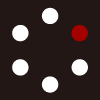
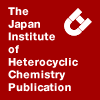
HETEROCYCLES
An International Journal for Reviews and Communications in Heterocyclic ChemistryWeb Edition ISSN: 1881-0942
Published online by The Japan Institute of Heterocyclic Chemistry
e-Journal
Full Text HTML
Received, 1st July, 2012, Accepted, 30th August, 2012, Published online, 11th September, 2012.
DOI: 10.3987/COM-12-S(N)85
■ Chemical Synthesis of Coelenterazine and Its Analogs: New Route toward Four Segment-Couplings
Chun-Ming Chou, Yu-Wen Tung, Meng-I Ling, Diana Chan, Wong Phakhodee, and Minoru Isobe*
Department of Chemistry, National Tsing Hua University, 101, Sec. 2, Kuang Fu Road, Hsinchu 30013, Taiwan, R.O.C.
Abstract
A novel and improved synthetic route includes four segment couplings toward coelenterazine and its analogs as luminescent molecules. Regio- and chemo-selective cross couplings using palladium catalysts with 5-iodo-3-bromo-2-aminopyrazine have enabled providing various aminopyrazine derivatives having different substituents. The improved synthesis of coelenterazine and its analogs employed advanced condensation with the aminopyrazines using various keto-ketal segments, which resulted in much higher yields to give the final imidazopyrazinone heterocycls than the previous method using keto-aldehydes.INTRODUCTION
Coelenterazine 1, as shown in Scheme 1, is a well-known chromophore involved in the marine bioluminescence substrate for the various different photoproteins. It (1) was first isolated and identified in 1960s. Later, Inoue et al. achieved the synthesis of coelenteramine 2 and coelenterazine from theacid-mediated condensation of two segments between a keto-aldehyde 3 and coelenteramine 2.1 This method was employed for many years without much improvement in the final step of acid-mediated condensation, which resulted in fair yields as 40-45%. But some efforts were paid for the segments synthesis. For example, our group reported that aminopyrazine, the precursor of coelenteramine, can be obtained from the condensation of N-Boc-glycine and aminonitrile hydrochloride and provided the new route based on cross-coupling between imino-tosylates and aryl-boronates to give various analogs.2 Maciej Adamczyk’s group developed a route from halogenated aminopyrazine to provide the Pd-mediated coupling precursor toward coelenteramine.3 Knochel’s group found the metal-halide exchange on pyrazine can yield the intermediate of coelenteramine through Negishi coupling reaction.4 Ohashi’s group5 and Knochel’s group4 both modified the Inoue’s method1c to generate α-bromoketone which can lead to keto-aldehyde. Based on the previous studies, the full method was well developed. However, we are looking for an even more efficient method to continue and supply modified chromophoric substrates for our own study on the bioluminescence of symplectin-photoprotein in Tobi-Ika (meaning flying squid in Japanese), a luminous oceanic squid (Symplectoteuthis oualaniensis). In our previous research, we found fluorinated analogs showed different performance in bioluminescence, for which we developed a different synthetic route using large amount of diazomethane.6 This method may not be suitable nowadays. In this article, we described a new synthetic route toward the keto-aldehyde-segment equivalents, and new synthetic intermediates of coelenterazine to make the analog synthesis efficiently.
RESULTS AND DISCUSSION
1. Novel synthetic route toward the keto-acetal
In the previous synthesis, α-bromoketone,1c,4 the precursor to yield ketoaldehyde, was prepared from diazomethane and phenylacetic acid. Diazomethane is a notorious explosive material in pure gas-form at room temperature, but it is almost universally used as a solution in diethyl ether. This compound is a popular methylating agent in chemical laboratory, but it is too hazardous to use in technical scales without special precautions. For the purpose of safety and simple route, we tried to design an alternative route without diazomethane. So we explored different approach to equivalent of ketoaldehyde 3 as described in the following sentence.
To obtain the ketoaldehyde 3, we tried several oxidative conditions of acetylene (Table 1) or 1,2-diol (Table 2), but we failed to obtain the target molecule. The oxidation of the olefinic precursors to α-hydroxyketone was not reproducible (Table 3). We concluded that uncontrollable over-oxidation or oxidative C-C bond cleavage is always associated because the enolization of the product ketone.
To avoid such enolization, we implemented different sequences to keto-acetal by addition to a carbonyl equivalent group rather than by oxidation. The Umpolung nucleophile 13 was prepared by the 1,3-propanedithiol protection of phenylacetaldehyde 12. The prepared nucleophile 13 can undergo Bouveault’s aldehyde formation7 together with the 2-benzyl-1,3-dithiane-2-carbaldehyde 14. Followed by acetal formation and deprotection of dithiane, the keto-acetal 4 was obtained in about 60% in total yield (Scheme 3). However, another target keto-acetal was not formed through the same pathway, but benzaldehyde 13e was obtained instead. Even a dianion nucleophile also led to the same result. To achieve our purpose, we change the method from aldehyde formation to ketone formation. We started the Weinreb amide 16b for the ketone formation from 2,4-difluorophenylacetic acid 6b rather than aldehyde or acyl halide to avoid oxidation or 2-times additions. The Umpolung nucleophile, precursor of aldehyde, underwent the ketone formation with amide 16c (Table 4).
