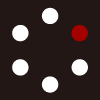
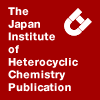
HETEROCYCLES
An International Journal for Reviews and Communications in Heterocyclic ChemistryWeb Edition ISSN: 1881-0942
Published online by The Japan Institute of Heterocyclic Chemistry
e-Journal
Full Text HTML
Received, 24th July, 2012, Accepted, 20th September, 2012, Published online, 2nd October, 2012.
DOI: 10.3987/COM-12-S(N)102
■ Tuning the Solubilities of Bis-triazinylphenanthroline Ligands (BTPhens) and Their Complexes
Dominic M. Laventine, Ashfaq Afsar, Michael J. Hudson, and Laurence M. Harwood*
Department of Chemistry, University of Reading, Reading RG6 6AD, U.K.
Abstract
A series of bis-triazinylphenanthroline ligands (BTPhens) was synthesized by modifying the triazine substituents. It was found that varying these substituents altered the solubilities of the ligands in a number of non-polar solvents. Thus C5-BTPhen showed significantly higher solubility in octanol than C1-BTPhen. The high solubility of C5-BTPhen and its complexes was exploited to facilitate the NMR titration experiments. These experiments shown that the dominant species in solution were the 1:2 complexes [Ln(III)(BTPhen)2], even at high Ln concentrations, and that the relative stability of the 2:1 to 1:1 BTPhen-Ln complexes varied with different lanthanides. C5-BTPhen therefore shows considerable promise for a once-through selective actinide separation process.INTRODUCTION
Heterocyclic molecules are likely to be the principal molecules in some future separation processes in the nuclear fuel cycle. Currently, the separation of minor actinides(III) from lanthanides(III) is still receiving much attention. This separation of actinides(III) from lanthanides(III) is particularly difficult owing to the chemical similarities between the two groups of elements.1 However, it is thought that the greater availability of the valence orbitals in the actinides means that there is a more covalent contribution to metal-ligand bonding than with the lanthanides.2 It has been shown that 1,2,4 tridentate N-donor ligands can exploit this small but significant difference between the actinides and lanthanides.2 However, in addition to forming complexes selectively with actinides(III) rather than lanthanides(III), the ligands must have a sufficiently high solubility in the non-polar organic diluents that are used in the nuclear industry, such as TPH (similar to dodecane) and octanol. Typically the reprocessing is carried out in 3M nitric acid under (sometimes intense) irradiation and consequently molecules must show acceptable resistance towards both acidic hydrolysis and radiolysis, and any degradation products that form must not interfere with the extraction. It is also desirable that the ligand be composed of only carbon, hydrogen, oxygen and nitrogen (CHON principle) so that the ligand or its degradation products can be completely incinerated at the end of its useful life, thereby avoiding secondary waste generation. The development of N-heterocyclic ligands which are capable of fulfilling all these criteria has thus been the subject of intense research, which has recently been reviewed.1,2
Two classes of 1,2,4-triazines emerging from previous research are the (2,6-bistriazinyl)pyridines (BTP, 1)3 and the 6,6-bistriazinyl-2,2-bipyridines (BTBP, 2).4 CyMe4-BTBP is currently the benchmark molecules for the SANEX (Selective Actinide Extraction) process. Experience with BTP and BTBP ligands has shown that it is beneficial to reduce the number of atoms in the ligand to achieve faster kinetics of extraction; whereas long aliphatic groups can provide the required solubility. The CyMe4 group has been found to be resistant to radiolysis, and provides a compromise between acceptable solubility and sufficiently high kinetics. There is a current view that molecules may be used in once-through processes and so resistance to radiolysis becomes less of a key feature in the design of the molecules. We have developed a class of ligands that replace the bipyridine skeleton with a 1,10-bisphenanthroline analogue (BTPhen, e.g. 3). By tethering the pyridine rings in this way, the molecule is held in the cis-conformation, removing the requirement for a conformational rearrangement prior to complexation.5 In addition, phenanthrolines have larger dipole moments than the 2,2’-bipyridines,6 and are known to coordinate strongly to water via hydrogen bonding,7 suggesting that they would interact more favourably with the organic/water interface. These changes have improved the kinetics of extraction compared to the corresponding BTBP while maintaining the high selectivity.
