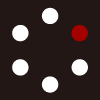
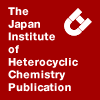
HETEROCYCLES
An International Journal for Reviews and Communications in Heterocyclic ChemistryWeb Edition ISSN: 1881-0942
Published online by The Japan Institute of Heterocyclic Chemistry
e-Journal
Full Text HTML
Received, 4th June, 2012, Accepted, 5th July, 2012, Published online, 17th July, 2012.
DOI: 10.3987/COM-12-S(N)27
■ Enantioselective Synthesis of cis- and trans-2-Methyl-6-nonylpiperidines: Alkaloids Solenopsin and Isosolenopsin
Mohamed Medjahdi, José Carlos González-Gómez,* Francisco Foubelo, and Miguel Yus*
Department of Organic Chemistry, Faculty of Sciences and Institute of Organic Synthesis (ISO), University of Alicante, P.O. Box 99, 03080 Alicante, Spain
Abstract
The cross-metathesis of the enantioenriched homoallylic amine 8 (readily accessible by α-aminoallylation of decanal) with methyl vinyl ketone using the Hoveyda-Blechert catalyst 10 in presence of 10 mol% of Ti(O-i-Pr)4 led exclusively to the (E)-enone 11, which by stereoselective reductive amination affords (+)-isosolenopsin (3a) and (+)-solenopsin (4a) with excellent selectivities.Piperidine ring constitutes a common structural motif of many biologically active natural products. Particularly, cis- and trans-6-alkyl-2-methylpiperidines are alkaloids which have been isolated from insects, amphibians and plants. Since these compounds display important biological activity,1 great efforts have been made to achieve their enantioselective synthesis.2 For instance, dihydropinidine (1),3 isolated from the Mexican bean beetle Epilachna varivestis, and alkaloid 241D (2), isolated from blue poison dart frog Dendrobates azureus,4 both exhibit interesting biological properties. On the other hand, solenopsin (4a, n = 8) and isosolenopsin (3a, n = 8) were isolated from the fire ants (Solenopsis) and display, hemolytic, insecticide and antibiotic properties (Figure 1).5 Intramolecular reductive amination of δ-amino ketones is a recurrent strategy for the synthesis of 2,6-disubstituted piperidines. Most of the reduction conditions lead to the cis-product, because it is assumed the axial hydride attack to the most stable half-chair of the iminic intermediate (stereoelectronic control).6 However, when the reduction is performed in the presence of a bulky Lewis acid, such as AlMe3, less stable trans-2,6-disubstituted piperidines are produced selectively.7 In this case, after coordination of AlMe3 to the nitrogen,
the equilibrium is shifted to the half-chair with the axial R group, in order to minimize the allylic repulsion A1,2. Consequently, the axial hydride attack to the iminic carbon leads to the trans-product (Scheme 1).
We have recently reported the stereoselective α-aminoallylation of aldehydes with chiral N-tert-butanesulfinamide and allylic bromides,8 and also the first example of cross metathesis of chiral N-tert-butane sulfinyl homoallyl amines with methyl vinyl ketone using Hoveyda-Blechert´s catalyst 10.9 These methodologies were applied to a concise synthesis of the natural (+)-isosolenopsin (3a, n = 8). With these antecedents in hands we found of interest to improve the synthesis of (+)-isosolenopsin (3a, n = 8),8 and to carry out the synthesis of its trans-isomer (+)-solenopsin (4a, n = 8), from a common precursor.
