hz, 1 H, 2'O-H), 6.63 (s, 1 H, 2-H), 7.38–7.53 (m, 1 H, 8-H), 7.61 (dd, J = 11.1, 4.0 Hz, 1 H, 9-H), 7.93 (d, J = 8.4 Hz, 1 H, 7-H), 8.15 (d, J = 8.2 Hz, 1 H, 10-H), 8.50 (s, 1 H, 6-H), 8.88 (s, 1 H, 11-H), 10.54 (s, 1 H, NH), 14.26 (s, 1 H, 1-OH); 13C-NMR (75 MHz, DMSO): δ = 22.0, 24.2 (2 x 1'-CH3), 62.0 (C-3'), 76.9 (C-2'), 86.0 (C-1'), 90.4 (C-4), 99.5 (C-2), 103.5 (C-12a), 113.6 (C-6), 119.1 (C-5a), 124.6 (C-8), 126.2 (C-7), 126.6 (C-11), 128.0 (C-6a), 128.7 (C-9), 129.3 (C-10), 135.8 (C-10a), 136.9 (C-11a), 140.5 (C-4a), 159.4 (C-3), 162.5 (C-1), 181.5 (C-12); HRMS (ESI) m/z calcd. for C22H20BrNO5: 454.0296 [M-H+]-, found: 454.0292.
4-Bromo-1-hydroxy-3-(1-methyl-1-oxiranylethoxy)-5H-benzo[b]acridin-12-one (rac-12): A solution of compound rac-11 (347 mg, 756 µmol, 1.00 equiv.) in pyridine (13 mL) was stirred at 0 °C for 30 min, then mesyl chloride (117 µL, 1.51 mmol, 2.00 equiv.) was added and stirring was continued for 1 h. After evaporation of the volatile compounds under reduced pressure the residue was dissolved in MeOH (20 mL), then K2CO3 (523 mg, 3.78 mmol, 5.00 equiv.) was added and the dark red solution was stirred for 3 h at rt. The solvent was evaporated at rt, ice-cold 1 n HCl (5 mL) was added and the aqueous layer extracted with EtOAc (3 x 10 mL). After drying over Na2SO4 silica gel (2 g) was added and the volatile compounds were removed under reduced pressure to give rac-12 (160 mg, 363 µmol, 48%) as an orange solid after purification on silica gel (PE/EtOAc = 4:1 to 3:1 to 2:1). Rf = 0.71 (PE/EtOAc = 2:1); 1H-NMR (301 MHz, DMSO): δ = 1.32, 1.50 (s, 6 H, 2 x 1'-CH3), 2.84 (dd, J = 4.7, 2.8 Hz, 1 H, 3'-Ha), 2.90 (t, J = 4.5 Hz, 1 H, 3'-Hb), 3.35 (dd, J = 4.3, 2.8 Hz, 1 H, 2'-H), 6.67 (s, 1 H, 2-H), 7.46 (d, J = 7.9 Hz, 1 H, 8-H), 7.59 (d, J = 7.2 Hz, 1 H, 9-H), 7.91 (d, J = 8.3 Hz, 1 H, 7-H), 8.13 (d, J = 8.3 Hz, 1 H, 10-H), 8.48 (s, 1 H, 6-H), 8.86 (s, 1 H, 11-H), 10.53 (s, 1 H, NH), 14.26 (s, 1 H, 1-OH); 13C-NMR (75 MHz, DMSO): δ = 20.1, 25.0 (2 x 1'-CH3), 44.6 (C-3'), 56.5 (C-2'), 82.4 (C-1'), 89.6 (C-4), 98.7 (C-2), 103.5 (C-12a), 113.6 (C-6), 119.1 (C-5a), 124.5 (C-8), 126.2 (C-7), 126.5 (C-11), 127.9 (C-6a), 128.7 (C-9), 129.3 (C-10), 135.8 (C-10a), 136.8 (C-11a), 140.4 (C-4a), 158.8 (C-3), 162.7 (C-1), 181.5 (C-12); HRMS (ESI) m/z calcd. for C22H18BrNO4: 438.0346 [M-H+]-, found: 438.0330.
