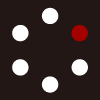
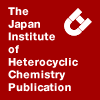
HETEROCYCLES
An International Journal for Reviews and Communications in Heterocyclic ChemistryWeb Edition ISSN: 1881-0942
Published online by The Japan Institute of Heterocyclic Chemistry
e-Journal
Full Text HTML
Received, 30th June, 2012, Accepted, 24th August, 2012, Published online, 10th September, 2012.
DOI: 10.3987/COM-12-S(N)82
■ Synthesis of Versatile Bifunctional Derivatives of Chiral Diamines Obtained through Anchimerically Assisted Nucleophilic Substitution Reactions on Diastereomeric Phenylprolinols
Jorge Vargas-Caporali, Carlos Cruz-Hernández, and Eusebio Juaristi*
Departamento de Química, Centro de Investigación y de Estudios Avanzados del Instituto Politécnico Nacional, A.P. 14-740, Avenida IPN 2508, Esquina Ticoman, C.P. 07000 México, D.F., Mexico
Abstract
Diastereomeric [(S)-1-benzylpyrrolidin-2-yl]-(R)-[(phenyl)methanamine] and [(S)-1-benzylpyrrolidin-2-yl]-(S)-[(phenyl)methanamine], were synthesized by selective internal backside nucleophilic substitution of the corresponding activated phenylprolinols. X-Ray diffraction structures of crystalline acetamide derivatives confirmed the anticipated stereochemistry for a SNib reaction mechanism. In order to apply this reaction to the synthesis of bifunctional analogs, a series of fragments such as a thiourea moiety and sulfonamide functions were introduced for the functionalization of the primary amino group in the substrate, obtaining more stable and potentially useful derivatives.INTRODUCTION
(N-Alkyl-pyrrolidin-2-yl)(phenyl)methanamine derivatives (Scheme 1) are useful pharmaceuticals in the treatment of diverse behavioural disorders such as: dementia, schizophrenia, and Parkinson’s disease.1
Furthermore, the development of synthetic methodologies to obtain this type of chiral diamines is important because of the promising structural features which could make these molecules helpful in asymmetric catalysis either as organocatalysts per se2 or as chiral ligands in coordination complexes used as catalysts.3
One of the principal routes for the preparation of β-aminopyrrolidines consists in the rearrangement reaction of piperidine or pyrrolidine derivatives via internal backside nucleophilic substitution (SNib).4 A salient feature of its mechanism is the anchimeric assistance of the heterocyclic nitrogen (Scheme 2).
This anchimeric mechanism is well known since 19485-7 and it was observed that strong nucleophiles such as amines are likely to favour formation of the pyrrolidinic framework, whereas weaker nucleophiles (−OH, −OAc) tend to generate mixtures of 5- and 6-membered ring products. Additionally, it is also worthy to mention that a similar behaviour is observed when linear β-aminoalcohols or polysubstituted pyrrolidinic β-aminoalcohols are used as starting materials in the Mitsunobu reaction.8
Nevertheless, the majority of recent studies have pursued the selective formation of six-membered derivatives,9 which exhibit biological activity as nonpeptidic NK-1 antagonists,10 anticancer drugs and anti-malarial agents.11 In this context, the enantioselective ring expansion of prolinols, has been intensively studied by J. Cossy and coworkers,12 whereas diverse methodologies have been developed to gain access to chiral piperidine derivatives13 and analogous nitrogenated heterocycles.14
On the other hand, few reports have focused in the development of enantioselective preparation of aminomethyl-pyrrolidine derivatives.8b,15 In this report we present our approach for the enantioselective synthesis of [(S)-1-benzylpyrrolidin-2-yl]-(R)-[(phenyl)methanamine] and [(S)-1-benzylpyrrolidin-2-yl]-(S)-[(phenyl)methanamine], (S,R)-1 and (S,S)-1, which are potential chiral building blocks for the preparation of new functionalized (S)-proline derivatives (Scheme 3).
RESULTS AND DISCUSSION
Synthesis of azide precursors via SNib reaction on chlorophenylpiperidines derived from enantiopure carbinols (S,R)- and (S,S)-7. Diastereomeric (N-benzylpyrrolidin-2-yl)(phenyl)methanols, (S,R)- and (S,S)-7 were prepared following a slightly modified synthetic methodology previously reported by Almansa et al.3a (Scheme 4). In particular, increased reaction times were used for the Swern oxidation,16 and the Grignard reaction was carried out at room temperature, and LiBr was used instead of CeCl3 to increase the reactivity of (unstable) aldehyde (S)-6.17 Commercially available phenylmagnesium bromide can be used although if prepared in situ, careful remotion of bromine (generated from PhBr) is necessary to avoid side reactions.18,19
The conversion of the hydroxy group in (S,R)- and (S,S)-7 into the desired amino group was accomplished by first reacting each amino alcohol with methanesulfonyl chloride and then carrying out the displacement reaction with azide, using NaN3 as the source of N-nucleophile. Specifically, the reaction between the amino alcohol (S,S)-7 and mesyl chloride afforded halogenated piperidine (R,R)-8, which is the product of ring expansion. The immediate internal nucleophilic attack from the pyrrolidinic nitrogen towards the carbon attached to the ephemeral methanesulfonyl group gives rise to the formation of the aziridinium ion, which is opened by a second addition of the chloride ion present in the reaction (Scheme 5). This result is in accordance with those observed with 1-ethylpyrrolidine-2-methanol and other analogs.7,12
Light sensitive piperidine (R,R)-8 was recrystallized to give suitable crystals for X-ray diffraction analysis, confirming the predicted relative configuration of both stereocenters (Figure 1).20
The chlorinated piperidine (R,R)-8 was subjected to nucleophilic substitution reaction conditions using a solution of sodium azide in DMSO-DMF, and irradiated with microwaves for two hours (40 Watts) in order to reduce the reaction time (ca. 8 h under normal heating conditions). Even though the desired pyrrolidinic derivative (S,S)-9 was obtained, the main product corresponded to (R,R)-10, originating from ring expansion (Scheme 6).
By contrast, amino alcohol (S,R)-7 gave rise to the analogous chloropiperidine (S,R)-8 as described previously by Langlois et al.9b However, it is important to point out that when (S,R)-8 reacted with NaN3 under the same conditions used with (R,R)-8, this conveniently afforded only the target azide (S,R)-9 (Scheme 7). The regioselective attack of the nucleophile can be explained by the development of a partial positive charge in the transition state, which seems to be more stabilized at the C–Ph atom (Scheme 7).21
The contrasting reactivity behaviour in (S,R)-8 and (R,R)-8 might be explained as follows: in the case of the aziridinium ion generated from chloropiperidine (S,R)-8, the bulky N-benzyl and phenyl groups are oriented to the same side (syn), which provokes steric repulsion that might induce an elongation and weakening of the N-C(Ph) bond. This effect facilitated the selective azide attack leading to the selective formation of pyrrolidinic product. By contrast, in the case of chloropiperidine (R,R)-8, the N-benzyl group is oriented anti to the phenyl group, so that no repulsive steric interaction between these groups takes place, and non-selective azide attack generates a mixture of the five and six-membered heterocyclic products.
Figure 2 compares the NMR spectra of azides (S,R)-9, (S,S)-9, and (R,R)-10, where the assignments were based on one- and two-dimensional NMR experiments. Salient observations are, (1) 3J1/5 = 3.72 Hz in (S,R)-9 is smaller than 3J1/5 = 7.45 Hz in (S,S)-9, which is similar to the trend observed in the corresponding starting amino alcohols (S,R)- and (S,S)-7. (2) Upfield shifts are observed for protons farther from the substituted carbons and for exocyclic methine proton H(5) in azide (S,S)-9, with respect to those of its (S,R)-9 diastereomer. (3) The methine proton H(1) in (S,S)-9 appears at lower field relative to the same proton in diastereomer (S,R)-9. (4) The difference in chemical shifts for the AB system in benzylic protons H(6) is largest in (R,R)-10 relative to both diastereomers (S,S)-9 and (S,R)-9.
Confirmation of the configurational assignment by means of X-Ray diffraction analysis.
Reduction of the azide function by means of catalytic hydrogenation afforded the diastereomeric N-benzylated diamines, (S,S)-1 and (S,R)-1, which were acetylated to give crystalline derivatives (S,S)-11 and (S,R)-11 (Scheme 8).
Figure 3 presents the structure and solid-state conformation of compounds (S,S)-11 and (S,R)-11. In these structures22,23 it is possible to confirm the anticipated configurations for the stereogenic centers in each diastereomer, allowing for the unambiguous conclusion that amino alcohol (S,R)-7 led to azide (S,R)-9 by a double nucleophilic attack (SN2ib) via the aziridinium ion intermediate from piperidine (S,R)-8. A similar conclusion can be reached for amino alcohol (S,S)-7, which gives rise to the corresponding azide (S,S)-9 with overall retention of configuration. The orientation of the hydrogen atoms bonded to the stereogenic carbons is also appreciated. For amide (S,S)-11 these hydrogens are oriented in opposite direction (anti) while in the diastereomer (S,R)-11 they are gauche-oriented.
Azido-piperidine (R,R)-10 was reduced according to the same procedure followed in the reduction of (S,S)-9 and (S,R)-9 affording diamine (R,R)-12, which was also acetylated to give crystalline amide (R,R)-13 (Scheme 9).
Figure 4 presents the structure and solid-state conformation of (R,R)-13 obtained by X-ray diffraction analysis. The cis configuration of the substituents at the stereogenic carbons is clearly appreciated, and this observation helps discard the possibility of a simple nucleophilic substitution in (R,R)-8, that would have resulted in inversion of the configuration at C(3).
Debenzylation reaction to obtain pharmacophore (R,R)-14.
Deprotection of diamine (R,R)-12 afforded derivative (R,R)-14, that offers an attractive strategy for the enantioselective preparation of various commercial drugs such as CP-122,721, CP-99994, among others, which are important neurokinin-1 receptor antagonists (Scheme 10).10b,13a
Conversion of the primary amino group into a thiourea moiety.