To date, CyMe4-BTPhen 3 is the only reported example of this class of ligand. Herein, we describe the synthesis of a number of examples of the BTPhen class of ligands with a variety of alkyl and aryl substituents on the triazine rings. Such modification can be simply achieved in the final step of the synthesis, allowing the solubilities of the ligands to be fine-tuned and solution phase characterization to be undertaken. A variety of substituents (including branched and cyclic aliphatic groups and aromatic groups) was chosen, in order to increase our understanding of the effect of substituent size on solubility and kinetics of extraction.
RESULTS AND DISCUSSION
The substituted BTPhen ligands were synthesized as shown in Scheme 1. The key intermediate, dihydrazide 8 was prepared from 2,9-dimethyl-1,10-phenanthroline 4 through a modification of our previously reported procedures. As in our previously reported method, dioxime 6 was produced by treatment of dialdehyde 5 with hydroxylamine hydrochloride, however, this intermediate was then isolated, and elimination achieved by treatment with p-toluenesulfonyl chloride and pyridine to afford the dinitrile 7. The reaction of the dinitrile 7 with hydrazine hydrate in EtOH gave the diamide dihydrazide 8 in near quantitative yield (40% overall from neocuproine 4).
The condensation of 8 was undertaken with commercially available and synthesized symmetrical 1,2-diketones. The synthesized diketones were produced by acyloin condensation ation of the appropriate ethyl ester followed by oxidation. The condensation reactions were performed in THF or dioxane, depending on the solubility of the diketone, under reflux in the presence of triethylamine (Et3N). The novel 2,9-bis(1,2,4-triazin-3-yl)-1,10-phenanthroline ligands (BTPhens) 9a-g were obtained in moderate to good yields as a yellow-orange solids and their solubilities in various industrially relevant solvents were assessed (see Table 1).
The choice of diluents used in solvent extraction processes is constrained to those used in the nuclear industry. Solvents commonly include long chain hydrocarbons such as dodecane, alcohols such as octanol and cyclohexanone.8 The solubilities of the BTPhen ligands, in all solvents tested, increased as chain length was increased. These results bode well for the use of this class of ligands in solvent extractions.
The improved solubilities achieved also allowed solution characterization of the ligands and the complexes formed with metal salts. NMR titrations of the ligands with a number of lanthanides (La, Eu, Lu) salts were performed in deuterated acetonitrile (CD3CN). Lanthanum (La) and Lutetium (Lu) were chosen to give the greatest difference in atomic weight. Higher atomic weight lanthanides have been used as analogues for actinides in selective extractions from lower weight lanthanides.9,10 The results of the NMR titrations indicated that the ligands initially formed only 2:1 ligand-metal complexes in solution, as conversion to a single complex was complete after addition of 0.5 equivalents of metal salt for all lanthanides tested. Subsequent formation of 1:1 complexes was observed upon addition of excess metal salt (see Figures 1.1-2.2), although in all cases, the majority of the BTPhen remained bound in 2:1 complexes even at excess levels (3 equivalents) of Ln. In the case of the lanthanum complex, formation of the 1:1 complex occurred to a greater degree for a given metal concentrations, compared to the corresponding lutetium complex. These results show that the relative thermodynamic stability of 2:1 complex compared to the 1:1 complex is greater for lutetium than for the lanthanum complexes. It has been postulated that the 2:1 complex is the actual extracting species owing to the metal center perhaps being completely enclosed by the hydrophobic shell formed by the two ligands. Hence the more relatively stable 2:1 complex would be more efficiently extracted, giving rise to the selectivity of solution phase extractions.