A mixture of decanal (5, 1.1 equivalents), (S)-N-tert-butanesulfinamide (6, 1.0 equivalents), titanium tetraethoxide (2.0 equivalents) and indium metal (1.0 equivalents) was stirred at room temperature for 1 hour. After that, allyl bromide (7, 1.2 equivalents) was added, and the reaction mixture was heated at 60 ºC over 5 hours to give homoallyl amine derivative 8 in 81% yield and 95:5 dr (Scheme 2). Nucleophilic addition took mainly place to the Re-face of the (SS)-imine intermediate and the major diastereoisomer 8 was isolated in enantiomerically pure form by column chromatography. In our previous reports on the synthesis of (+)-isosolenopsin (3a),8,10 we found that the cross metathesis of N-tert-butanesulfinyl amine 8 with commercially available methyl vinyl ketone (9) took place smoothly at
45 ºC for 48 hours in the presence of the Hoveyda-Blechert ruthenium catalyst 10, to give enone 11 in 72% yield. The yield was notably improved and the reaction time was shortened by performing the cross metathesis with the same ruthenium catalyst but using also a 10 mol% of titanium tetraisopropoxide as an additive. Presumably, titanim tetraiosopropoxide compete with the ruthenium carbine for the coordination of the sulfinyl group.11 This accelerating effect of titanium tetraisopropoxide has been also observed by other authors in the cross metathesis of alkene sulfinamides.12 Under these reaction conditions, enone 11 was isolated after 24 hours at 45 ºC in 90% yield (Scheme 2). It is noteworthy that enone 11 showed always (E)-configuration exclusively. Quantitative hydrogenation of the double bond of enone 11 with molecular hydrogen was obtained when Wilkinson catalyst was used. By contrast, hydrogenation did not take place by means of palladium or platinum catalysts. An explanation for this behaviour is that the sulfinyl group could poison the catalyst. The sulfinyl group was removed under acidic conditions to give imine 12 in high yield, and it was not necessary its purification for the next transformations. Reduction of imine 12 with sodium borohydride in a citrate-phosphate buffer medium (pH 5) led to (+)-isosolenopsin (3a) in 93% yield and >98:2 cis-trans selectivity. On the other hand, when the reduction of imine 12 was carried out applying H. Yamamoto’s protocol (AlMe3/LiAlH4),7 (+)-solenopsin (4a) was isolated in 83% yield and with excellent diastereoselectivity (>98:2 trans-cis selectivity, Scheme 2).
In summary, 2,6-disubstituted piperidinic alkaloids (+)-isosolenopsin (3a) and (+)-solenopsin (4a) were accessible from a common imine intermediate 12 through four synthetic operation (α-amino allylation, cross metathesis, hydrogenation-desulfinylation, stereoselective reduction) and three purification steps, from commercially available n-decanal, (S)-N-tert-butanesulfinamide, allyl bromide and methyl vinyl ketone.
EXPERIMENTAL
All chemicals were commercially available (Acros, Aldrich). TLC was performed on Merck silica gel 60 F254, using aluminum plates and visualized with phosphomolybdic acid (PMA) stain. Chromatographic purification was performed by flash chromatography using Merck silica gel 60 (0.040-0.063 mm) and hexane/EtOAc as eluent. GC-MS was developed in an Agilent 6890N spectrometer with FID detector, helium gas transportation (2 mL/min), 12 psi injection pressure, 270 ºC temperature in detection and injection blocks, 1.0 µL volume of sample and 5 mm/min registration speed. Program temperature: 60 ºC, initial temperature for 3 min; heating 15 ºC/min; 270 ºC, final temperature. Column type HP-1, 12 m long, 0.22 mm internal diameter, 0.25 μm thickness methylsilicone rubber and OV-101 stationary phase. IR spectra were measured (film) with a Nicolet Impact 510 P-FT Spectrometer. Melting points were recorded on an OptiMelt (Stanford Research Systems) apparatus and reported without corrections. HPLC analyses were performed on a JASCO 200-series equipped with a Chiralcel OD-H column (condidtions: hexane/isopropanol, 9:1; 0.5 mL/min). NMR spectra were recorded with a Bruker AC-300 or a Bruker ADVANCE DRX-500 using CDCl3 as the solvent and TMS as internal standard. HRMS (EI) were recorded on a Finnigan MAT 95S.
(4S,SS)-N-(tert-Butanesulfinyl)tridec-1-en-4-amine (8). A mixture of indium powder (173 mg, 1.50 mmol), (SS)-N-tert-butanesulfinamide (6, 121 mg, 1.00 mmol), decanal (5, 180 mg, 0.216 mL, 1.15 mmol) and Ti(OEt)4 (456 mg, 0.450 mL, 2.00 mmol) in THF (2 mL) was stirred under argon for 1 h at 23 ºC. Then, the corresponding allyl bromide (7, 181 mg, 0.130 mL, 1.50 mmol) was added and the reaction mixture heated for 5 h at 60 ºC. The mixture was left cooling to room temperature, quenched with brine (2 mL) and diluted with EtOAc (10 mL). The resulting suspension was filtered through a short pad of Celite and concentrated under vacuum (15 Torr). The residue was purified by column chromatography (silica gel, hexane/EtOAc) to yield 244 mg of pure product 8 (81% yield). Physical and spectroscopic data follow: Colourless oil; [α]20D +45.8 (c 2.6, CH2Cl2); Rf 0.49 (hexane/AcOEt 2:1); IR ν (film) 3217, 1643 cm-1; 1H NMR (300 MHz, CDCl3) δ 0.88 (3H, t, J = 6.9, CH3), 1.21 [9H, s, C(CH3) 3], 1.23–1.34 (14H, m, 7 × CH2), 1.49 (2H, m, CH2), 2.31 (1H, dt, J = 13.7, 6.9, CHH), 2.41 (1H, dt, J = 12.8, 6.4, CHH), 3.22 (1H, d, J = 6.1, NH), 3.28-3.34 (1H, m, CHNH), 5.12-5.18 (2H, m,CH=CH2), 5.74-5.81 (m, 1H, CH=CH2); 13C NMR (75 MHz, CDCl3) δ 14.2, 22.8 (CH3), 25.6, 29.4, 29.6, 29.6, 29.7, 32.0, 35.0, 40.6 (CH2), 55.0 (CH), 55.9 (C), 119.0 (CH2), 134.4 (CH); HRMS (EI) calcd for C13H27NOS (M-C4H8) 245.1813, found 245.1822.