Chiral thioureas are important compounds due to its great versatility as Brønsted acid activators of prochiral substrates,25 chiral solvating agents in the enantiodiscrimination of carboxylic compounds,26 and more recently as catalysts in organocatalytic Mannich, aza-Henry, and Michael reactions (Figure 5).27
The conversion of primary amino group of derivatives (S,R)-1 and (S,S)-1 into a thiourea moiety was easily carried out by treatment of each diamine with phenyl isothiocyanate at room temperature, giving rise to the expected thioureas (S,R)-15 and (S,S)-15 (Scheme 11).28
Incorporation of a sulfonamide function at the amino group.
We also developed the synthesis of sulfonamide derivatives as described in Scheme 12, and it was possible to obtain a crystal structure of compound (S,R)-17, which is shown in Figure 6.
CONCLUSIONS
Pyrrolidinic diamines (S,R)-1 and (S,S)-1 were synthesized by anchimerically assisted nucleophilic substitution reaction (SNib) from carbinols (S,R)-7 and (S,S)-7, respectively. The overall retention of configuration could be confirmed by analysis of the NMR spectra of azides (S,R)-9 and (S,S)-9 and by examination of X-ray structures of crystalline derivatives (S,R)-11 and (S,S)-11.
The ring-expansion product (R,R)-10 was obtained solely from the diastereomeric carbinol (S,S)-7, as corroborated through the X-Ray crystallographic structure of its aminoamide derivative (R,R)-13. The relevant pharmacophore (R,R)-14 could be readily prepared from (R,R)-10.
Finally, a variety of bifunctional compounds with potential applications in asymmetric catalysis could be generated in good yields via the incorporation of thiourea and sulfonamide moieties in (S,R)-1 and (S,S)-1.
EXPERIMENTAL
General
Commercially available reagents were used as received. Anhydrous solvents were obtained by routine distillation techniques.30 TLC: Merck DC-F254 plates, detection UV light, iodine vapor. Flash chromatography: Merck silica gel (0.040–0.063 mm). Melting points: Melt Temp ‘Electrothermal’ apparatus, uncorrected. Optical rotations were measured in a Perkin-Elmer 241 polarimeter. NMR spectra were obtained with JEOL GSX-270 (270 MHz), Bruker Advance 300 (300 MHz), JEOL Eclipse 400 (400 MHz) and Jeol ECA-500 (500 MHz) spectrometers. Chemical shifts (δ) are given in parts per million relative to tetramethylsilane as internal reference, giving coupling constants J in hertz (Hz). IR spectra were recorded on a Varian Model 640 apparatus. Mass spectra were obtained in a Hewlett–Packard HP-5986 instrument 5989-AMS-ENGINE, Thermo Electron Trace-DSQ spectrometer, at 20 eV. High-resolution mass spectra (HRMS) were obtained on one HPLC 1100 coupled to MSDTOF Agilent Technologies apparatus mod. 1069A. Elemental analyses were obtained using a Thermo-Finnigan CHNS/O 1112 apparatus. The structural X-ray crystallographic data were obtained on an Enraf-Nonius Kappa CCD diffractometer. Microwave irradiation experiments were achieved in a single-mode Discover System reactor from CEM Corporation.
Synthesis of (S)-proline methyl ester hydrochloride, (S)-3. SOCl2 (76 mL, 1.04 mol) was added dropwise to a solution of (S)-proline (28.64 g, 0.25 mol) in MeOH (500 mL) at 0 °C. When the addition was complete, the mixture was refluxed for 2.5 h. After cooling to room temperature, the solvent was evaporated under reduced pressure. The resultant (S)-3 was obtained in 99% yield (42 g, 0.25 mol) as pale yellow oil and was used in the alkylation step without further purification. [α]D25 -34 (c 3.66, MeOH) {lit.,31 [α]D25-33.9 (c 3.55, MeOH)}; δH (D2O, 270 MHz): 2.35-2.52 (m, 3H); 2.71-2.78 (m, 1H); 3.64-3.88 (m, 3H); 4.16 (s, 3H). δC (D2O, 68 MHz): 23.8, 28.6, 47.0, 54.4, 60.2, 170.9. Spectroscopic data in accordance with those reported in lit.31
Synthesis of N-benzyl (S)-proline methyl ester, (S)-4. Et3N (77 mL, 55.9 g, 0.55 mol) was added dropwise to a cold solution of (S)-3 (41.2g, 0.25 mol) in dry CH2Cl2 under inert atmosphere. Then, benzyl bromide (33.2 mL, 47.74 g, 0.28 mol) was added to the thick reaction mixture, allowing it to reach room temperature after complete addition. This solution was stirred during 17 h and latter, it was extracted with CH2Cl2 (3 x 400 mL). The combined organic layers were dried over MgSO4. After filtration and evaporation of the solvents, the crude residue was purified by distillation under reduced pressure (120 °C, 0.5-1 mmHg), giving 49.6 g (0.23 mol) of the product, (S)-4, as a clear oil in 91% yield. Rf 0.7 (hexane:AcOEt, 7:3), [α]D25 –65 (c 3.0, CH2Cl2) {Aldrich, 97% ee, [α]D25–62.0 (neat)}, δH (CDCl3, 300 MHz): 1.71-1.79 (m, 1H); 1.88-2.0 (m, 2H); 2.06-2.17 (m, 1H); 2.39 (dd, 1H, J = 17 Hz); 3.0-3.08 (m, 1H); 3.25 (dd, 1H, J = 6.4Hz); 3.57 (d, 1H, J = 12.75 Hz); 3.64 (s, 3H); 3.89 (d, 1H, J = 12.75 Hz); 7.24-7.35 (m, 5H). δC (CDCl3, 75 MHz): 23.4, 29.8, 52.1, 53.7, 59.2, 65.7, 127.5, 128.6, 129.7, 138.5, 174.9. Chemical shifts in accordance with those reported in the literature.32
Reduction of amino ester (S)-4 to obtain N-benzyl (S)-prolinol, (S)-5. 25.75 g (0.68 mol) of LiAlH4 were slowly added via powder funnel to a cooled solution of (S)-4 (49.6 g, 0.23 mol) in anhydrous THF (400 mL) under N2. After stirring for one hour at 0 °C, the suspension was allowed to reach room temperature and then, it was refluxed during 3 h. The mixture was cooled again at 0 °C in order to quench the reaction. Quenching was carried out by carefully adding the cold content on 1.5 L of ice, this last in turn contained in a 4 L Erlenmeyer flask equipped with a cooling bath. Once the frost has melted, the resulting magnesia is poured onto a celite pad to remove suspended alkalis and the solid residue was washed with 300 mL of hot AcOEt. The resultant biphasic filtrate was separated and THF was removed from the aqueous layer in a rotary evaporator, extracting again the residual liquid previously cooled with a similar amount of AcOEt. The combined organic layers were dried with Na2SO4 and concentrated, and the crude was distilled under reduced pressure (110 °C, 1.5 mmHg), obtaining 41.8 g (0.22 mol) of the desired amino alcohol (S)-5 as a colourless liquid in 97% yield. Rf 0.4 (hexane:AcOEt, 7:3), [α]D25 -60 (c 1.0, CHCl3) {lit.,33 [α]D25 -59.9 (c 1.0, CHCl3)}, δH (CDCl3, 500 MHz): 1.60-1.69 (m, 2H); 1.78 (sext., 1H, J = 6.5 Hz); 1.84-1.92 (m, 1H); 2.23 (dd, 1H, J = 0.95 Hz, 8.45 Hz); 2.64-2.59 (m, 1H); 2.92 (quint., 1H, J = 4.7 Hz); 3.26 (br, 1H); 3.31 (d, 1H, J = 12.95 Hz); 3.45 (dd, 1H, J = 2.65 Hz, 10.8 Hz); 3.59 (dd, 1H, J = 3Hz, 10.75 Hz); 3.95 (d, 1H, J = 12.95 Hz); 7.18-7.23 (m, 1 H); 7.27 (d, 4H, J = 4.05 Hz). δH (CDCl3, 125 MHz): 23.45, 27.94, 54.57, 58.86, 62.41, 64.56, 127.13, 128.53, 128.91, 139.40. IR (ATR, cm-1): νmax 3420, 3060, 3026, 2960, 2872, 2796, 1738, 1602, 1494, 1452, 1374, 1352, 1250, 1210, 1076, 1030, 912, 848, 740, 700. Spectroscopic data in accordance with those reported in the literature.34
Swern Oxidation of N-benzylprolinol (S)-5 to the corresponding aldehyde, (S)-6. 21.05 mL (31.56 g, 0.25 mol) of oxalyl chloride were dissolved with 600 mL of dry CH2Cl2 under nitrogen atmosphere in a 2-L three-necked round bottomed flask equipped with magnetic stirring, two addition funnels and two or three evolving gas traps. The solution was cooled at -78 °C and then, 38.6 mL of DMSO (42.4 g, 0.54 mol) were added dropwise, allowing time for the system to depressurise the immediately evolved CO2. After completion of addition, the reaction mixture was stirred during one hour. Then, 41.8 g (0.22 mol) of (S)-5 dissolved in 100 mL of dry CH2Cl2 were added over a period of 20 min. The resulting thick mixture was left under these conditions for 3 h more and latter, Et3N (142 mL, 103 g, 1.02 mol) were added leaving the reaction mixture to reach 0 °C and stirring during 3 h at this temperature. Then, 300 mL of brine were added and the organic layer was washed thrice to remove the maximum amount of Me2S. The organic layer was dried over Na2SO4 and after filtration and evaporation of the solvent; the resultant crude aldehyde (S)-6 was dried under vacuum for a prolonged period. Then, 39 g of aldehyde (S)-6 were obtained (94.2% yield) as a crude orange oil which was used in the next step without further purification due to quick decomposition. Rf 0.5 (hexane/AcOEt, 7:3), δH (CDCl3, 270 MHz): 1.79-1.91 (m, 4H); 2.34-2.44 (m, 1H); 2.95-3.02 (m, 1H); 3.06-3.15 (m, 1H); 3.65 (d, 1H, J = 12.9); 3.75 (d, 1H, J = 12.9); 7.22-7.35 (m, 5H); 9.3 (dd, 1H, J = 3.7 Hz, 0.78 Hz). δC (CDCl3, 68 MHz): 23.47, 26.51, 53.99, 59.44, 71.55, 127.34, 128.82, 129.08, 138.31, 202.75. HR-ESI-TOF [M+H]+ (m/z) calculated for C12H16NO: 190.1226; found: 190.1226, (error = -0.2141). Chemical shifts in accordance with those reported in the literature.35
Grignard reaction to obtain the mixture of diastereomeric amino alcohols (S,R)- and (S,S)-7. 27.5 g (1.13 mol) of clean Mg and 200 mL of anhydrous THF were added in a 1-L round-bottomed flask equipped with a magnetic stir bar and conditioned with inert atmosphere. Afterwards, 119 mL (177 g, 1.13 mol) of freshly distilled bromobenzene were added dropwise to the cooled heterogeneous mixture. Once the content became homogeneous, 17.9 g (0.21 mol) of LiBr dissolved in 100 mL of anhydrous THF were added at 0 °C to the Grignard solution and stirred during 1 h at this temperature. Then, 39 g (0.21 mol) of (S)-6 dissolved in dry THF (100 mL) were added via cannula and the final solution was stirred during 4 h at 0 °C and left to reach room temperature overnight. The reaction was quenched by adding in cold 200 mL of a saturated aqueous solution of NH4Cl. When Grignard reagent was eliminated, filtering and washing of solid precipitates, and extraction of residual liquids were carried out following the same techniques described above for (S)-5. AcOEt was evaporated under vacuum obtaining the diastereomeric mixture crude. Amino alcohols were separated by column chromatography using gradient (hexane:AcOEt, 95:5→85:15), obtaining 23.71 g (0.09 mol) of (S,R)-7 and 21.9 g (0.08 mol) of (S,S)-7 (d. r. 52:48, 81% overall yield).