As the result shown in Table 4, we can obtain various ketone 17b, 18b, 19b in 26~95% chemical yield. Two kinds of sulfide cleavage methods were applied to transform the dithioacetal 17b and 18b as well as the hemithioacetal 19b to dimethylacetal (Table 5). However, only the hemithioacetal 19b could be transformed to acetal 20b in the presence of copper(II) chloride and copper oxide in anhydrous methanol under reflux condition with 61% chemical yield. On the other hand, the acetal formation was not successful for other substrates (Scheme 4).
We were not satisfied with this result, so we kept improving the condition (Table 6). However, those other method did not provide good result. The α-sulfonyl ketone 24b, 24c were synthesized in good yield as equivalent as keto-acetal 20 (Scheme 5).
2. A precursor synthesis of coelenteramine analogs.
The synthesis of coelenteramine was reported for many years. There are two kinds of methods to yield coelenteramine. Kishi and Goto developed the first method in 1972 through the condensation of keto-oxime and aminonitrile. The other method was developed by Adamczyk et al.3,8 and Nakamura et al.9 through the palladium-meditated cross coupling on the commercially available 2-aminopyrazine. However, we would like to obtain the 8-position analogs of coelenterazine for our research interest. The method of Adamczyk et al. was modified to reach our goal.
We started from 2-aminopyrazine, which is commercially available. In route A, the mono-bromination product 26 was obtained from bromination of 2-aminopyrazine 25. Through the Suzuki-Miyaura coupling, the bromination, and further demethylation, the coelenteramine analog precursor 31 was obtained in 31% in 4 steps. On the other hand, we did iodination, which was immediately followed by bromination.
The halogenation product 29 can undergo selective Suzuki-Miyaura coupling on the 5-position rather than the 3-position of 2-aminopyrazine. Demethylation of the coupling product resulted in giving the precursor 31 with total yield 31%. The structure of 2-amino-5-iodopyrazine 28 was confirmed from the chemical shifts of the two protons at δ 7.81 and δ 8.19 ppm with small coupling constant (J = 1.4 Hz) as a long range coupling. The other evidence was in the fact that the same compound 30 was identified with those obtained from both routes A and B. The coelenteramine 2, 30, 31, 32 can be yielded through Negishi coupling8 or Suzuki-Miyaura coupling at the 3-position of the precursor 31.
3. Synthesis of coelenterazine
Since we developed several routes to yield keto-acetal and coelenteramine, we tried to synthesize coelenterazine itself by an acid-mediated condensation between keto-acetal and coelenteramine (Table 7). The obtained result shows that keto-acetal or keto-sulfone can react with coelenteramine 2 as better precursor to keto-aldehyde. In fact, these conditions have provided much higher yields (79~92%) than 63% (best results, but not repeatable) chemical yield from the condensation of keto-aldehyde and coelenteramine. And the keto-acetal is also suitable to undergo the condensation with other coelenteramine analog in good yields (Table 8).
EXPERIMENTAL
General
Proton NMR spectra were recorded on a Varian Mercury-400 for 400 MHz, or a Varian Unityinova-500 for 500 MHz. Chemical shift (δ) are given in parts per million relative to CD3OD (δ 3.30) or CDCl3 (δ 7.24) as internal standard. Coupling constants (J) are given in Hz. Carbon NMR spectra were recorded on Varian Mercury-400 for 100 MHz, or a Varian Unityinova-500 for 125 MHz. Chemical shifts are (δ) given in parts per million relative to CDCl3 (δ 77.0) or CD3OD (δ 49.0) as internal standard. Coupling constants (J) are given in Hz. Fluorine NMR spectra were recorded on a Varian Unityinova-500 for 470 MHz. Chemical shifts are (δ) given in parts per million relative to 1,1,1-trifluorotoluene (δ 0.00) as external standard. High-resolution (HR) MS spectra were measured with a Finnigan LCQ ion-trap mass spectrometer (Thermo Finnigan Co., San Jose, CA). All the solvents were of reagent grade. Analytical thin-layer chromatography (TLC) was conducted on precoated tlc plates: silica gel 60 F-254 [E. Merck (Art 5715) Darmstadt, Germany], layer thickness 0.25 mm. Silica gel column chromatography utilized Silica Gel 60 (spherical) 40-50 μm [E. Merck (Art 5715) Darmstadt, Germany].
2-(4-Methoxybenzyl)-1,3-dithiane (13a).