(6S,E)-N-[(SS)-tert-Butanesulfinyl]-6-aminopentadec-3-en-2-one (11). To a solution of compound 8 (210.7 mg, 0.70 mmol) in dry dichloromethane (12 mL) under argon was successively added methyl vinyl ketone (196 mg, 0.238 mL, 2.80 mmol), Hoveyda-Grubs ruthenium catalyst 10 (43 mg, 0.07 mmol) and a solution of titanium tetraisopropoxide (39 mg, 0.021 mL, 0.07 mmol) in dichloromethane (2 mL). The reaction mixture was stirred for 24 h at 45 °C. After removal of the solvent under vacuum (15 Torr), the residual brown oil was purified by column chromatography on silica gel (hexane/EtOAc) to yield 216 mg of pure product 11 [90% yield (E/Z >98:2)]. Physical and spectroscopic data follow: Brownish oil; [α]20D +25.0 (c 3.3, CHCl3); Rf 0.24 (hexane/AcOEt 1:2); IR ν (film) 3244, 1674, 1629 cm-1; 1H NMR (300 MHz, CDCl3) 0.88 (3H, t, J = 6.6, CH3), 1.21 [9H, s, C(CH3) 3], 1.20–1.40 (14H, m, 7 × CH2), 2.28 (3H, s, CH3), 2.51-2.59 (2H, m, CH2), 3.09 (1H, d, J = 7.7, NH), 3.35-3.40 (1H, m, CHNH), 6.15 (1H, d, J = 16.0, CH=CHCO), 6.83 (1H, dt, J = 16.0, 7.5, CH=CHCO); 13C NMR (75 MHz, CDCl3) δ 14.2, 22.7 (CH3), 25.8 (CH2), 27.2 (CH3), 29.3, 29.4, 29.6, 31.9, 35.8, 39.7 (CH2), 56.2, 134.1, 143.7 (CH), 198.5 (CO); LRMS (MALDI) m/z 344.243 (M+H), 366.251 (M+Na).
(2R,6S)-2-Methyl-6-nonylpiperidine [(+)-isosolenopsin] (3a). A flame-dried flask was cooled under a stream of argon and charged with Wilkinson catalyst (50 mg, 0.05 mmol) and a solution of enone 11 (377 mg, 1.10 mmol) in EtOH (5.0 mL). A balloon of H2 was connected to the flask and the reaction mixture was stirred at room temperature for 14 h. After removal of the solvent under vacuum, the residual brown oil was dissolved in 1:1 hexane/t-BuOMe and filtered through a short pad of Celite to remove the solid (Ph3P=O). The organic solution was concentrated under vacuum (15 Torr) and the crude product (>95% yield according to 1H NMR) was used in the next step without further purification. To a solution of protected amino ketone (376 mg, 1.09 mmol) in MeOH (5 mL) was added a 4M HCl solution in dioxane (2.5 mL) at 0-5 ºC and the reaction mixture was stirred over 1 h while the temperature increase to 23 ºC. After solvent evaporation under vacuum (15 Torr), the residue was dissolved in citrate-phosphate buffer (3 mL) and THF (3 mL), adjusting the pH to 5 with 1M NaOH aqueous solution. To this solution was added sodium cyanoborohydride (100 mg, 1.6 mmol) at 0-5 ºC and the mixture was stirred for 3 h at 23 ºC. The reaction mixture was made basic with 4M NaOH aqueous solution (10 mL) and extracted with dichloromethane (3 × 20 mL). Organics were washed with brine, dried over potassium carbonate and concentrated (15 Torr) to afford 230 mg of the desired piperidine 3a (93%) as a pale yellow oil. GC-MS Rt (major) = 12.65 min; m/z (% abundance) 225 (1, M+), 224 (2), 210 (6), 99 (17), 98 (100), 70 (6), 69 (5), 56 (7), 55 (11); Rt (minor-2R,6R) = 12.90 min (similar fragmentation). The corresponding hydrochloride was crystallized using ethereal HCl. Recrystallization from 1:3 EtOH/EtOAc afforded pure product (cis/trans > 99:1), which spectral data was identical with those reported.13