(R)-[(2S)-1-Benzylpyrrolidin-2-yl](phenyl)methanol, (S,R)-7. Yellow oil; Rf 0.55 (hexane/AcOEt, 7:3); [α]D25 –69 (c 1.16, CHCl3) {lit.,3a [α]D25 –69.2 (c 1.3, CHCl3)}, δH (CDCl3, 400 MHz): 1.28-1.43, 1.6–1.83 (1H and 3H, respectively, 2m, CH2CH2CH), 2.36 (1H, q, J = 8.65 Hz, 1 x CH2CHHN), 2.89–2.98 (1H, m, CH-CHOH), 3.03–3.14 (1H, m, 1 x CH2CHHN), 3.5, 4.22 (1H each, 2d, J = 12.88 Hz each, CH2Ph), 4.93 (1H, d, J = 2.92 Hz, CH-OH), 7.34–7.59 (10H, m, ArH); δC (CDCl3, 100 MHz): 23.3, 24.1 (CH2CH2CH), 54.81 (CH2CH2N), 58.36 (NCH2Ph), 69.28 (CH2CHN), 70.22 (CH-OH), Ar: 125.61, 126.9, 127.31, 128.24, 128.55, 128.91, 139.11 (C-ipso), 141.63 (C-ipso). Chemical shifts and other spectroscopic data are in agreement with those reported in the literature.3a
(S)-[(2S)-1-Benzylpyrrolidin-2-yl](phenyl)methanol, (S,S)-7. White solid, mp 72-74 °C (AcOEt/ hexane); Rf 0.33 (hexane/AcOEt, 7:3); [α]D25 +118.3 (c 1.04, CHCl3) {lit.,3a [α]D25 +101.8 (c 1.1, CHCl3)}, δH (CDCl3, 270 MHz): 1.71–1.84, 1.91–2.04 (3H and 1H, respectively, 2m, CH2CH2CH), 2.36–2.48, 2.92–3.01 (1H each, 2m, CH2CH2N), 3.05–3.14 (1H, m, -CHN), 3.35, 3.66 (1H each, 2d, J = 12.9 Hz each, N-CH2Ph), 4.39 (1H, d, J = 5.2 Hz, *CH-OH), 7.20–7.41 (10H, m, ArH); δC (CDCl3, 68 MHz): 24.26, 29.33 (CH2CH2CH), 54.22 (CH2CH2N), 61.19 (NCH2Ph), 70.15 (CH2CHN), 75.19 (CH-OH), Ar: 126.12, 127.03, 127.10, 128.24, 128.32, 128.65, 139.40 (C-ipso), 143.69 (C-ipso). Chemical shifts and other spectroscopic data are in agreement with those reported in the literature.3a
General procedure for the synthesis of 1-benzyl-3-chloro-2-phenylpiperidines. A determined amount of the required diastereomer 7 was dissolved with CH2Cl2 in a three-necked round-bottomed flask equipped with magnetic stirring and conditioned with nitrogen atmosphere. Subsequently, 1.6 equivalents of Et3N and 1.5 equivalentes of MeSO2Cl are simultaneously added at 0 °C. The reaction mixture was left stirring during three more hours at this temperature. After this time, the reaction was ended by extracting twice with saturated aqueous solution of NaHCO3 and latter with brine, then the organic layer was dried with Na2SO4. Activated charcoal is incorporated to the organic phase and the mixture was slightly heated before pouring onto a compressed celite pad in a porous glass filter. Solvent was evaporated under reduced pressure and the resultant oil is dissolved again in a minimum volume of heated hexane:AcOEt (95:5) mixture. The saturated solution is cooled thus precipitating colourless crystals which were filtrated and dried under vacuum.
(2R,3R)-1-Benzyl-3-chloro-2-phenylpiperidine, (R,R)-8. The general procedure described above was followed with 5.5 g (0.02 mol) of (S,S)-8, 4.6 mL (3.34 g, 0.03 mol) of Et3N and 2.4 mL (3.55 g, 0.03 mol) of MeSO2Cl and 100 mL of CH2Cl2 to afford the desired piperidine (R,R)-8 (5.8 g, 98.4% yield), as a colourless solid, mp 94-96 °C (AcOEt/hexane); Rf 0.58 (hexane/AcOEt, 9:1); [α]D25 +20 (c 1.07, CHCl3), δH (CDCl3, 500 MHz): 1.48-1.55, (m, 1H, CH2-CH2-CH2-), 1.96 (tdd, 1H, J = 13.5, 3.5, 3.2 Hz, -CHCl-CH2-), 2.04 (td, 1H, J = 12.0, 3.2 Hz, -CH2-N), 2.14-2.25 (m, 2H, -CHCl-CH2-CH2-), 2.93 (d, 1H, J = 14.0 Hz, NCH2Ph), 3.06 (ddd, 1H, J = 12.0, 5.0, 3.2 Hz, -CH2-CH2-N), 3.54 (d, 1H, J = 2.2, -CHPh-CHCl-), 3.93 (d, 1H, J =14.0 Hz, NCH2Ph), 4.22 (ddd, 1H, J = 3.2, 2.2, 1.7 Hz, CHPh-CHCl ), 7.18-7.37 (m, 8H, ArH), 7.46-7.51 (m, 2H, Ar). δC (CDCl3, 125 MHz): 20.07 (CH2-CH2-CH2-), 33.56 (-CHCl-CH2-), 53.28 (CH2-N), 59.17 (NCH2Ph), 64.00 (-CHCl-), 71.38 (-CHPh-CHCl-), ArC: 126.73, 127.57, 127.93, 128.14, 128.57, 129.00, 138.85 (NCH2Ph, Cipso), 140.52 (-CHPh-CHCl-, Cipso). IR (ATR, cm-1): νmax 3060, 3023, 2954, 2929, 2804, 1600, 1492, 1449, 1315, 1304, 1133, 1099, 1065, 1028, 996, 941, 885, 755, 737, 703, 640, 600. HR-ESI-TOF [M+H]+ calculated for C18H21ClN: 286.1357; found: 286.1357 (error = -0.0139).
(2S,3R)-1-Benzyl-3-chloro-2-phenylpiperidine, (S,R)-8. The general procedure described for the synthesis of piperidines was followed with 4.0 g (0.02 mol) of (S,R)-8, 3.34 mL (2.43 g, 0.02 mol) of Et3N and 1.74 mL (2.58 g, 0.02 mol) of MeSO2Cl and 80 mL of CH2Cl2 to afford the desired piperidine (S,R)-8 (4.27 g, 99% yield), as a colourless solid, mp 60-61 °C (AcOEt/hexane); Rf 0.67 (hexane/AcOEt, 9:1); [α]D25 +25 (c 1.0, CHCl3), {lit.,9b [α]D298 +27(c 1.6, CHCl3)}, δH (CDCl3, 500 MHz): 1.63-1.81 (m, 3H); 2.00-2.06 (m, 1H); 2.35-2.40 (m, 1H); 2.87 (d, 1H, J = 13.5 Hz); 2.93 (m, 1H); 3.19 (d, 1H, J = 9.55 Hz); 3.68 (d, 1H, J = 13.5 Hz); 3.96 (ddd, 1H, J = 11.55, 9.55, 4.5 Hz); 7.18-7.49 (m, 10H, ArH). δC (CDCl3, 125 MHz): 25.41, 36.38, 52.23, 59.50, 63.66, 75.64, 126.85, 127.93, 128.19, 128.45, 128.58, 139.38, 141.44. HR-ESI-TOF [M+H]+ calculated for C18H21ClN: 286.1357; found: 286.1358 (error = 0.3559). Chemical shifts are in agreement with those reported in the literature.9b
General procedure for the nucleophilic substitution reaction employing NaN3. A determined amount of the required piperidine 8 were dissolved in 10-20 mL of DMF, and added to a 100-mL long-necked flask (special for MW equipment use) containing 1.1 equiv of NaN3 previously homogenized in 30-40 mL of DMF:DMSO (9:1). This mixture was stirred and later, the flask was placed inside the microwave equipment to irradiate the reaction with 40 Watts of power in order to heat at 55 °C. The reaction was irradiated during 2 h under controlled temperature by using simultaneous external cooling with compressed air. At the end of this period, the reaction was cooled to room temperature and subsequently added to a flask with 100 mL of brine at 0 °C to facilitate the extraction with petroleum ether (3 x 50 mL). The combined organic layers were washed with 30 mL of water to remove residual polar solvents, dried with Na2SO4 and concentrated in the rotary evaporator. The crude was dried under reduced pressure and purified by flash chromatography (2% AcOEt in hexane) to give the corresponding azides.