To a solution of 2-(4-methoxyphenyl)acetaldehyde (1.00 g, 6.66 mmol), 4Å MS (6.00 g) and 1,3-propanedithiol (0.80 mL, 7.98 mmol) in anhydrous CH2Cl2 (20.0 mL) under Argon at 0 °C, boron trifluoride diethyl etherate (3.30 mL, 26.6 mmol) was added. The reaction mixture was stirred for 2 h at 0 °C, and then was allowed to warm to room temperature and stirred for 12 h. The reaction mixture was quenched by NaHCO3(aq), filtered, and extracted with CH2Cl2 (10 mL × 3). The combined organic layer was dried over MgSO4 and evaporated under reduced pressure to dryness. The crude was purified by column chromatography (EtOAc:hexane = 1:8) to give 2-(4-methoxybenzyl)-1,3-dithiane as a yellow-green solid (1.455 g, 91%). 1H NMR (CDCl3, 400 MHz): δ 1.75-1.90 (m, 1H), 2.02-2.14 (m, 1H), 2.72-2.83 (m, 4H), 2.95 (d, 2H, J = 7.2 Hz), 3.76 (s, 1H), 4.19 (t, 1H, J = 7.2 Hz), 6.83 (d, 2H, J = 8.6 Hz), 7.15 (d, 2H, J = 8.6Hz). 13C NMR (CDCl3, 100 MHz): δ 25.5, 30.2, 40.6, 48.6, 54.8, 113.4, 129.0, 129.9, 158.2. HRMS (APCI) calcd for C12H16OS2 241.0715 (M+H+), found 241.0712 (M+H+).
2-(4-Methoxybenzyl)-1,3-dithiane-2-carbaldehyde (14a)
To a solution of 2-(4-methoxybenzyl)-1,3-dithiane (1.00 g, 4.16 mmol) in anhydrous THF (10.0 mL) at -20 °C, n-BuLi (2.5 M, 1.70 mL, 4.25 mmol) was added. After 30 min, anhydrous DMF (1.60 mL, 20.8 mmol) was added, and the reaction mixture was allowed to warm to room temperature and stirred for 6 h. The reaction was quenched by 10% HCl(aq) (30 mL), then extracted with EtOAc (20 mL × 3). The combined organic layer was dried over MgSO4 and evaporated under reduced pressure to dryness. The crude was purified by column chromatography (EtOAc : hexane = 1 : 8) to give 2-(4-methoxybenzyl)- 1,3-dithiane-2-carbaldehyde as a colorless oil (0.970 g, 87%). 1H NMR (CDCl3, 400 MHz): δ 1.65-1.81 (m, 1H), 1.98-2.11 (m, 1H), 2.53-2.63 (m, 2H), 2.89-3.02 (m, 2H), 3.08 (s, 2H), 3.75 (s, 3H), 6.80 (d, 2H, J = 8.8 Hz), 7.11 (d, 2H, J = 8.8 Hz), 9.20 (s, 1H). 13C NMR (CDCl3, 100 MHz): δ 24.1, 27.0, 41.5, 55.2, 58.7, 113.8, 125.1, 131.2, 159.1, 189.2. HRMS (APCI) calcd for C13H16O2S2 269.0664 (M+H+), found 269.0669 (M+H+).
2-(2-(4-Methoxybenzyl)-1,3-dithian-2-yl)-1,3-dioxolane (14a)
To a solution of 2-(4-methoxybenzyl)-1,3-dithiane-2-carbaldehyde (1.00 g, 3.73 mmol) in toluene (20.0 mL), ethylene glycol (1.0 mL, 17.9 mmol) and p-toluenesulfonic acid (6 mg, 1 mol%) was added. The reaction mixture was refluxed for 12 h under Dean-Stark conditions. The reaction was cooled to room temperature, quenched by NaHCO3(aq), and extracted with EtOAc (20 mL × 3). The combined organic layer was dried over MgSO4 and evaporated under reduced pressure to dryness. The crude was purified by column chromatography (EtOAc : hexane = 1 : 8) to give 2-(2-(4-methoxybenzyl)-1,3-dithian-2-yl)-1, 3-dioxolane as a colorless oil (0.955 g, 82%). 1H NMR (CDCl3, 400 MHz): δ 1.70-1.87 (m, 1H), 1.97-2.10 (m, 1H), 2.44-2.55 (m, 2H), 3.02 (s, 2H), 3.14-3.26 (m, 2H), 3.76 (s, 3H), 3.87-3.95 (m, 2H), 4.09-4.18 (m, 2H), 5.00 (s, 1H), 6.79 (d, 2H, J = 8.4 Hz, ArH), 7.27 (d, 2H, J = 8.4 Hz). 13C NMR (CDCl3, 100 MHz): δ 24.6, 27.1, 43.1, 53.3, 55.1, 65.6, 109.6, 113.1, 126.6, 132.1, 158.5. HRMS (APCI) calcd for C15H20O3S2 313.0927 (M+H+), found 313.0935 (M+H+).