Assessment of these ligands partition coefficients and separation factors is currently being undertaken. Studies are also ongoing into the introduction of hydrophilic chains onto the triazine rings, producing novel water-soluble ligands that would allow reverse extraction or act as masking agents.
EXPERIMENTAL
All reagents were either supplied by Acros, Aldrich, Fluka or Lancaster fine chemical suppliers, and were used as supplied. Diethyl ether (Et2O), toluene and tetrahydrofuran (THF) were dried by refluxing over and distillation from sodium benzophenone ketyl under nitrogen.
All 1H and 13C NMR spectra were recorded using either a Bruker AMX400 or an Avance DFX400 nstrument in CDCl3 or dimethyl sulfoxide-d6 (DMSO-d6). Chemical shifts (δ) are reported in parts per million (ppm) with the abbreviations s, d, t, q, dd and br denoting singlet, doublet, triplet, quartet, double doublet and broad resonances respectively. Coupling constants (J) are quoted in Hertz. IR spectra were recorded as Nujol® mulls (N) on a Perkin Elmer RX1 FT-IR instrument. Melting points were determined on a Gallenkamp melting point apparatus. Mass spectra (m/z) were recorded by electrospray ionisation(ESI) on an Xcalibur Tune 2.1 (SP1) as [MH]+, [M + Na]+ or [2M + Na]+.
Synthesis of 1,10-Phenanthroline-2,9-dicarbaldehyde (5).
Selenium dioxide (11.62 g, 104.7 mmol, 2.1 eq.) dissolved in dioxane (250 mL) and water (~ 7mL) was heated to reflux. To this solution was added a solution of 2,9-dimethyl-1,10-phenanthroline 4 (10.35 g, 49.7 mmol) in dioxane (250 mL) dropwise over 35 min. The solution was heated under reflux for 30 min. The reaction mixture was transferred while hot and allowed to cool overnight. The solid that separated was filtered and washed with Et2O (5 × 50 mL) and dried in a vacuum oven (40 oC) to afford dialdehyde 5 as a pale brown solid (10.92 g, 93%).
δH (CDCl3) = 8.05 (s, 2H), 8.39 (d, J = 8.2 Hz, 2H), 8.52 (d, J = 8.2 Hz, 2H), 10.57 (s, 2H). δC (DMSO-d6) = 120.0, 129.1, 131.3, 138.3, 145.1, 152.1, 193.6 ppm. C14H8N2O2 [M + Na] requires m/z 259.0478; (FTMS + p ESI) MS found m/z 259.0843; IR vmax/cm-1 = 3351, 3159, 1695 (C=O), 1573, 1491, 1365.
Synthesis of 1,10-Phenanthroline-2,9-dicarbonitrile (7)
To a suspension of 1,10-phenanthroline-2,9-dicarbaldehyde 5 (14.02 g, 59.3 mmol) in dry MeCN (600 mL) was added hydroxylamine hydrochloride (8.94 g, 128.7 mmol, 2.2eq) and Et3N (50 mL, 358.5 mmol, 6 eq). After heating the solution under reflux for 4 h, the mixture was allowed to cool to room temperature. p-Toluenesulfonyl chloride (35.99 g, 188.9 mmol, 3.3 eq) and pyridine (30 mL, 372.7 mmol, 6.3 eq) were added and the mixture was heated under reflux for 24 h. The mixture was filtered while hot and the solid residue was washed with hot MeCN (50 mL). The filtrate was evaporated to afford a brown semi-solid which was triturated with MeOH (200 mL), then filtered and washed with MeOH (200 mL) and Et2O (200 mL) to afford 7 as a pale brown solid (8.25 g, 60%).