(S)-2-[(R)-Azido(phenyl)methyl]-1-benzylpyrrolidine, (S,R)-9. The general procedure described above was followed with 2.54 g (9 mmol) of chloropiperidine (S,R)-8, 0.64 g (10 mmol) of NaN3 and 50 mL of DMF:DMSO (4:1) to afford 2.3 g (8 mmol) of the desired azide (S,R)-9 as a clear oil in 89 % yield, Rf 0.59 (hexane:AcOEt, 9:1), [α]D25 –97 (c 1.0, CHCl3), δH (CDCl3, 500 MHz): 1.55-1.69 (m, 2H, CH2CH2CH), 1.71-1.84 (m, 1H, CH2CH2CH), 1.87-1.96 (m, 1H, CH2CH2CH), 2.25 (ddd, 1H, J = 9.9, 9.35, 6.3 Hz, CH2CH2N), 2.92 (ddd, 1H, J = 8.8, 4.65, 4.1 Hz, NCHCH2), 3.03 (ddd, 1H, J = 9.2, 7.1, 2.1 Hz, CH2CH2N), 3.48, 3.93 (d, 1H each, J = 12.89 Hz, NCH2Ph), 4.57 (d, 1H, J = 4.1 Hz, CHCHN3), 7.21-7.35 (m, 10H, ArH); δC (CDCl3, 125 MHz): 23.59 (CH2-CH2-CH2-), 26.00 (CH2CH2CH), 54.86 (CH2CH2N), 59.71 (NCH2Ph), 67.2 [CH(Ph)N3], 69.65 (NCHCH2), ArC: 126.87, 126.98, 127.56, 128.26, 128.36, 128.81, 138.46 (NCH2Ph, Cipso), 139.40 [CH(Ph)N3, Cipso]. IR (ATR, cm-1): νmax 2967, 2796, 2097 (N3), 1495, 1452, 1351, 1288, 1269, 1122, 1074, 1029, 846, 740, 697. MS (IE) m/z (%): 65.1 (22.96), 77.1 (8.88), 89.1 (8.2), 90.1 (8.88), 91.1 (100), 92.1 (9.62), 104.1 (13.7), 118.1 (25.06), 146 (11.38), 160.2 (29.52), 194.2 (10.52), 236.3 (10.6). HR-ESI-TOF [M+H]+ (m/z) calculated for C18H21N4: 293.1760; found: 293.1764, (error = 1.1143).
(S)-2-[(S)-Azido(phenyl)methyl]-1-benzylpyrrolidine, (S,S)-9, and (2R,3R)-3-azido-1-benzyl-2- phenyl piperidine, (R,R)-10. The general procedure described above was followed with 2.73 g (9.6 mmol) of chloropiperidine (R,R)-8, 0.64 g (10.5 mmol) of NaN3 and 50 mL of DMF:DMSO (4:1) to obtain 0.86 g (3 mmol, 31% yield) of the desired azide (S,S)-9 and 1.5 g (5.3 mmol, 55% yield) of azidopiperidine (R,R)-10 both as colourless oils.
(S)-2-[(S)-Azido(phenyl)methyl]-1-benzylpyrrolidine, (S,S)-9. Rf 0.41 (hexane:AcOEt, 9:1), [α]D25 +84 (c 1.0, CHCl3). δH (CDCl3, 500 MHz): 1.39-1.52 (m, 2H, one for each CH2CH2CH), 1.53-1.61 (m, 1H, CH2CH2CH), 1.61-1.72 (m, 1H, CH2CH2CH), 2.30 (td, 1H, J = 9.42, 6.3 Hz, CH2CH2N), 2.91 (ddd, 1H, J = 10.0, 6.52, 2.97 Hz, CH2CH2N), 3.07 (ddd, 1H, J = 8.95, 7.45, 3.94 Hz, NCHCH2), 3.53, 4.31 (d, 1H each, J = 13.03 Hz, NCH2Ph), 4.39 (d, 1H, J = 7.45 Hz, CHCHN3), 7.22-7.43 (m, 10H, ArH); δC (CDCl3, 125 MHz): 23.72 (CH2-CH2-CH2-), 28.51 (CH2CH2CH), 54.70 (CH2CH2N), 61.02 (NCH2Ph), 67.43 [CH(Ph)N3], 71.19 (NCHCH2), ArC: 126.81, 127.84, 128.02, 128.22, 128.39, 128.83, 138.16 (NCH2Ph, Cipso), 139.82 [CH(Ph)N3, Cipso]. IR (ATR, cm-1): νmax 3032, 2970, 2939, 2866, 2796, 2090 (N3), 1493, 1453, 1343, 1275, 1249, 1098, 1027, 917, 870, 818, 751, 699, 657, 606. MS (EI) m/z (%): 65.1 (13.66), 91.0 (100), 160.2 (70.71), 161.2 (9.31). HR-ESI-TOF [M+H]+ calculated for C18H21N4: 293.1760; found: 293.1767, (error = 2.1376).
(2R,3R)-3-Azido-1-benzyl-2-phenyl piperidine, (R,R)-10. Rf 0.54 (hexane:AcOEt, 9:1), [α]D25 -36.7 (c 1.09, CHCl3). δH (CDCl3, 500 MHz): 1.47-1.54 (m, 1H, CH2CH2CH2), 1.72 (tdd, 1H, J = 13.39, 4.01, 3.22 Hz, -CH2CH2CHN3), 1.91 (tt, 1H, CH2CH2CH, J = 12.82, 3.46 Hz), 1.99 (ddd, 1H, J = 12.17, 11.46, 2.46 Hz, CH2CH2N), 2.11 (dddd, 1H, J = 13.69, 6.17, 3.22, 1.63 Hz, CH2CH2N), 2.88 (d, 1H, J = 13.75 Hz, NCH2Ph), 3.03 (dtd, 1H, J = 11.01, 3.03, 1.43 Hz, CH2CH2N), 3.41 [d, 1H, J = 2.43, NCH(Ph)CH(N3)], 3.62 [dd, 1H, J = 5.87, 3.01 Hz, CH(Ph)CH(N3)], 13.88 (d, 1H, J = 13.75 Hz, NCH2Ph), ArH: 7.18-7.23 (m, 1H), 7.24-7.31 (m, 5H), 7.33-7.39 (m, 2H), 7.47-7.54 (2H); δC (CDCl3, 125 MHz): 20.48 (CH2-CH2-CH2-), 29.83 (CH2CH2CHN3), 52.95 (CH2CH2N), 59.48 (NCH2Ph), 63.40 [CH(Ph)CH(N3)], 70.75 [NCH(Ph)CH(N3)], ArC: 126.71, 127.58, 128.10, 128.37, 128.56, 128.59, 138.63 (NCH2Ph, Cipso), 140.43 [CH(Ph)CH(N3), Cipso]. IR (ATR, cm-1): νmax 3858.0, 3740, 3618, 3028, 2942, 2792, 2360, 2104 (N3), 1700, 1650, 1494, 1452, 1332, 1268, 1132, 1070, 1032, 962, 898, 744, 702. MS (EI) m/z (%): 65.1 (24.31), 91.1 (100), 104.1 (13.76), 118.1 (25.34), 146.2 (10.39),160.2 (29.13), 194.2 (10.24), 236.3 (9.67). HR-ESI-TOF [M+H]+ calculated for C18H21N4: 293.1760; found: 293.1762, (error = 0.4321).
General procedure for the catalytic hydrogenation of azido group. A determined amount of the required azide 9 was dissolved in 50 mL of EtOH in a PYREX® heavy wall centrifuge bottle which was conditioned with inert atmosphere. Subsequently, 10 % w/w of palladium on charcoal (1 %) was added to the solution, the recipient was placed in the hydrogenator and then, the system was purged twice with hydrogen pressure. Finally 60-70 psi were charged leaving the reaction mixture under mechanical stirring during 2-5 h. The reaction was monitored by TLC and once the initial azide is depleted, the mixture is poured onto a celite pad to remove the catalyst and the filtrate was concentrated under vacuum. The crude was purified with column chromatography, using CH2Cl2:AcOEt (9:1) as eluent, obtaining the corresponding diamine.
(R)-[(S)-1-Benzylpyrrolidin-2-yl](phenyl)methanamine, (S,R)-1. The general procedure described above was used with 2.48 g (9.31 mmol) of azide (S,R)-9 and 0.25 g of Pd/C (1% w/w) suspended in 50 mL of EtOH under 60 psi of H2 pressure during 3 h. The diamine (S,R)-1 was obtained after chromatography with 90% yield as a yellow oil, improved purification could be achieved by Kugelrohr distillation (150 °C, 0.5-1 mmHg). Rf 0.63 (CH2Cl2:AcOEt, 9:1), [α]D25 –44 (c 1.05, CHCl3), δH (CDCl3, 500 MHz): 1.36 (ddd, 1H, J = 17.54, 12.9, 8.73 Hz, CH2CH2CH), 1.54-1.7 (m, 2H, CH2CH2CH), 1.70-1.84 (b, 2H, NH2), 1.89 (dddd, 1H, J = 12.9, 9.1, 6.38, 4.1 Hz, one for CH2CH2CH), 2.20 (td, J = 9.52, 7.2 Hz, CH2CH2N), 2.77 (ddd, 1H, J = 9.24, 6.23, 3.44 Hz, NCHCH2), 3.00 (ddd, 1H, J = 8.99, 6.84, 2.29 Hz, CH2CH2N), 3.38, 4.10 (d, 2 X 1H, J = 13.1 Hz, NCH2Ph), 4.24 [d, 1H, J = 3.29 Hz, CHCH(Ph)NH2], 7.19-7.27 (m, 2H, ArH), 7.29-7.37 (m, 6H, ArH), 7.38-7.42 (m, 2H, ArH). (CDCl3, 125 MHz): 23.46 (CH2-CH2-CH2-), 23.97 (CH2CH2CH), 54.77 [CHCH(Ph)NH2], 55.06 (CH2CH2N), 59.02 (NCH2Ph), 69.97 (NCHCH2), ArC: 126.75, 126.81, 126.99, 128.27, 128.39, 128.75, 140.00 (NCH2Ph, Cipso), 143.69 [CH(Ph)NH2, Cipso]. IR (ATR, cm-1): νmax 3025, 2961, 2871, 2787, 1681, 1602, 1494, 1451, 1373, 1290, 1207, 1118, 1071, 1028, 912, 873, 736, 697, 648. HR-ESI-TOF [M+H]+ calculated for C18H23N2: 267.3881; found: 267.1855, (error = 0.8407).