1-(1,3-Dioxolan-2-yl)-2-(4-methoxyphenyl)ethanone (4a)
To a solution of 2-(2-(4-methoxybenzyl)-1,3-dithian-2-yl)-1,3-dioxolane (1.00 g, 3.20 mmol) in the mixed solvent of MeCN (100.0 mL) and water (35.0 mL), N-chlorosuccinimide (2.13 g, 16.0 mmol) and silver nitrate (2.72 g, 16.0 mmol) was added. The reaction solution was stirred for 5 min. The reaction was quenched by Na2SO3(aq) (40 mL), NaHCO3 (aq) (40 mL), and NaCl (aq) (40 mL), filtered, and extracted with EtOAc (100 mL × 3). The combined organic layer was dried over MgSO4 and evaporated under reduced pressure to dryness. The crude was purified by column chromatography (EtOAc : hexane = 1 : 5) to give 1-(1,3-dioxolan-2-yl)-2-(4-methoxyphenyl)ethanone as a colorless oil (0.647 g, 91%). 1H NMR (CDCl3, 400 MHz): δ 3.74-3.82 (m, 2H), 3.78 (s, 3H), 3.85-4.05 (m, 2H), 5.10 (s, 1H), 6.83 (d, 2H, J = 7.6 Hz, ArH), 7.10 (d, 2H, J = 7.6 Hz). 13C NMR (CDCl3, 100 MHz): δ 43.0, 55.1, 65.6, 101.5, 114.0, 124.9, 130.6, 158.6, 203.3. HRMS (APCI) calcd for C12H14O4 223.0965 (M+H+), found 223.0968 (M+H+).
2-(2,4-Difluorophenyl)-N-methoxy-N-methylacetamide (16b)
To a solution of 2,4-difluorophenylacetic acid (0.512 g, 2.98 mmol) in THF (5.0 mL) in ice bath under Ar, 1,1'-carbonyldiimidazole (0.580 g, 3.58 mmol) was add in portions and allowed to stir for 1 h. N,O-Dimethylhydroxylamine hydrochloride (0.32 g, 3.28 mmol) and N,N-diisopropylethylamine (0.55 mL, 3.16 mmol)were dissolved in DMF (2.0 mL). The DMF solution was injected to the solution of 2,4-difluorophenylacetic acid and allowed to stir for 24 h. The crude product was evaporated under reduced pressure to dryness, dissolved in EtOAc, washed with 1M HCl(aq), sat. NaHCO3(aq), and water, and then dried over MgSO4. The organic layer was evaporated under reduced pressure to dryness to give 2-(2,4-difluorophenyl)-N-methoxy-N-methylacetamide as light yellow liquid (0.609 g, 95%). 1H NMR (CDCl3, 400 MHz): δ 3.16 (s, 3H), 3.66 (s, 3H), 3.72 (s, 2H), 6.73-6.82 (m, 2H), 7.17-7.25 (m, 1H). 13C NMR (CDCl3, 100 MHz): δ 31.5, 32.2, 61.2, 103.5 (t, 2JC-F = 25.6 Hz), 110.0 (dd, 2JC-F = 20.9 Hz, 4JC-F = 3.9 Hz), 118.0 (dd, 2JC-F = 16.2 Hz, 4JC-F = 3.7 Hz), 132.1 (dd, 3JC-F = 9.6, 5.9 Hz), 160.8 (dd, 1JC-F = 248.0 Hz, 3JC-F = 11.8 Hz), 161.9 (dd, 1JC-F = 248.0 Hz, 3JC-F = 11.8 Hz), 171.0. 19F NMR (CDCl3, 470 MHz): -112.5, -113.7. HRMS (APCI) calcd for C10H11F2NO2 216.0831 (M+H+), found 216.0834 (M+H+).
2-(2,6-Difluorophenyl)-N-methoxy-N-methylacetamide (16c)
To a solution of 2,6-difluorophenylacetic acid (0.51 g, 2.98 mmol) in THF (5.0 mL) in ice bath under Ar, 1,1'-carbonyldiimidazole (0.58 g, 3.58 mmol) was add in portions and allowed to stir for 1h. N,O-Dimethylhydroxylamine hydrochloride (0.32 g, 3.28 mmol) and N,N-diisopropylethylamine (0.55 mL, 3.16 mmol) were dissolved in DMF (2.0 mL). The DMF solution was injected to the solution of 2,4-difluorophenylacetic acid and allowed to stir for 24h. The crude product was evaporated under reduced pressure to dryness, dissolved in EtOAc, washed with 1M HCl(aq), sat. NaHCO3(aq), and water, and then dried over MgSO4. The organic layer was evaporated under reduced pressure to dryness to give 2-(2,6-difluorophenyl)-N-methoxy-N-methylacetamide as colorless liquid (0.590 g, 92%). 1H NMR (CDCl3, 400 MHz): δ 3.13 (s, 3H), 3.65 (s, 3H), 3.77 (s, 2H), 6.76-6.84 (m, 2H), 7.09-7.17 (m, 1H). 13C NMR (CDCl3, 100 MHz): δ 25.9, 31.9, 60.8, 110.6 (dd, 2JC-F = 18.9 Hz, 4JC-F = 6.7 Hz), 111.0 (t, 2JC-F = 20.2 Hz), 128.3 (t, 3JC-F = 10.3 Hz), 161.3 (dd, 1JC-F = 247.5 Hz, 3JC-F = 7.5 Hz), 169.7. 19F NMR (CDCl3, 470 MHz): -115.1. HRMS (APCI) calcd for C10H11F2NO2 216.0831 (M+H+), found 216.0825 (M+H+).