(+)-Isosolenopsin hydrochloride: Colourless needles (cis/trans > 99:1 according to GC-MS); mp 172-174 ºC (lit.,14 174-175 ºC); [α]20D +10.2 (c 0.93, CHCl3) (lit.,14 [α]20D +11.1); 1H NMR (300 MHz, CDCl3) δ 0.88 (3H, t, J = 6.5, CH3), 1.20–1.50 (15H, m, 7 × CH2, CHH), 1.57 (3H, d, J = 6.2, CH3), 1.55–1.65 (1H, m, CHH), 1.70–2.00 (5H, m, 2 × CH2, CHH), 2.09–2.18 (1H, m, CHH), 2.79–2.94 (1H, m, CHN), 3.02–3.18 (1H, m, CHN), 9.06 (1H, br s, NHH), 9.44 (1H, br s, NHH); 13C NMR (75 MHz, CDCl3) δ 14.2, 19.6 (CH3), 22.8, 23.0, 25.8, 27.6, 29.6, 29.5, 29.4, 29.7, 30.9, 32.0, 33.4 (CH2), 54.7, 58.8 (CH); LRMS (EI) m/z 225 (1%), 224 (2), 210 (6), 99 (17), 98 (100), 70 (6), 69 (5), 56 (7), 55 (11).
(2S,6S)-2-Methyl-6-nonylpiperidine [(+)-solenopsin] (4a). A flame-dried flask was cooled under a stream of argon and charged with Wilkinson catalyst (30 mg, 0.03 mmol) and a solution of enone 11 (207 mg, 0.60 mmol) in EtOH (2.0 mL). A balloon of H2 was connected to the flask and the reaction mixture was stirred at room temperature for 14 h. After removal of the solvent under vacuum, the residual brown oil was dissolved in 1:1 hexane/t-BuOMe and filtered through a short pad of Celite to remove the solid (Ph3P=O). The organic solution was concentrated under vacuum (15 Torr) and the crude product (>95% yield according to 1H NMR) was used in the next step without further purification. To a solution of protected amino ketone (198 mg, 0.57 mmol) in MeOH (1.8 mL) was added a 4M HCl solution in dioxane (0.82 mL) at 0-5 ºC and the reaction mixture was stirred over 1 h while the temperature increase to 23 ºC. After solvent evaporation under vacuum (15 Torr), the residue was dissolved in a saturated sodium bicarbonate aqueous solution (20 mL) and extracted with dichloromethane (2 × 30 mL). Organic phase was dried over potassium carbonate and concentrated (15 Torr) to afford 127 mg of imine 12 (0.56 mmol). To a solution of imine 12 (127 mg, 0.56 mmol) in THF (0.5 mL) a 2 M heptane solution of trimethylaluminium (1.2 mL, 2.40 mmol) was added at −78 ºC for 10 min. To the resulting reaction mixture, a 1 M lithium aluminum hydride THF solution (2.4 mL, 2.40 mmol) was slowly added and stirring was continued first for 1 hour at -78 ºC, then 1 hour at −45 ºC, 1 hour at −20 ºC and finally 1 h at 0 ºC. The reaction was carefully hydrolyzed by adding a 50% sodium potassium tartrate aqueous solution (Rochelle’s solution) until no more gas evolution occurred. To the resulting suspension was added 6 mL of the Rochelle’s solution and stirring was continued at room temperature for 14 h. The reaction mixture was diluted with water (20 mL), extracted first with diethyl ether (20 mL) and then with AcOEt (30 mL). Organics were washed with brine (10 mL), dried over potassium carbonate and concentrated (15 Torr) to afford 112 mg of the desired piperidine 4a (83%) as a pale yellow oil. GC-MS Rt (major-2S,6R) = 12.08 min (trans), Rt (minor-2R,6R) = 12.90 min (similar fragmentation). The corresponding hydrochloride was crystallized using ethereal HCl. Recrystallization from 1:3 EtOH/EtOAc afforded pure products (trans/cis > 98:2).