(S)-[(S)-1-Benzylpyrrolidin-2-yl](phenyl)methanamine, (S,S)-1. The general procedure described above was used with 3.66 g (13.41 mmol) of azide (S,S)-9 and 0.37 g of Pd/C (1% w/w) suspended in 100 mL of EtOH under 60 psi of H2 pressure during 2 h. The diamine (S,S)-1 was obtained after chromatography with 97% yield as a yellow oil. Rf 0.68 (CH2Cl2:AcOEt, 9:1), [α]D25 +41 (c 1.0, CHCl3), δH (CDCl3, 500 MHz): 1.54-1.7 (m, 3H, CH2CH2CH), 1.73-1.81 (m, 1H one for CH2CH2CH), 1.82-1.92 (b, 2H, NH2), 2.31 (td, J = 9.16, 6.35 Hz, CH2CH2N), 2.92 (ddd, 1H, J = 9.99, 6.34, 3.62 Hz, NCHCH2), 3.01 (ddd, 1H, J = 9.02, 6.45, 2.86 Hz, CH2CH2N), 3.44 (d, 1H, J = 13.32 Hz, NCH2Ph), 3.84 [d, 1H, J = 6.59 Hz, CHCH(Ph)NH2], 3.97 (d, 1H, J = 13.32 Hz, NCH2Ph), 7.2-7.27 (m, 2H, ArH), 7.28-7.37 (m, 6H, ArH), 7.37-7.42 (m, 2H, ArH). (CDCl3, 125 MHz): 24.33 (CH2-CH2-CH2-), 28.62 (CH2CH2CH), 54.74 [CHCH(Ph)NH2], 60.54 (CH2CH2N), 61.83 (NCH2Ph), 70.35 (NCHCH2), ArC: 126.71, 126.88, 127.42, 128.13, 128.19, 128.50, 140.37 (NCH2Ph, Cipso), 144.55 [CH(Ph)NH2, Cipso]. IR (ATR, cm-1): νmax IR (cm-1): 3060, 3025, 2964, 2870, 2787, 1663, 1601, 1493, 1451, 1352, 1122, 1069, 1028, 910, 870, 737, 697, 673, 597, 568. HR-ESI-TOF [M+H]+ calculated for C18H23N2: 267.3881; found: 267.1857, (error = 0.4665).
(2R,3R)-1-Benzyl-2-phenylpiperidin-3-amine, (R,R)-12. The general procedure described above was used with 4.67 g (15.8 mmol) of azide (R,R)-10 and 0.47 g of Pd/C (1% w/w) suspended in 100 mL of EtOH under 60 psi of H2 pressure overnight. The diamine (R,R)-12 was obtained after chromatography with 97% yield as a yellow oil (15.3 mmol, 4.08 g). δH (CDCl3, 500 MHz): 1.45-1.52 (m, 1H, CH2CH2CH2CH), 1.58 (br, 2H, NH2), 1.69-1.87 (m, 2H, CH2CH2CH), 1,90-2.02 (m, 2H, CH2CH2CH2CH), 2.83-2.88 (m, 1H, CH2CH2CH), 2.85 (d, 1H, J = 13.92 Hz, NCH2Ph), 3.00 (dt, 1H, J = 11.54, 4.00 Hz, CH2CH2CH2CH), 3.40 (d, 1H, J = 2.32 Hz, CHCHNBn), 3.93 (d, 1H, J = 13.92 Hz, NCH2Ph), 7.18-7.45 (m, 10H, ArH); δC (CDCl3, 125 MHz): 19.95 (CH2CH2CH2CH), 32.24 (CH2CH2CH2CH), 51.98 (CH2CH2CHNH2), 53.67 (CH2CH2CH2CH), 59.68 (NCH2Ph), 72.62 (CHCHNBn) ArC: 126.61, 126.97, 128.10, 128.17, 128.32, 128.38, 139.45 (NCH2Ph, Cipso), 142.01 [CH(Ph)CH, Cipso]. HR-ESI-TOF [M+H]+ calculated for C18H23N2: 267.1855; found: 267.1856, (error = 0.0922).
General procedure for the acetylation reaction. A determined amount of the required diamine was dissolved with anhydrous THF in a round-bottomed flask equipped with magnetic stirring and conditioned with nitrogen atmosphere. Subsequently, 1.3 equivalents of Et3N and 1.2 equivalents of MeCOCl were simultaneously added at 0 °C. Then, the reaction mixture was left stirring during 48 h at room temperature. After this time, the reaction was concentrated and the crude was extracted twice with brine and AcOEt. Subsequently, the organic layer was dried with Na2SO4, the solvent was evaporated under reduced pressure and the resultant concentrate is supported on silica and purified using CH2Cl2:AcOEt (9:1) as eluent in flash chromatography, obtaining the corresponding acetamides.
N-{(R)-[(S)-1-Benzylpyrrolidin-2-yl](phenyl)methyl}acetamide, (S,R)-11. The general procedure which was described above was followed using 2.13 g (8 mmol) of (S,R)-1, 1.5 mL (1.05 g, 10.4 mmol) of Et3N and 0.7 mL (0.75 g, 9.6 mmol) of MeCOCl and 30 mL of THF to obtain the acetamide (S,R)-11 in 62 % of isolated yield after recrystallization, as a white solid, mp 180-181 °C (AcOEt/hexane); Rf 0.48 (CH2Cl2/MeOH, 9:1); [α]D25 –62 (c 1.0, CHCl3), δH (CDCl3, 500 MHz): 1.36-1.48 (m, 1H, CH2CH2CH), 1.48-1.62 (m, 3H, CH2CH2CH), 2.05 (s, 3H, CH3CO), 2.23 (td, 1H, J = 9.04, 8.45 Hz, CH2CH2N), 2.88 (td, 1H, J = 7.59, 4.96 Hz, CH2CHN), 2.94 (ddd, 1H, J = 9.54, 7.11, 2.63 Hz, CH2CH2N), 3.28 (d, 1H, J = 12.82 Hz, NCH2Ph), 4.04 (d, 1H, J = 12.89 Hz, NCH2Ph), 5.07 (t, 1H, J = 5.12 Hz, CHCHNHAc), 6.35 (d, 1H, J = 4.15 Hz, NH), 7.18-7.35 (m, 10H, ArH); δC (CDCl3, 125 MHz): 21.78 (COCH3), 23.41 (CH2-CH2-CH2-), 26.00 (CH2CH2CH), 53.54 (CH2CH2N), 54.18 [CH(Ph)NHAc], 58.25 (NCH2Ph), 67.26 (NCHCH2), ArC: 126.29, 126.84, 127.03, 128.22, 128.30, 128.73, 139.04 (NCH2Ph, Cipso), 140.24 [CH(Ph)NHAc, Cipso], 170.35 [NH(C=O)CH3]. IR (ATR, cm-1): νmax 3323, 2793, 1640, 1550, 1455, 1132, 1029, 978, 845, 739, 696, 644, 581. MS (IE) m/z (%): 91.1 (42), 160.05 (100), 161.05 (11). HR-ESI-TOF [M+H]+ (m/z) calculated for C20H25N2O: 309.1961; found: 309.1968, (error = 2.1343).
N-{(S)-[(S)-1-Benzylpyrrolidin-2-yl](phenyl)methyl}acetamide, (S,S)-11. The general procedure which was described above was followed using 1.15 g (4.3 mmol) of (S,R)-1, 0.8 mL (0.57 g, 5.6 mmol) of Et3N and 0.37 mL (0.41 g, 5.2 mmol) of MeCOCl and 15 mL of THF to obtain the acetamide (S,R)-11 in 90% of yield, as a white solid, mp 136 °C (AcOEt/hexane); Rf 0.53 (CH2Cl2/MeOH, 9:1); [α]D25+76 (c 1.0, CHCl3), δH (CDCl3, 500 MHz): 1.58-1.72 (m, 2H, CH2CH2CH), 1.72-1.78 (m, 1H, CH2CH2CH), 2.07-2.13 (m, 1H, CH2CH2CH), 2.13 (s, 3H, CH3CO), 2.24 (td, 1H, J = 9.85, 6.35 Hz, CH2CH2N), 2.90 (ddd, 1H, J = 9.20, 6.77, 2.36 Hz, CH2CH2N), 2.97 (d, 1H, J = 13.03 Hz, NCH2Ph), 3.19 (ddd, 1H, J = 9.49, 4.77, 2.11 Hz, NCHCH2), 3.26 (d, 1H, J = 12.89 Hz, NCH2Ph), 5.06 (dd, 1H, J = 8.31, 2.0 Hz, CHCHNHCOCH3), 6.85 (d, 1H, J = 8.02 Hz, NH), 7.09-7.39 (m, 10H, ArH); δC (CDCl3, 125 MHz): 23.50 (COCH3), 24.17 (CH2-CH2-CH2-), 30.61 (CH2CH2CH), 55.25 (CH2CH2N), 55.74 [CH(Ph)NHCOCH3], 60.52 (NCH2Ph), 67.74 (NCHCH2), ArC: 125.94, 126.82, 126.85, 128.16, 128.39, 128.56, 139.53 (NCH2Ph, Cipso), 141.46 [CH(Ph)NHAc, Cipso], 169.84 [NH(C=O)CH3]. IR (ATR, cm-1): 3337, 2970, 2801, 1646, 1534, 1373, 1295, 1140, 1111, 744, 700, 627. HR-ESI-TOF [M+H]+ (m/z) calculated for C20H25N2O: 309.1961; found: 309.1963, (error = 0.5172).