3-(2,4-Difluorophenyl)-1-methoxy-1-(phenylthio)propan-2-one (19b)
To the solution of methoxymethyl phenyl sulfide (2.10 mL, 14.3 mmol) in THF (50 mL) at -78 °C, n-BuLi (5.8 mL, 2.5M, 14.5 mmol) was added and allowed to stir for 1 h. The prepared solution was added to the solution of 2-(2,4-difluorophenyl)-N-methoxy-N-methylacetamide (0.997 g, 4.64 mmol) in THF (50 mL) at -78 °C and allowed to stir for 0.5 h. The reaction solution was poured into NH4Cl(aq), extracted with EtOAc. The combined organic layer was dried over MgSO4 and evaporated under reduced pressure to dryness. The crude was purified by column chromatography (EtOAc : hexane = 1 : 6) to give 3-(2,4-difluorophenyl)-1-methoxy-1-(phenylthio)propan-2-one as a yellow color oil (1.358 g, 95%). 1H NMR (CDCl3, 400 MHz): δ 3.50 (d, J = 17.7 Hz, 1H, ABq), 3.61 (s, 3H), 3.88 (d, J = 17.7 Hz, 1H, ABq), 5.08 (s, 1H), 6.72-6.80 (m, 2H), 6.86-6.94 (m, 1H), 7.27-7.34 (m, 3H), 7.45-7.50 (m, 2H). 13C NMR (CDCl3, 125 MHz): δ 38.1, 56.2, 91.9, 103.6 (t, 2JC-F = 25.9 Hz), 111.1 (dd, 2JC-F = 21.0 Hz, 4JC-F = 3.8 Hz), 116.6 (dd, 2JC-F = 15.5 Hz, 4JC-F = 3.3 Hz), 128.8, 129.1, 130.5, 132.3 (dd, 3JC-F = 9.4, 6.1 Hz), 134.4, 161.0 (dd, 1JC-F = 248.0 Hz, 3JC-F = 12.2 Hz), 162.1 (dd, 1JC-F = 248.0 Hz, 3JC-F = 12.2 Hz), 198.7. 19F NMR (CDCl3, 470MHz): -112.6, -111.9. HRMS (APCI) calcd for C16H14F2O2S 309.0755 (M+H+), found 309.0742 (M+H+).
3-(2,6-Difluorophenyl)-1-methoxy-1-(phenylthio)propan-2-one (19c)
To the solution of methoxymethyl phenyl sulfide (2.10 mL, 14.3 mmol) in THF (50 mL) at -78 °C, n-BuLi (5.8 mL, 2.5M, 14.5 mmol) was added and allowed to stir for 1 h. The prepared solution was add to the solution of 2-(2,6-difluorophenyl)-N-methoxy-N-methylacetamide (0.997 g, 4.64 mmol) in THF (50 mL) at -78 °C and allowed to stir for 0.5 h. The reaction solution was poured into NH4Cl(aq), extracted with EtOAc. The combined organic layer was dried over MgSO4 and evaporated under reduced pressure to dryness. The crude was purified by column chromatography (EtOAc:hexane = 1:6) to give 3-(2,6-difluorophenyl)-1-methoxy-1-(phenylthio)propan-2-one as a yellow color oil (0.943 g, 66%). 1H NMR (CDCl3, 400 MHz): δ 3.59 (d, J = 18.2 Hz, 1H, ABq), 3.64 (s, 3H), 4.05 (d, J = 18.2 Hz, 1H, ABq), 5.10 (s, 1H), 6.80-6.88 (m, 2H), 7.14-7.23 (m, 1H), 7.28-7.34 (m, 3H), 7.47-7.52 (m, 2H). 13C NMR (CDCl3, 125 MHz): δ 32.5, 56.2, 91.8, 109.9 (t, 2JC-F = 20.6 Hz), 110.9 (dd, 2JC-F = 19.8 Hz, 4JC-F = 5.5 Hz), 128.7, 128.8 (t, 3JC-F = 10.3 Hz), 129.0, 130.4, 134.3, 161.4 (dd, 1JC-F = 247.8 Hz, 3JC-F = 8.3 Hz), 197.7. 19F NMR (CDCl3, 470 MHz): -114.3. HRMS (APCI) calcd for C16H14F2O2S 309.0755 (M+H+), found 309.0745 (M+H+).