5-Hydroxy-1-(hydroxymethyl)-2,2-dimethyl-1,2-dihydrobenzo[b]furo[3,2-h]acridin-6(13H)-one (rac-13): Epoxide rac-12 (59.4 mg, 135 µmol, 1.00 equiv.) was dissolved in THF (10 mL), cooled to −78 °C and stirred for 15 min at that temperature. n-BuLi (205 µL, 472 µmol, 3.50 equiv., 2.5 m in hexane) was added at −78 °C and the reaction was allowed to warm to rt within 20 h. Sat. aq. NH4Cl-sol. (2 mL) was added, the aqueous layer extracted with EtOAc (3 x 20 mL), the organic layer dried over MgSO4, silica gel (250 mg) was added and the volatile compounds were removed under reduced pressure. rac-13 (30.3 mg, 83.9 µmol, 62%) was obtained after purification on silica gel (PE/EtOAc = 3:1 " 2:1) as an orange solid. Rf = 0.21 (PE/EtOAc = 2:1); 1H-NMR (301 MHz, DMSO): δ = 1.42, 1.50 (s, 6 H, 2 x 2-CH3), 3.45 (dd, J = 8.0, 4.4 Hz, 1 H, 1-H), 3.73 (t, J = 9.3 Hz, 1 H, 1'-Ha), 3.93 (dd, J = 10.5, 4.2 Hz, 1 H, 1'-Hb), 5.96 (s, 1 H, 1'-OH), 6.01 (s, 1 H, 4-H), 7.42 (ddd, J = 8.0, 6.7, 1.1 Hz, 1 H, 10-H), 7.58 (ddd, J = 8.3, 6.7, 1.2 Hz, 1 H, 9-H), 7.85 (s, 1 H, 12-H), 7.95 (d, J = 8.1 Hz, 1 H, 11-H), 8.11 (d, J = 8.3 Hz, 1 H, 8-H), 8.86 (s, 1 H, 7-H), 11.49 (s, 1 H, NH), 14.53 (s, 1 H, 5-OH); 13C-NMR (75 MHz, DMSO): δ = 22.0, 28.4 (2 x 2-CH3), 51.0 (C-1), 60.1 (1-CH2), 89.9 (C-4), 90.1 (C-2), 102.4 (C-5a), 102.6 (C-13b), 111.4 (C-12), 119.5 (C-12a), 124.1 (C-10), 126.2 (C-7), 126.3 (C-11), 127.7 (C-11a), 128.4 (C-9), 129.3 (C-8), 135.7 (C-7a), 136.9 (C-6a), 139.8 (C-13a), 165.0 (C-3a), 165.3 (C-5), 180.9 (C-6); HRMS (ESI) m/z calcd. for C22H19NO4: 360.1241 [M-H+]-, found: 360.1236.
1-(Chloromethyl)-5-hydroxy-2,2-dimethyl-1,2-dihydrobenzo[b]furo[3,2-h]acridin-6(13H)-one (rac-3): Alcohol rac-13 (9.30 mg, 25.7 µmol, 1.00 equiv), PPh3 (20.3 mg, 77.2 µmol, 3.0 equiv.) and CCl4 (23.0 µL, 232 mmol, 9.00 equiv.) were dissolved in DCE (6 mL) and stirred for 18 h at 50 °C in a pressure flask. Silica gel (50 mg) was added, removal of the solvent under reduced pressure and purification on silica gel (PE/EtOAc = 10:1) gave rac-3 (8.31 mg, 21.9 µmol, 85%) as an orange solid. Rf = 0.71 (PE/EtOAc = 2:1); 1H-NMR (600 MHz, DMSO): δ = 1.42, 1.71 (s, 6 H, 2 x 2-CH3), 3.77 (dd, J = 6.9, 2.5 Hz, 1 H, 1-H), 3.95 (dd, J = 12.1, 2.5 Hz, 1 H, 1'-Ha), 4.01 (dd, J = 12.0, 7.0 Hz, 1 H, 1'-Hb), 6.05 (s, 1 H, 4-H), 7.46 (mc, 1 H, 10-H), 7.62 (mc, 1 H, 9-H), 8.00 (d, J = 8.7 Hz, 1 H, 11-H), 8.09 (s, 1 H, 12-H), 8.16 (d, J = 8.4 Hz, 1 H, 8-H), 8.90 (s, 1 H, 7-H), 11.12 (s, 1 H, NH), 14.63 (s, 1 H, 5-OH); 13C-NMR (126 MHz, DMSO): δ = 21.6, 28.7 (2 x 2-CH3), 43.0 (C-1'), 48.7 (C-1), 89.9 (C-4), 90.3 (C-2), 100.4 (C-13b), 102.4 (C-5a), 112.0 (C-12), 119.6 (C-12a), 124.3 (C-10), 126.2 (C-7), 126.4 (C-11), 127.7 (C-11a), 128.5 (C-9), 129.3 (C-8), 135.6 (C-7a), 137.1 (C-6a), 139.7 (C-13a), 165.2 (C-3a), 165.6 (C-5), 181.0 (C-6); HRMS (ESI) m/z calcd. for C22H19ClNO3: 378.0902 [M-H+]-, found: 378.0886.
ACKNOWLEDGEMENTS
We would like to thank the Fonds of the Chemical Industry for generous financial support. J.R.R. and S.C.D. thank the Konrad-Adenauer-Foundation for a Doctoral Fellowship.