N-[(2R,3R)-1-Benzyl-2-phenylpiperidin-3-yl]acetamide, (R,R)-13. The general procedure which was described above was followed using 4.1 g (15.3 mmol) of (R,R)-12, 2.8 mL (2.02 g, 20 mmol) of Et3N and 1.3 mL (1.44 g, 18.4 mmol) of MeCOCl and 100 mL of THF to obtain the acetamide (S,R)-11 in 79% of isolated yield after recrystallization, as a white solid, mp 160-161 °C (AcOEt/hexane); Rf 0.61 (CH2Cl2/MeOH, 9:1); [α]D25 +3.3 (c 1.0, CHCl3). δH (CDCl3, 500 MHz): 1.51-1.58 (m, 1H, CH2CH2CH2CH), 1.59-1.80 (m, 2H, CH2CH2CH), 1.86 (s, 3H, CH3CO), 1.92-1.98 (m, 1H, CH2CH2CH), 2.02 (ddd, 1H, J = 11.89, 11.86, 2.94 Hz, CH2CH2CH2), 2.87 (d, 1H, J = 13.89 Hz, NCH2Ph), 3.02 (ddd, 1H, J = 11.81, 3.97, 3.94 Hz, CH2CH2CH2), 3.46 (d, 1H, J = 2.58 Hz, CHCHNBn), 3.95 (d, 1H, J = 13.89 Hz, NCH2Ph), 4.14 (dd, 1H, J = 8.88, 2.72 Hz, CH2CHNHAc), 6.30 (d, 1H, J = 8.73 Hz, NH), 7.19-7.40 (m, 10H, ArH); δC (CDCl3, 125 MHz): 20.60 (COCH3), 23.28 (CH2-CH2-CH2-), 29.77 (CH2CH2CH), 50.12 (CH2CH2N), 53.35 [CH(Ph)NHCOCH3], 59.36 (NCH2Ph), 70.45 (NCHCH2), ArC: 126.87, 127.33, 127.80, 128.16, 128.24, 128.57, 138.59 (NCH2Ph, Cipso), 140.34 [CH(Ph)NHAc, Cipso], 168.93 [NH(C=O)CH3]. IR (ATR, cm-1): 3244, 3060, 2944, 2793, 2359, 2162, 1978, 1651, 1625, 1538, 1495, 1449, 1370, 1288, 1225, 1203, 1134, 1105, 1030, 737, 701, 598. HR-ESI-TOF [M+H]+ (m/z) calculated for C20H25N2O: 309.1961; found: 309.1965, (error = 1.1640).
(2R,3R)-2-Phenylpiperidin-3-amine, (R,R)-14. 0.54 g (1.8 mmol) of diamine (R,R)-12 was dissolved in 50 mL of EtOH acidified with 0.12 mL of AcOH (0.12 g, 2 mmol) under inert atmosphere in a quartz glass for hydrogenation reactor. Subsequently, 15% w/w Pd(OH)2 (1%) was added to the solution and then, the recipient was placed inside a reactor with mechanical stirring. Afterwards, the system was purged twice with hydrogen pressure. Finally, the reactor was charged with 1000 psi of hydrogen pressure and heated at 60 °C during 72 h. After this period, temperature was automatically set to room temperature and the suspension is poured onto a celite pad to remove the catalyst and the filtrate was concentrated under vacuum. The crude was purified with column chromatography, using CH2Cl2:MeOH:NH4OH(conc) (90:9:1) as eluent, obtaining the corresponding diamine in 80% yield as a yellow oil. δH (CDCl3, 500 MHz): 1.32-1.45 (m, 1H, CH2CH2CHNH2), 1.63-1.79 (m, 2H, CH2CH2CHNH2), 1.81-1.92 (m, 1H, CH2CH2CHNH2), 2.68 (td, 1H, J = 11.77, 2.65 Hz, CH2CH2NH), 2.90 (d, 1H, J = 1.83 Hz, CH2CH2CHNH2), 3.11 (dd, 1H, J = 10.39, 3.06 Hz, CH2CH2NH), 3.74 (d, 1H, J = 1.22 Hz, CHCHNH), 7.07-7.32 (m, 5H, ArH); δC (CDCl3, 125 MHz): 19.48 (NHCH2-CH2-CH2-), 31.94 (NHCH2-CH2-CH2-), 47.28 (NHCH2CH2CH2), 50.48 (CH2CHNH2), 64.60 [CH(Ph)NH], ArC: 126.30, 126.71, 128.07, 142.45 [CH(Ph), Cipso]. Chemical shifts and other spectroscopic data are in agreement with those reported in the literature.13a
General procedure for the conversion of amino group into a thiourea functionality. A determined amount of the required diamine was dissolved with anhydrous THF in a round-bottomed flask equipped with magnetic stirring and conditioned with nitrogen atmosphere. Subsequently, 1.6 equivalents of Edman reagent were added at 0 °C. Then, the reaction mixture was left stirring during 48 h at room temperature. After this time, the reaction was concentrated and the crude was extracted twice with brine and AcOEt. Subsequently, the organic layer was dried with Na2SO4, the solvent was evaporated under reduced pressure and the resultant concentrate is supported on silica and purified by flash chromatography using hexane:AcOEt (8:2) as eluent, giving rise to a pure thiourea.
1-{(R)-[(S)-1-Benzylpyrrolidin-2-yl](phenyl)methyl}-3-phenylthiourea, (S,R)-15. The general procedure described above was followed with 1.4 g (5.3 mmol) of (S,R)-1, 1.6 mL (1.14 g, 8.4 mmol) of PhN=C=S and 50 mL of THF to afford 1.49 g of the desired thiourea (S,R)-15 (3.7 mmol, 71% yield), as a pale yellow foam, Rf 0.34 (hexane/AcOEt, 7:3); [α]D25 -45 (c 1.0, CHCl3), δH (DMSO-d6, 120 °C, 500 MHz): 1.48-1.68 (m, 5H), 2.21-2.28 (m, 1H), 2.76-2.88 (m, 2H), 3.31 (d, 1H, J = 12.8 Hz), 3.99 (d, 1H, J =12.6 Hz), 5.69 (s, 1H), 7.05-7.40 (m, 15H). δC (DMSO-d6, 120 °C, 125 MHz): 22.71, 26.88, 53.85, 58.48, 58.98, 68.06, 114.86, 123.88, 124.78, 124.85, 126.98, 127.21, 127.49, 128.53, 128.96, 129.16, 129.25, 140.19, 182.22. IR (ATR, cm-1): νmax 2966, 2798, 1597, 1521, 1494, 1451, 732, 695. HR-ESI-TOF [M+H]+ calculated for C25H28N3S: 402.1998; found: 402.2002 (error = 0.8792).
1-{(S)-[(S)-1-Benzylpyrrolidin-2-yl](phenyl)methyl}-3-phenylthiourea, (S,S)-15. The general procedure described above was followed with 1.25 g (4.7 mmol) of (S,S)-1, 1.43 mL (1.02 g, 7.5 mmol) of PhN=C=S and 40 mL of THF to afford 1.31 g of the desired thiourea (S,S)-15 (3.3 mmol, 69% isolated yield), as a white solid, mp 155-156 °C; Rf 0.38 (hexane/AcOEt, 7:3); [α]D25 –212 (c 1.02, CHCl3), δH (DMSO-d6, 120 °C, 500 MHz): 1.61 (s, 2H), 1.85 (br, 1H), 2.04 (br, 1H), 2,17 (br, 1H), 2.55 (br, 1H), 2.83 (br, 1H), 3.12 (br, 1H), 3.21 (br, 1H), 5.53 (br, 1H), 6.71 (br, 1H), 7.14-7.46 (m, 15H). δC (DMSO-d6, 120 °C, 125 MHz): 24.14, 31.22, 55.11, 60.36, 61.77, 68.49, 125.68, 126.32, 126.69, 127.01, 127.93, 128.16, 128.73, 130.32, 136.29, 139.50, 141.34, 150.00, 181.08. IR (ATR, cm-1): νmax 3140, 2956, 1595, 1491, 1449, 1243, 1067, 794, 750, 668, 696. HR-ESI-TOF [M+H]+ calculated for C25H28N3S: 402.1998; found: 402.1997 (error = -0.3639). Anal. Calcd for C25H27N3S: C, 74.77; H, 6.78; N, 10.46. Found: C, 74.98; H, 7.02; N, 10.66.
General procedure for the conversion of amino group into a sulfonamide functionality. A determined amount of the required diamine was dissolved with anhydrous THF in a round-bottomed flask equipped with magnetic stirring and conditioned with nitrogen atmosphere. Subsequently, 1.1 equiv of Et3N were added at room temperature. Afterwards, 1.05 equiv. of the required 4-substituted benzenesulfonyl chloride, dissolved in dry THF, was slowly added to the mixture at 0 °C and stirred during 1-2 h at this temperature. Then, the reaction was left stirring overnight at room temperature and later, the solution was concentrated and the crude was extracted twice with brine and CH2Cl2. The combined organic layers were dried with Na2SO4 and concentrated under reduced pressure. The resultant concentrate is supported on silica and purified by flash chromatography using Hexane:AcOEt (8:2) as eluent, giving rise to the corresponding sulfonamide.
N-{(R)-[(S)-1-Benzylpyrrolidin-2-yl](phenyl)methyl}-4-nitrobenzenesulfonamide, (S,R)-16. The general procedure described above was followed with 1.4 g (5.3 mmol) of (S,R)-1, 0.81 mL (0.59 g, 5.8 mmol) of Et3N, 1.22 g (5.5 mmol) of 4-nitrobenzenesulfonyl chloride and 50 mL of THF; obtaining after recrystallization, 1.6 g of sulfonamide (S,R)-16 (3.54 mmol, 67% isolated yield) as pale yellow crystals, mp 162-163 °C (AcOEt/hexane); Rf 0.26 (hexane/AcOEt, 7:3); [α]D25 -92 (c 1.0, CHCl3), δH (CDCl3, 500 MHz): 1.32-1.35 (m, 1H), 1.56-1.60 (m, 2H), 1.75-1.77 (m, 1H), 2.22 (dd, 1H, J = 8.6, 17.95 Hz), 2.75 (ddd, 1H, J = 7.95, 4.5, 8.0 Hz), 3.03-3.04 (m, 1H), 3.18 (d, 1H, J = 13.4 Hz), 3.76 (d, 1H, J = 13.35 Hz), 4.53 (d, 1H, J = 4.5 Hz), 7.25 (m, 10H); 7.74 (d, 2H, J = 8.95Hz), 7.99 (d, 2H, J = 8.95Hz). δC (CDCl3, 125 MHz): 21.72, 25.31, 53.97, 57.15, 57.21, 67.68, 123.80, 127.37, 127.40, 127.81, 128.26, 128.30, 128.62, 128.71, 137.71, 138.15, 145.37, 149.78. IR (ATR, cm-1): νmax 3172, 2880, 1527, 1338, 1309, 1172, 1127, 1090, 858, 735, 687. HR-ESI-TOF [M+H]+ calculated for C24H26N3O4S: 452.1638; found: 452.1638 (error = -0.1212). Anal. Calcd for C24H25N3O4S: C, 63.84; H, 5.58; N, 9.31; S, 7.10. Found: C, 63.98; H, 5.63; N, 9.13; S, 6.86.