3-(2,4-Difluorophenyl)-1,1-dimethoxypropan-2-one (20b)
To the solution of 3-(2,4-difluorophenyl)-1-methoxy-1-(phenylthio)propan-2-one (1.000 g, 3.23 mmol) in anhydrous MeOH (30 mL) under Ar, copper(II) chloride (0.653 g, 4.86 mmol) and copper oxide (0.773 g, 9.72 mmol) were added and allowed to stir. The reaction mixture was refluxed for 1.5 h, and then cooled to room temperature. The reaction crude mixture was filtered, evaporated under reduced pressure to dryness, dissolved in water, extracted with EtOAc. The combined organic layer was dried over MgSO4 and evaporated under reduced pressure to dryness. The crude was purified by column chromatography (EtOAc : hexane = 1 : 20) to give 3-(2,4-difluorophenyl)-1,1-dimethoxypropan-2-one as a yellow color oil (0.453 g, 61%). 1H NMR (CDCl3, 400 MHz): δ 3.43 (s, 6H), 3.86 (s, 2H), 4.54 (s, 1H), 6.76-6.85 (m, 2H), 7.06-7.14 (m, 1H). 13C NMR (CDCl3, 125 MHz): δ 36.9, 54.9, 103.7 (t, 2JC-F = 25.1 Hz), 104.0, 111.1 (dd, 2JC-F = 21.2 Hz, 4JC-F = 3.5 Hz), 116.7 (dd, 2JC-F = 17.0 Hz, 4JC-F = 3.7 Hz), 132.4 (dd, 3JC-F = 10.3, 5.9 Hz), 161.1 (dd, 1JC-F = 248.0 Hz, 3JC-F = 12.2 Hz), 162.2 (dd, 1JC-F = 248.0 Hz, 3JC-F = 12.2 Hz), 201.2. 19F NMR (CDCl3, 470 MHz): -113.1, -112.0. HRMS (APCI) calcd for C12H12F2O3 231.0827 (M+H+), found 231.0829 (M+H+).
3-(2,6-Difluorophenyl)-1-methoxy-1-(phenylsulfonyl)propan-2-one (24c)
To the solution of 3-(2,6-difluorophenyl)-1-methoxy-1-(phenylthio)propan-2-one (0.301 g, 0.98 mmol) in CH2Cl2 (9 mL) at -10 °C, meta-chloroperoxybenzoic acid (0.676 g, 3.92 mmol) and NaHCO3 (0.329 g, 3.92 mmol) were added and allowed to stir for 2 h. The reaction crude was poured into water, extracted with EtOAc. The combined organic layer was dried over MgSO4 and evaporated under reduced pressure to dryness. The crude was purified by column chromatography (EtOAc : hexane = 1 : 4) to give 3-(2,6-difluorophenyl)-1-methoxy-1-(phenylsulfonyl)propan-2-one as a white color solid (0.300 g, 90%). 1H NMR (CDCl3, 400 MHz): δ 3.71 (d, J = 18.9 Hz, 1H, ABq), 3.79 (s, 3H), 4.15 (d, J = 18.2 Hz, 1H, ABq), 4.83 (s, 1H), 6.80-6.88 (m, 2H), 7.16-7.25 (m, 1H), 7.53-7.59 (m, 2H), 7.65-7.71 (m, 1H), 7.85-7.90 (m, 2H). 13C NMR (CDCl3, 125 MHz): δ 34.1, 62.0, 100.0, 109.0 (t, 2JC-F = 20.6 Hz), 111.0 (dd, 2JC-F = 20.0 Hz, 4JC-F = 5.1 Hz), 128.2, 129.2, 129.7, 134.7 (t, 3JC-F = 220.6 Hz), 161.3 (dd, 1JC-F = 247.8 Hz, 3JC-F = 8.3 Hz), 169.9, 196.1. 19F NMR (CDCl3, 470 MHz): -114.4. HRMS (APCI) calcd for C16H14F2O4S 341.0654 (M+H+), found 341.3039 (M+H+).
5-Iodopyrazin-2-amine (28)
To a solution of pyrazin-2-amine (3.00 g, 3.16 mmol) in DMF (106 mL), was added NIS (13.6 g, 6.04 mmol). The mixture was stirred overnight. The reaction was diluted by addition of sat. Na2S2O3(aq) (100 mL), then extracted with EtOAc (150 mL × 3). The combined organic layer was washed with brine (150 mL) and dried over Mg2SO4, then concentrated under vacuum. The residual solid was purified by column chromatography (EtOAc : hexane = 1 : 3) to give 5-iodopyrazin-2-amine as a yellow solid (4.3 g, 61%). 1H NMR (CDCl3, 400 MHz), δ 4.59 (br s, 2H), 7.81 (d, 1H, J = 1.4 Hz), 8.19 (d, 1H, J = 1.4 Hz). 13C NMR (CDCl3, 100 MHz), δ 100.2, 133.5, 149.5, 153.6. HRMS (ESI) calcd for C4H4IN3 220.9450, found 220.9450 (M+).
3-Bromo-5-iodopyrazin-2-amine (29)
To a solution of 5-iodopyrazin-2-amine (2.00 g, 9.05 mmol) in DMF (43 mL), was added NBS (1.93 g, 10.86 mmol). The mixture was stirred for 2 h. Additional of NBS (0.81 g, 4.53 mmol) was added, and the resulting solution was stirred for 1 h. The reaction extracted with ether (150 mL × 3). The combined organic layer was washed with brine (150 mL) and dried over Mg2SO4, then concentrated under vacuum.
The residual solid was purified by column chromatography (EtOAc : hexane = 1 : 5) to give 3-bromo- 5-iodopyrazin-2-amine and 3,5-dibromopyrazin-2-amine as a yellow solid in a ratio 5:1 (2.0 g, 75%).