δH (DMSO-d6) = 8.28 (s, 2H), 8.41 (d, J = 8.2 Hz, 2H), 8.83 (d, J = 8.2 Hz, 2H) ppm. δC (DMSO-d6) = 117.6, 127.6, 129.2, 130.5, 132.9, 138.6, 144.8 ppm. C14H6N4 [MH]+ requires m/z 231.0665; (FTMS + p ESI) MS found m/z 231.0661; IR vmax/cm-1 = 3086, 3063, 2238 (C≡N), 1621, 1500, 1368.
Synthesis of 1,10-Phenanthroline-2,9-dicarbohydrazonamide (8)
To a suspension of 1,10-phenanthroline-2,9-dicarbonitrile 7 (4.17 g, 18.1 mmol) in EtOH (250 mL) was added hydrazine hydrate (150 mL, 64%). The suspension was stirred at room temperature for 6 days. After concentrating the mixture under reduced pressure, Et2O (200 mL) was added and the precipitated solid was filtered and allowed to dry in the air overnight to afford 8 as a brown solid (4.51 g, 85%).
δH (DMSO-d6) = 5.66 (br s, 4H), 6.12 (br s, 4H), 7.94 (s, 2H), 8.28 (d, J = 8.5 Hz, 2H), 8.37 (d, J = 8.5 Hz, 2H) ppm. δC (DMSO-d6) = 118.9, 125.9, 128.1, 136, 143.3, 143.4, 151.2 ppm. C14H14N8 [MH]+ requires m/z 295.1414; (FTMS + p ESI) MS found m/z 295.1412; IR vmax/cm-1 = 3326 (N-H), 3174 (N-H), 3043 (N-H), 1619, 1497, 1128.
Synthesis of 2,9-Bis(dimethyl-1,2,4-triazin-3-yl)-1,10-phenanthroline (Me2-BTPhen, 9a)
To a suspension of 1,10-phenanthroline-2,9-dicarbohydrazonamide 8 (0.95 g, 3.2 mmol) in THF (60 mL) was added 2,3-butanedione (0.60 mL, 6.9 mmol, 2.2 eq). Et3N (6 mL, 42.7 mmol) was added and the mixture was heated under reflux for 24 h. After allowing the solution to cool to room temperature, the solvent was evaporated and the remaining solid residue was triturated with Et2O (20 mL). The insoluble solid was filtered and washed with MeOH (10 mL) and Et2O (20 mL) and allowed to dry in a vacuum oven (40 oC) to afford the ligand 9a as a pale brown solid (1.02 g, 79%).
mp 218-221 °C; δH (CDCl3) = 2.74 (s, 6H), 2.77 (s, 6H), 8.16 (s, 2H), 8.71 (d, 2H), 8.71 (d, 2H). δC (CDCl3) = 19.3, 21.7, 122.3, 127.7, 129.4, 137.9, 144.6, 151.7, 157.6, 160, 160.2 ppm. C22H18N8 [MH]+ requires m/z 395.1727; (FTMS + p ESI) MS found m/z 395.1723; IR vmax/cm-1 = 3516, 3407, 3174, 2985 (C-H), 1626, 1528, 1497, 1449, 1394, 1371, 1166.
Synthesis of 2,9-Bis(dimethyl-1,2,4-triazin-3-yl)-1,10-phenanthroline (n-C4-BTPhen, 9b)
To a suspension of 1,10-phenanthroline-2,9-dicarbohydrazonamide 8 (1.00 g, 3.4 mmol) in THF (100 mL) was added decane-5,6-dione (1.33 g, 7.8 mmol, 2.3 eq). Et3N (6 mL, 42.6 mmol) was added and the mixture was heated under reflux for 3 days. The solution was allowed to cool to room temperature and filtered and the remaining solid residue was washed with DCM (50 mL). The filtrate was evaporated and the solid was triturated with ice-cold Et2O (200 mL). The insoluble solid was filtered and washed with ice-cold Et2O (200 mL) and allowed to dry in air to afford the ligand 9b as an orange solid (0.61 g, 62%). mp 147-150 °C; δH (CDCl3) = 1.00 (m, 12H), 1.55 (m, 8H), 1.92 (m, 8H), 3.13 (m, 4H), 7.96 (s, 2H), 8.49 (d, J = 8.4 Hz, 2H), 8.94 (d, J = 8.4 Hz). δC (CDCl3) = 13.9, 22.6, 22.9, 30.4, 30.6, 32.2, 33.9, 123.1, 127.7, 130.0, 137.4, 146.6, 153.4, 160.1, 161.4, 162.7. C34H43N8 [MH]+ requires m/z 563.3611; (FTMS + p ESI) MS found m/z 563.3616; IR vmax/cm-1 = 3511, 2955 (C-H), 2930 (C-H), 2871 (C-H), 2676, 1621, 1586, 1519, 1497, 1443, 1383.