N-{(S)-[(S)-1-Benzylpyrrolidin-2-yl](phenyl)methyl}-4-nitrobenzenesulfonamide, (S,S)-16. The general procedure described above was followed with 1.48 g (5.6 mmol) of (S,S)-1, 0.85 mL (0.62 g, 6.1 mmol) of Et3N, 1.34 g (6 mmol) of 4-nitrobenzenesulfonyl chloride and 50 mL of THF to obtain 1.71 g of sulfonamide (S,S)-16 (3.79 mmol, 68%) as a yellow resin-like oil; Rf 0.54 (hexane/AcOEt, 7:3); [α]D25 +94 (c 1.05, CHCl3), δH (CDCl3, 500 MHz): 1.22-1.28 (m, 1H), 1.60-1.66 (m, 1H), 1.70-1.80 (m, 2H), 1.89-1.98 (m, 1H), 2.39-2.46 (m, 1H), 2.93-2.99 (m, 1H), 3.04-3.10 (m, 1H), 3.30 (d, 1H, J = 12.95 Hz), 3.41 (d, 1H, J = 12.95 Hz), 4.26 (d, 1H, J = 5.1 Hz), 6.99 (m, 2H), 7.08 (m, 3H), 7.20 (m, 2H), 7.29 (m, 3H), 7.67 (d, 2H, J = 9.1Hz), 8.07 (d, 2H, J = 9.05 Hz). δC (CDCl3, 125 MHz): 24.20, 29.26, 54.66, 61.07, 61.20, 68.67, 123.69, 127.25, 127.39, 127.65, 128.25, 128.37, 128.62, 128.87, 139.05, 139.14, 146.88, 149.52. IR (ATR, cm-1): νmax 3292, 2962, 2800, 1606, 1527, 1494, 1347, 1309, 1106, 1091, 853, 926, 735, 699, 605. HR-ESI-TOF [M+H]+ calculated for C24H26N3O4S: 452.1638; found: 452.1647 (error = 1.8691).
N-{(R)-[(S)-1-Benzylpyrrolidin-2-yl](phenyl)methyl}-4-methylbenzenesulfonamide, (S,R)-17. The general procedure for sulfonamide synthesis was followed with 1.4 g (5.3 mmol) of (S,R)-1, 0.81 mL (0.59 g, 5.8 mmol) of Et3N, 1.06 g (5.6 mmol) of 4-methylbenzene-1-sulfonyl chloride and 50 mL of THF; obtaining after recrystallization, 1.5 g of sulfonamide (S,R)-17 (3.57 mmol, 67% isolated yield) as white crystals, mp 140 °C; Rf 0.49 (hexane/AcOEt, 7:3); [α]D25 -104 (c 1.0, CHCl3), δH (CDCl3, 500 MHz): 1.21-1.31 (m, 1H, CH2CH2CH-), 1.45-1.68 (m, 2H, CH2CH2CH-), 1.81 (dddd, 1H, J = 13.35, 10.27, 8.16, 5.51 Hz, CH2CH2CH-), 2.13 (td, 1H, J = 9.16, 8.45 Hz, CHNCH2CH2), 2.27 (s, 3H, C6H5CH3), 2.64 (td, 1H, J = 8.16, 4.41 Hz, NCHCHPh), 2.97 (ddd, 1H, J = 9.45, 7.59, 2.58 Hz, CHNCH2CH2), 3.00, 3.57 (d, 2 x 1H, J = 13.25 Hz, NCH2Ph), 4.37 (d, 1H, J = 4.41 Hz, NCHCHPh), 5.5-6.0 (br, 1H, NH), 6.98-7.02 (m, 2H, ArH), 7.14-7.18 (m, 2H, ArH), 7.19-7.3 (m, 6H, ArH), 7.3-7.35 (m, 2H, ArH), 7.53-7.57 (m, 2H, ArH). δC (CDCl3, 125 MHz): 21.41 (-C6H4CH3), 21.48 (-CH2CH2CH-), 24.89 (CH2CH2CH-), 53.72 (CHNCH2CH2), 56.58 (NCHCHPh), 56.77 (NCH2Ph), 67.92 (NCHCHPh), ArC: 126.99, 127.16, 127.26, 127.44, 127.98, 128.25, 128.31, 129.26, 135.92 (NCH2Ph, Cipso), 138.22 (SO2C6H4, Cipso), 138.63 (NCHCHPh, Cipso), 143.25 (C6H4-CH3, Cipso). IR (ATR, cm-1): νmax 3303, 2926, 2801, 1599, 1423, 1321, 1152, 1054, 839, 807, 755, 697, 670, 590. MS (IE) m/z (%): 160.1 (100), 161.1 (12.96). HR-ESI-TOF [M+H]+ calculated for C25H29N2O2S: 421.1944; found: 421.1943 (error = -0.3007).
N-{(S)-[(S)-1-Benzylpyrrolidin-2-yl](phenyl)methyl}-4-methylbenzenesulfonamide, (S,S)-17. The general procedure for sulfonamide synthesis was followed with 1.4 g (4.24 mmol) of (S,S)-1, 0.65 mL (0.47 g, 4.7 mmol) of Et3N, 0.88 g (4.6 mmol) of 4-methylbenzenesulfonyl chloride and 40 mL of THF; obtaining after recrystallization, 1.38 g of sulfonamide (S,S)-17 (3.28 mmol, 77% isolated yield) as white crystals, mp 137-138 °C (AcOEt/hexane); Rf 0.54 (hexane/AcOEt, 7:3); [α]D25 +127 (c 0.98, CHCl3), δH (CDCl3, 500 MHz): δH (CDCl3, 500 MHz): 1.49-1.71 (m, 3H, -CH2CH2CH-), 1.89 (ddd, 1H, J = 17.61, 12.39, 8.95 Hz, -CH2CH2CH-), 2.28 (ddd, 1H, J = 9.74, 9.09, 6.8 Hz, CHNCH2CH2), 2.35 (s, 3H, C6H5CH3), 2.82 (ddd, 1H, J = 9.88, 6.52, 3.44 Hz, CHNCH2CH2), 3.04 (dt, 1H, J = 9.16, 4.03 Hz, NCHCHPh), 3.13, 3.38 (d, 2 x 1H, J = 12.96 Hz, NCH2Ph), 4.28 (d, 1H, J = 4.03, NCHCHPh), 7.08-7.19 (m, 9H, ArH), 7.21-7.33 (m, 3H, ArH), 7.54-7.58 (m, 2H, ArH). δC (CDCl3, 125 MHz): 21.41 (-C6H4CH3), 23.91 (-CH2CH2CH-), 29.34 (-CH2CH2CH-), 54.32 (CHNCH2CH2), 60.33 (NCHCHPh), 60.68 (NCH2Ph), 68.80 (NCHCHPh), ArC: 126.82, 126.96, 127.03, 128.09, 128.30, 128.62, 129.14, 137.75 (NCH2Ph, Cipso), 139.06 (SO2C6H4-, Cipso), 140.31 (NCHCHPh, Cipso), 142.75 (C6H4-CH3, Cipso). IR (ATR, cm-1): νmax 3303, 2926, 2801, 1599, 1423, 1321, 1152, 1054, 839, 807, 755, 697, 670, 590. HR-ESI-TOF [M+H]+ calculated for C25H29N2O2S: 421.1944; found: 421.1949 (error = 1.1237). Anal. Calcd for C25H28N2O2S C, 71.40; H, 6.71; N, 6.66. Found: C, 71.04; H, 6.89; N, 6.98.
ACKNOWLEDGMENT
We are indebted to CONACyT for financial support via (project No. 130826). Jorge Vargas-Caporali and Carlos Cruz-Hernández are grateful to CONACyT for their doctoral fellowships.
References
1. (a) R. S. Cheeseman et al., Du Pont Merck, U. S. Patent US 1993/5254569; (b) G. Dargazanli et al., Sanofi-Aventis, U. S. Patent US 2007/0208006 A1.
2. (a) J. L. Olivares-Romero and E. Juaristi, Tetrahedron, 2008, 64, 9992; CrossRef (b) B. Ni, Q. Zhang, and A. D. Headley, Tetrahedron Lett., 2008, 49, 1249; CrossRef (c) M. Pouliquen, J. Blanchet, and J. Maddaluno, Tetrahedron: Asymmetry, 2010, 21, 1511; CrossRef (d) L.-W. Xu, J. Luo, and Y. Lu, Chem. Commun., 2009, 1807. CrossRef
3. (a) R. Roudeau, D. Gomez Pardo, and J. Cossy, Tetrahedron, 2006, 62, 2388; CrossRef (b) T. Hamada, K. Manabe, and S. Kobayashi, Chem. Eur. J., 2006, 12, 1205; CrossRef (c) R. Almansa, D. Guijarro, and M. Yus, Tetrahedron:Asymmetry, 2007, 18, 2828; CrossRef (d) J. E. D. Martins and M. Wills, Tetrahedron:Asymmetry, 2008, 19, 1250; CrossRef (e) S. Bräse, T. Baumann, S. Dahmen, and H. Vogt, Chem. Commun., 2007, 1881; CrossRef (f) R. Melgar-Fernández, R. González-Olvera, J. L. Olivares-Romero, V. González-López, L. Romero-Ponce, M. Ramírez-Zárate, P. Demare, I. Regla, and E. Juaristi, Eur. J. Org. Chem., 2008, 655; CrossRef (g) C.-L. Liu, C.-Y. Wei, S.-W. Wang, and Y.-G. Peng, Chirality, 2011, 23, 921; CrossRef (h) M. Jaworska, M. Wełniak, J. Zięciak, A. Kozakiewicz, and A. Wojtczak, ARKIVOC, 2011, ix, 189.