3-Bromo-5-(4-methoxyphenyl)pyrazin-2-amine (30)
To a solution of 3-bromo-5-iodopyrazin-2-amine (200 mg, 0.67 mmol) in degassed DME (3 mL), 4-methoxyphenylboronic acid (102 mg, 0.67 mmol), 10% Na2CO3 aq. (1.42 mL, 1.34 mmol) and Pd(PPh3)4 (39 mg, 0.034 mmol) were added, then the reaction mixture was refluxed for 1 day under nitrogen. The solution was cooled to room temperature and extracted with EtOAc (20 mL × 3). The combined organic layer was washed with brine (10 mL), and dried over MgSO4. After removal of the solvent, the residual solid was purified by column chromatography (EtOAc : hexane = 1 : 1) to give 3-bromo-5-(4-methoxyphenyl)pyrazin-2-amine as a yellow solid (126 mg, 67%). 1H NMR (CDCl3, 400 MHz), δ 3.53 (s, 3H), 4.99 (br s, 2H), 6.94 (d, 2H, J = 8.8 Hz), 7.78 (d, 2H, J = 8.8 Hz), 8.32 (s, 1H).
4-(5-Amino-6-bromopyrazin-2-yl)phenol (29)
A solution of the 3-bromo-5-(4-methoxyphenyl)pyrazin-2-amine (200mg, 0.71 mmol) in anhydrous CH2Cl2 (4 mL) was cooled to -78 °C and then BBr3 (0.14 mL, 1.42 mmol) was added. After the mixture was stirred for 1 h at -78 °C, the temperature was warmed to -30 °C, and then stirred for 5 h. The reaction was quenched by water (10 mL), and the CH2Cl2 was removed in vacuo. The aqueous solution was neutralized by NaHCO3 sat. aq., and then extracted with EtOAc (50 mL × 3). The combined organic layer was washed with brine (50 mL) and dried over MgSO4, then concentrated under vacuum. The residue was purified by column chromatography (EtOAc : hexane = 1 : 1) to give 19 as a yellow solid (100 mg, 53%). 1H NMR (CDCl3, 400 MHz) 4.98 (br s, 1H), 6.88 (d, 2H, J = 8.4 Hz), 7.74 (d, 2H, J = 8.8 Hz), 8.31 (s, 1H). 13C NMR (CD3COCD3, 125 MHz) 116.4, 125.6, 127.5, 128.2, 137.8, 142.8, 152.4, 158.6. HRMS (EI) calcd for C10H8BrN3O 264.9851, found 264.9846 (M+).
General procedure of synthesis of coelenterazine
To a solution of aminopyrazine (0.258 mmol) and keto-acetal (0.516 mmol) in degassed MeOH (3.0 mL) under Ar, 12N HCl (1.0 mL) was added and allowed to stir for 5 min. The reaction solution was heat to 80 °C for 4 h, and then cooled to room temperature. The crude product was diluted with water (100 mL), and filtered through Cosmosil. The collected fraction was purified by the crude mixture was purified by column chromatography (MeOH : CH2Cl2 = 1 : 20) to give coelenterazine as a solid.
8-Benzyl-2-(4-methoxybenzyl)-6-(4-methoxyphenyl)imidazo[1,2-a]pyrazin-3(7H)-one (33a)
1H NMR (400 MHz, CD3OD): δ 3.66 (3H, s), 3.71 (3H, s), 4.04 (2H, s), 4.32 (2H, s), 6.75 (2H, d, J = 8.7 Hz), 6.85 (2H, d, J = 8.7 Hz), 7.19-7.22 (5H, m, aromatic), 7.34-7.36 (3H, m), 7.40-7.43 (3H, m). 13C NMR (100 MHz, CD3OD): δ 31.59, 40.12, 55.61, 55.82, 108.05, 114.82, 115.31, 128.13, 129.07, 129.71, 129.77, 130.77, 131.74, 132.34, 137.99, 159.70, 162.10. HRMS (ESI) calcd for C28H25N3O3 451.1896, found 451.1896 (M+).
8-Benzyl-2-(2,4-difluorobenzyl)-6-(4-hydroxyphenyl)imidazo[1,2-a]pyrazin-3(7H)-one (1b)
1H NMR (400 MHz, CD3OD): δ 4.16 (2H, s), 4.37 (2H, s), 6.84-6.91 (2H, m), 6.91-6.96 (2H, m), 7.18-7.24 (1H, m), 7.24-7.33 (3H, m), 7.37 (2H, d, J = 8.4 Hz), 7.44 (2H, d, J = 8.4 Hz), 7.56 (1H, s). HRMS (APCI) calcd for C26H20F2N3O2 444.1508 (M+H+), found 444.1528 (M+H+).
8-Benzyl-2-(2,6-difluorobenzyl)-6-(4-hydroxyphenyl)imidazo[1,2-a]pyrazin-3(7H)-one (1c)
1H NMR (400 MHz, CD3OD): δ 4.20 (2H, s), 4.28 (2H, s), 6.83-6.87 (2H, m), 6.92-6.99 (2H, m), 7.15-7.24 (3H, m), 7.26-7.27 (1H, m), 7.31 (2H, d, J = 8.0 Hz), 7.39 (2H, d, J = 8.0 Hz), 7.47 (1H, s). HRMS (APCI) calcd for C26H20F2N3O2 444.1518 (M+H+), found 444.1511 (M+H+).