References
1. a) D. J. Newman and G. M. Cragg, J. Nat. Prod., 2007, 70, 461; CrossRef b) J. W.-H. Li and J. C. Vederas, Science, 2009, 325, 161. CrossRef
2. L. F. Tietze, H. P. Bell, and S. Chandrasekhar, Angew. Chem. Int. Ed., 2003, 42, 3996. CrossRef
3. I. S. Johnson, J. G. Armstrong, and M. Gorman, Cancer Res., 1963, 23, 1390.
4. a) L. F. Tietze, B. Krewer, F. Major, and I. Schuberth, J. Am. Chem. Soc., 2009, 131, 13031; CrossRef b) L. F. Tietze, B. Krewer, and H. Frauendorf, Eur. J. Mass Spectrom., 2009, 15, 661; CrossRef c) L. F. Tietze, B. Krewer, J. M. von Hof, H. Frauendorf, and I. Schuberth, Toxins, 2009, 1, 134; CrossRef d) L. F. Tietze and B. Krewer, Chem. Biol. Drug Des., 2009, 74, 205; CrossRef e) L. F. Tietze, J. M. von Hof, M. Müller, B. Krewer, and I. Schuberth, Angew. Chem. Int. Ed., 2010, 49, 7336; CrossRef f) L. F. Tietze, F. Behrendt, F. Major, B. Krewer, and J. M. von Hof, Eur. J. Org. Chem., 2010, 6909; CrossRef g) L. F. Tietze and K. Schmuck, Curr. Pharm. Des., 2011, 17, 3527; CrossRef h) T. Wirth, K. Schmuck, L. F. Tietze, and S. A. Sieber, Angew. Chem., 2012, 124, 2928. CrossRef
5. a) G. K. Hughes, Nature, 1948, 162, 223; CrossRef b) L. P. Macdonald, Aust. J. Chem., 1966, 19, 275; CrossRef G. H. Svoboda, Lloydia, 1966, 29, 206; d) G. H. Svoboda, J. Pharm. Sci., 1966, 55, 758. CrossRef
6. a) J. H. Scarffe, Cancer Treat. Rep., 1983, 67, 93; b) N. Costes, J. Med. Chem., 2000, 43, 2395. CrossRef
7. a) L. J. Hanka, A. Dietz, S. A. Gerpheide, S. L. Kuentzel, and D. G. Martin, J. Antibiot., 1978, 31, 1211; CrossRef b) D. G. Martin, C. Biles, S. A. Gerpheide, L. J. Hanka, W. C. Krueger, J. P. McGovren, S. A. Mizsak, G. L. Neil, J. C. Stewart, and J. Visser, J. Antibiot., 1981, 34, 1119. CrossRef
8. a) M. Ichimura, T. Ogawa, K. Takahashi, E. Kobayashi, I. Kawamoto, T. Yasuzawa, I. Takahashi, and H. Nakano, J. Antibiot., 1990, 43, 1037; CrossRef b) M. Ichimura, T. Ogawa, S. Katsumata, K.Takahashi, I. Takahashi, and H. Nakano, J. Antibiot., 1991, 44, 1045; CrossRef c) D. L. Boger and D. S. Johnson, Angew. Chem., 1996, 108, 1542; CrossRef Angew. Chem., Int. Ed. Engl., 1996, 35, 1438. CrossRef
9. R. Baird and S. Winstein, J. Am. Chem. Soc., 1963, 85, 567. CrossRef
10. E. Taffarel, S. Chirayil, and R. P. Thummel, J. Org. Chem., 1994, 59, 823. CrossRef
11. F. Tillequin, Phytochem. Rev., 2007, 6, 65. CrossRef
12. R. Tirado and J. A. Prieto, J. Org. Chem., 1993, 58, 5666. CrossRef
13. a) V. Van Rheenen, R. C. Kelly, and D. Y. Cha, Tetrahedron Lett., 1976, 17, 1973; CrossRef b) V. Van Rheenen, D. Y. Cha, and W. M. Hartley, Org. Synth., 1988, Coll. Vol. 6, 342.
14. a) S. Boutefnouchet, J. Med. Chem., 2008, 51, 7287; CrossRef b) Y. Morimoto, Org. Biomol. Chem., 2008, 6, 1709. CrossRef
15. a) L. F. Tietze, H. J. Schuster, B. Krewer, and I. Schuberth, J. Med. Chem., 2009, 52, 537; CrossRef b) L. F. Tietze, H. J. Schuster, J. M. von Hof, S. M. Hampel, J. F. Colunga, and M. John, Chem. Eur. J., 2010, 16, 12678. CrossRef
16. R. Appel, Angew. Chem., Int. Ed. Engl., 1975, 14, 801. CrossRef
17. G. S. Hari, Y. R Lee, X. Wang, W. S. Lyoo, and S. H. Kim, Bull. Korean. Chem. Soc., 2010, 31, 2406. CrossRef