4. R. H. Reitsema, J. Am. Chem. Soc., 1949, 71, 2041. CrossRef
5. R. C. Fuson and C. L. Zirkle, J. Am. Chem. Soc., 1948, 70, 2760. CrossRef
6. (a) R. Paul and S. Tchelitcheff, Bull. Soc. Chim. Fr., 1958, 736; (b) C. F. Hammer and J. D. Weber, Tetrahedron, 1981, 37, 2173. CrossRef
7. For a thorough overview including reaction conditions, kinetics and stereochemistry of this reaction, see: C. F. Hammer, S. R. Heller, and J. H. Craig, Tetrahedron, 1971, 28, 239. CrossRef
8. (a) D. Hugues, Org. Prep. Proced. Int., 1996, 28, 127; CrossRef (b) A. Dondoni, B. Richichi, A. Marra, and D. Perrone, Synlett, 2004, 1711. CrossRef
9. (a) J. Cossy and D. Gomez Pardo, CHEMTRACTS-Org. Chem., 2002, 15, 579; (b) S. B. D. Jarvis and A. B. Charette, Org. Lett., 2011, 13, 3830; CrossRef (c) O. Calvez and N. Langlois, Tetrahedron Lett., 1998, 39, 9447; CrossRef Ibid., 1999, 40, 7099; CrossRef (e) S. Kovačková, M. Dračínský, and D. Rejman, Tetrahedron, 2011, 67, 1485. CrossRef
10. (a) B. Burger, C. Navarro, D. Gomez Pardo, J. Cossy, Y. Zhao, and T. Cohen, Synlett, 2009, 2157; CrossRef (b) Y. Shishido, H. Wakabayashi, H. Koike, Naomi Ueno, A. Sakakibara, and K. Satake, Bioorg. Med. Chem., 2008, 16, 7193. CrossRef
11. J. L. Bilke, S. P. Moore, P. O’Brien, and J. Gilday, Org. Lett., 2009, 11, 1935. CrossRef
12. (a) A. Cochi, D. Gomez Pardo, and J. Cossy, Org. Lett., 2011, 13, 4442; CrossRef (b) I. Déchamps, D. Gomez Pardo, and J. Cossy, Synlett, 2007, 263; CrossRef (c) J. Cossy, D. Gomez Pardo, C. Dumas, O. Mirguet, I. Déchamps, T.-X. Métro, B. Burger, R. Roudeau, J. Appenzeller, and A. Cochi, Chirality, 2009, 21, 850; CrossRef (d) A. Cochi, D. Gomez Pardo, and J. Cossy, Eur. J. Org. Chem., 2012, 2023. CrossRef
13. (a) C. G. Kokotos and V. K. Aggarwal, Chem. Commun., 2006, 2156; CrossRef (b) M. Ahari, A. Perez, C. Menant, J.-L. Vasse, and J. Szymoniak, Org. Lett., 2008, 10, 247; CrossRef (c) H. M. Lovick and F. E. Michael, J. Am. Chem. Soc., 2010, 132, 1249. CrossRef
14. (a) B. Kazi, L. Kiss, E. Forró, and F. Fülöp, Tetrahedron Lett., 2010, 51, 82; CrossRef (b) T. Morie and S. Kato, Heterocycles, 1998, 48, 427; CrossRef (c) S. Zhao, J. P. Freeman, C. L. Bacon, G. B. Fox, E. O'Driscoll, A. G. Foley, J. Kelly, U. Farrell, C. Regan, S. A. Mizsak, and J. Szmuszkovicz, Bioorg. Med. Chem., 1999, 7, 1647. CrossRef
15. B. D. Nahre, V. Sriramurthy, and V. K. Yadav, Org. Biomol. Chem., 2012, 10, 4390. CrossRef
16. G. Cremonesi, P. Dalla Croce, F. Fontana, A. Forni and C. La Rosa, Tetrahedron: Asymmetry, 2007, 18, 1667. CrossRef
17. Cf. A. Krasovski, F. Kopp, and P. Knochel, Angew. Chem. Int. Ed., 2006, 45, 497. CrossRef
18. D. D. Perrin and W. L. F. Armarego, ‘Purification of Laboratory Chemicals’, Pergamon Press, Inc., London, 1988, p. 100.
19. A. Krasovskiy and P. Knochel, Synthesis, 2006, 890. CrossRef
20. Crystal data for (R,R)-8: C18H20ClN, monoclinic, space group P21, a = 6.1910(0) Å, b = 16.5440(0) Å, c = 7.7750(0) Å, α = 90 °, β = 99.759(0) °, γ = 90 °, V = 784.82 Å3, crystal size: 0.625 x 0.55 x 0.5 mm3, R1 = 0.0393, (wR2 = 0.0994). CCDC 898455 contains the supplementary crystallographic data for this paper. These data can be obtained free of charge from The Cambridge Crystallographic Data Center via http://www.ccdc.cam.ac.uk/data_request/cif.
21. S. H. L. Verhelst, B. Paez Martinez, M. S. M. Timmer, G. Lodder, G. A. Van der Marel, H. S. Overkleeft, and J. H. Van Boom, J. Org. Chem., 2003, 68, 9598. CrossRef
22. Crystal data for (S,S)-11: C20H24N2O, monoclinic, space group C2, a = 20.8080(0) Å, b = 5.1620(0) Å, c = 16.3680(0) Å, α = 90 °, β = 97.510(0) °, γ = 90 °, V = 1743.02 Å3, crystal size: 0.8 x 0.1 x 0.08 mm3, R1 = 0.0568, (wR2 = 0.1362). CCDC 898459 contains the supplementary crystallographic data for this paper. These data can be obtained free of charge from The Cambridge Crystallographic Data Center via http://www.ccdc.cam.ac.uk/data_request/cif.
23. Crystal data for (S,R)-11: C20H24N2O, orthorhombic, space group P212121, a = 8.4980(0) Å, b = 9.8990(0) Å, c = 21.46999(0) Å, α = 90 °, β = 90 °, γ = 90 °, V = 1806.09 Å3, crystal size: 0.425 x 0.375 x 0.125 mm3, R1 = 0.0730, (wR2 = 0.2048). CCDC 898457 contains the supplementary crystallographic data for this paper. These data can be obtained free of charge from The Cambridge Crystallographic Data Center via http://www.ccdc.cam.ac.uk/data_request/cif.
24. Crystal data for (R,R)-13: C20H24N2O, orthorhombic, space group P31, a = 20.573(0) Å, b = 20.573(0) Å, c = 11.2766(0) Å, α = 90 °, β = 90 °, γ = 120 °, V = 4129.48 Å3, crystal size: 0.25 x 0.25 x 0.15 mm3, R1 = 0.0774, (wR2 = 0.1998). CCDC 898456 contains the supplementary crystallographic data for this paper. These data can be obtained free of charge from The Cambridge Crystallographic Data Center via http://www.ccdc.cam.ac.uk/data_request/cif.
25. See, for example: M. Hernández-Rodríguez, C. G. Ávila-Ortiz, J. M. del Campo, D. Hernández-Romero, M. J. Rosales-Hoz, and E. Juaristi, Aust. J. Chem., 2008, 61, 364. CrossRef
26. See, for example: M. Hernández-Rodríguez, and E. Juaristi, Tetrahedron, 2007, 63, 7673. CrossRef
27. (a) Z. Zhang and P. R. Schreiner, Chem. Soc. Rev., 2009, 38, 1187; CrossRef (b) Y. Yamaoka, H. Miyabe, Y. Yasui, and Y. Takemoto, Synthesis, 2007, 2571; CrossRef (c) T. Okino, S. Nakamura, T. Furukawa, and Y. Takemoto, Org. Lett., 2004, 6, 625; CrossRef (d) T. Okino, Y. Hoashi, and Y. Takemoto, J. Am. Chem. Soc., 2003, 125, 12672; CrossRef (e) M. P. Lalonde, Y. Chen, and E. N. Jacobsen, Angew. Chem. Int. Ed., 2006, 45, 6366. CrossRef
28. (a) Y.-J. Cao, Y.-Y. Lai, X. Wang, Y.-J. Li, and W.-J. Xiao, Tetrahedron Lett., 2007, 48, 21; CrossRef (b) L. Wang, C. Cai, D. P. Curran, and W. Zhang, Synlett, 2010, 433. CrossRef
29. Crystal data for (S,R)-17: C25H28N2O2S, monoclinic, space group P2, a = 10.2330(0) Å, b = 9.7000(0) Å, c = 11.6750(0) Å, α = 90 °, β = 101.2099 °, γ = 90 °, V = 1136.75 Å3, crystal size: 0.45 x 0.3 x 0.125 mm3, R1 = 0.0432, (wR2 = 0.1117). CCDC 898458 contains the supplementary crystallographic data for this paper. These data can be obtained free of charge from The Cambridge Crystallographic Data Center via http://www.ccdc.cam.ac.uk/data_request/cif.
30. D. D. Perrin and W. L. F. Armarego, ‘Purification of Laboratory Chemicals’, Pergamon Press, Inc., London, 1988, pp. 145, 161, 284, 296.
31. (a) P. Huy, J.-M. Neudörfl, and H.-G. Schmalz, Org. Lett., 2011, 13, 216; CrossRef (b) G. Szöllősi, G. London, L. Baláspiri. C. Somlai, and M. Bartók, Chirality, 2003, 15, S90. CrossRef
32. P.-A. Wang, W.-M. Liu, and X.-L. Sun, Org. Prep. Proced. Int., 2011, 43, 477. CrossRef
33. S. Itsuno, I. Shinichi, A. Hirao, and S. Nakahama, J. Chem. Soc., Perkin Trans. 1, 1984, 2887. CrossRef
34. W. Yang, B. Mao, S. Zhu, X. Jiang, Z. Liu, and R. Wang, Eur. J. Org. Chem., 2009, 3790. CrossRef
35. K. Thai, L. Wang, T. Dudding, F. Bilodeau, and M. Gravel, Org. Lett., 2010, 12, 5708. CrossRef