2-(2,4-Difluorobenzyl)-6-(4-hydroxyphenyl)-8-(naphthalen-2-yl)imidazo[1,2-a]pyrazin-3(7H)-one (33b)
1H NMR (400 MHz, CD3OD): δ 4.18 (2H, s), 6.80-6.96 (3H, m), 7.25-7.33 (1H, m), 7.24-7.33 (3H, m), 7.54-7.65 (2H, m), 7.73 (1H, d, J = 8.1 Hz), 7.84 (1H, s), 7.95 (1H, d, J = 9.2 Hz), 7.99-8.05 (1H, m), 8.17 (2H, d, J = 8.1 Hz), 8.65 (1H, s). HRMS (APCI) calcd for C29H20F2N3O2 480.1500 (M+H+), found 480.1518 (M+H+).
2-(2,4-Difluorobenzyl)-6-(4-hydroxyphenyl)-8-(4-methoxyphenyl)imidazo[1,2-a]pyrazin-3(7H)-one (34b)
1H NMR (400 MHz, CD3OD): δ 3.87 (3H, s), 4.14 (2H, s), 6.82-6.93 (4H, m), 7.07 (2H, d, J = 8.6 Hz), 7.27 (1H, q, J = 8.5 Hz), 7.61 (2H, d, J = 8.6 Hz), 7.67 (1H, br s), 8.02 (2H, d, J = 8.6 Hz). HRMS (APCI): calcd for C26H18F2N3O3 (M-H+) 458.1311; found 458.1302.
References
1. (a) T. Goto, H. Iio, S. Inoue, and H. Kakoi, Tetrahedron Lett., 1974, 15, 2321; CrossRef (b) Y. Kishi, H. Tanino, and T. Goto, Tetrahedron Lett., 1972, 13, 2747; CrossRef (c) S. Inoue, S. Sugiura, H. Kakoi, K. Hasizume, T. Goto, and H. Iio, Chem. Lett., 1975, 141. CrossRef
2. (a) M. Isobe, M. Kuse, Y. Yasuda, and H. Takahashi, Bioorg. Med. Chem. Lett., 1998, 8, 2919; CrossRef (b) M. Kuse and M. Isobe, Tetrahedron, 2000, 56, 2629; CrossRef (c) M. Kuse, N. Kondo, Y. Ohyabu, and M. Isobe, Tetrahedron, 2004, 60, 835; CrossRef (d) N. Kondo, M. Kuse, T. Mutarapat, N. Thasana, and M. Isobe, Heterocycles, 2005, 65, 843; CrossRef (e) M. Kuse, I. Doi, N. Kondo, Y. Kageyama, and M. Isobe, Tetrahedron, 2005, 61, 5754; CrossRef (f) A. Makarasen, M. Kuse, T. Nishikawa, and M. Isobe, Bull. Chem. Soc. Jpn., 2009, 82, 870; CrossRef (g) W. Phakhodee, M. Toyoda, C.-M. Chou, N. Khunnawutmanotham, and M. Isobe, Tetrahedron, 2011, 67, 1150. CrossRef
3. M. Adamczyk, S. R. Akireddy, D. D. Johnson, P. G. Mattingly, Y. Pan, and R. E. Reddy, Tetrahedron, 2003, 59, 8129. CrossRef
4. M. Mosrin, T. Bresser, and P. Knochel, Org. Lett., 2009, 11, 3406. CrossRef
5. F. Q. Chen, J. L. Zheng, T. Hirano, H. Niwa, Y. Ohmiyab, and M. Ohashi, J. Chem. Soc., Perkin Trans. 1, 1995, 2129. CrossRef
6. (a) M. Isobe, T. Fujii, M. Kuse, K. Miyamoto, and K. Koga, Tetrahedron, 2002, 58, 2117; CrossRef (b) V. Kongjinda, Y. Nakashima, N. Tani, M. Kuse, T. Nishikawa, C.-H. Yu, N. Harada, and M. Isobe, Chem. Asian J., 2011, 6, 2080. CrossRef
7. L. I. Smith and J. Nichols, J. Org. Chem., 1941, 06, 489. CrossRef
8. (a) E. Negishi, L. F. Valente, and M. Kobayashi, J. Am. Chem. Soc., 1980, 102, 3298; CrossRef (b) M. Kobayashi and E. Negishi, J. Org. Chem., 1980, 45, 5223; CrossRef (c) E. Negishi, Acc. Chem. Res., 1982, 15, 340. CrossRef
9. (a) C. Wu, K. Kawasaki, S. Ohgiya, and Y. Ohmiya, Tetrahedron Lett., 2006, 47, 753; CrossRef (b) H. Nakamura, D. Takeuchi, and A. Murai, Synlett, 1995, 1227. CrossRef