(+)-Solenopsin hydrochloride: White solid; (trans/cis > 98:2 according to GC-MS); mp 132-134 ºC; [α]20D +14.0 (c 1.00, CHCl3); IR ν (film) 2917, 2855, 2725, 1461, 1012 cm-1; 1H NMR (300 MHz, CDCl3) δ 0.88 (3H, t, J = 6.5, CH3), 1.19–1.41 (14H, m, 7 × CH2), 1.47 (3H, d, J = 6.7, CH3), 1.58–1.72 (5H, m, 2 × CH2, CHH), 1.86–2.08 (3H, m, CH2, CHH), 3.21–3.33 (1H, m, CHN), 3.47–3.59 (1H, m, CHN), 9.32 (2H, br s, NH2); 13C NMR (75 MHz, CDCl3) δ 14.26, 17.05 (CH3), 17.48, 22.82, 26.02, 26.28, 29.06, 29.43, 29.50, 29.67, 30.85, 32.01 (CH2), 48.06, 51.90 (CH); HRMS (EI) calcd for C15H31N 225.2457, found 225.2439.
ACKNOWLEDGEMENTS
This work was generously supported by the Spanish Ministerio de Ciencia e Innovación (Grant No. CTQ2007-65218, Consolider Ingenio 2010-CSD-2007-00006 and CTQ2011-24165), the Generalitat Valenciana (Grant No. PROMETEO/2009/039 and FEDER) and the University of Alicante. We also thank MEDALCHEMY S.L. for a gift of chemicals.
References
1. M. Schneider, Alkaloids: Chemical and Biological Perspectives, Vol. 10, ed. by S. W. Pelletier, Pergamon: Oxford, 1996, 55–299.
2. For reviews, see: (a) R. W. Bates and K. Sa-Ei, Tetrahedron, 2002, 58, 5957; CrossRef (b) M. G. P. Buffat, Tetrahedron, 2004, 60, 1701. CrossRef
3. A. B. Attygale, S. C. Xu, K. D. McCormick, J. Meinwald, C. L. Blankespoor, and T. Eismer, Tetrahedron, 1993, 49, 9333. CrossRef
4. M. W. Edwards and J. W. Daly, J. Nat. Prod., 1988, 51, 1188. CrossRef
5. S. Leclerq, I. Thirionet, F. Broeders, D. Daloze, R. Van der Meer, and J. C. Braeckman, Tetrahedron, 1994, 50, 8465. CrossRef
6. (a) P. Deslongchamps, Stereoelectronic Effects in Organic Chemistry; Pergamon: New York, 1983, Chap. 6; (b) R. V. Stevens, Acc. Chem. Res., 1984, 17, 289; CrossRef (c) D. M. Ryckman and R. V. Stevens, J. Org. Chem., 1987, 52, 4274. CrossRef
7. (a) Y. Matsumura, K. Maruoka, and H. Yamamoto, Tetrahedron Lett., 1982, 23, 1929; CrossRef (b) K. Maruoka, T. Miyazaki, M. Ando, Y. Matsumura, S. Sakane, K. Hattori, and H. Yamamoto, J. Am. Chem. Soc., 1983, 105, 2831. CrossRef
8. J. C. González-Gómez, M. Medjahdi, F. Foubelo, and M. Yus, J. Org. Chem., 2010, 75, 6308. CrossRef
9. For reviews, see: (a) S. J. Connon and S. Blechert, Angew. Chem. Int. Ed., 2003, 42, 1900; CrossRef (b) A. H. Hoveyda, D. G. Gillingham, J. J. Van Veldhuizen, O. Kataoka, S. B. Garber, J. S. Kingsburry, and J. P. A. Harrity, Org. Biomol. Chem., 2004, 2, 8. CrossRef
10. J. C. González-Gómez, F. Foubelo, and M. Yus, Synlett, 2008, 2777. CrossRef
11. A. Fürstner and K. Langemann, J. Am. Chem. Soc., 1997, 119, 9130. CrossRef
12. S. Fustero, S. Monteagudo, M. Sanchez-Rosello, S. Flores, P. Barrio, and C. del Pozo, Chem. Eur. J., 2010, 16, 9835. CrossRef
13. X. Wang, Y. Dong, J. Sun, X. Xu, R. Li, and Y. Hu, J. Org. Chem., 2005, 70, 1897. CrossRef
14. S. Ciblat, P. Besse, V. Papastergiou, H. Veschambre, J.-L. Canet, and Y. Troin, Tetrahedron: Asymmetry, 2000, 11, 2221. CrossRef