Synthesis of 2,9-Bis(dimethyl-1,2,4-triazin-3-yl)-1,10-phenanthroline (s-C4-BTPhen, 9c).
To a suspension of 1,10-phenanthroline-2,9-dicarbohydrazonamide 8 (0.56 g, 1.9 mmol) in THF (50 mL) was added 3,6-dimethyloctane-4,5-dione (1.33 g, 7.8 mmol, 2.3 eq). Et3N (1.5 mL, 10.7 mmol) was added and the mixture was heated under reflux for 7 days. The solution was allowed to cool to room temperature and filtered and the remaining solid residue was washed with DCM (25 mL). The filtrate was evaporated and the solid was triturated with Et2O (100 mL). The insoluble solid was filtered and washed with Et2O (100 mL) and allowed to dry in air to afford the ligand 9c as a brown solid (0.241 g, 23%). NMR analysis indicated that 9c was obtained as a mixture of diastereoisomers.
mp 148-151 °C; δH (CDCl3) = 0.95 (m, 12H), 1.46 (m, 12H), 1.86 (m, 4H), 2.1 (m, 4H), 3.24 (m, 4H), 7.96 (m, 2H), 8.48 (m, 2H), 8.96 (m, 2H) ppm. δC (CDCl3) = 12.2, 12.4, 19.4, 20.5, 28.7, 29.5, 36.8, 37.7, 122.7, 123.2, 126.6, 127.7, 129.6, 129.8, 137.4, 163.1, 165.3 ppm. C34H43N8 [MH]+ requires m/z 563.3611; (FTMS + p ESI) MS found m/z 563.3612; IR vmax/cm-1 = 3361, 2963 (C-H), 2873 (C-H), 1615, 1495, 1381.
Synthesis of 2,9-bis(5,6-dipentyl-1,2,4-triazin-3-yl)-1,10-phenanthroline (C5-BTPhen, 9d)
To a suspension of 1,10-phenanthroline-2,9-dicarbohydrazonamide 8 (0.50 g, 1.7 mmol) in THF (50 mL) was added dodecane-6,7-dione (0.76 g, 3.8 mmol, 2.2 eq). Et3N (3 mL, 21.3 mmol) was added and the mixture was heated under reflux for 3 days. . After allowing the solution to cool to room temperature, the solvent was evaporated and the remaining semi-solid residue was triturated with ice-cold Et2O (100 mL). The insoluble solid was filtered and washed with ice-cold Et2O (100 mL) and allowed to dry in air to afford the ligand 9d as a yellow solid (0.27 g, 35%).
mp 138-141 °C; δH (CDCl3) = 0.94 (m, 12H, 1.45 (m, 16H), 1.90 (m, 8H), 3.07 (t, J = 8.7 Hz, 4H), 3.13 (t, J = 8.7 Hz, 4H), 7.96 (s, 2H), 8.45 (d, J = 8.3 Hz, 2H), 8.93 (d, J = 8.3 Hz, 2H) ppm. δC (CDCl3) = 14.0, 14.1, 22.5, 22.5, 28.2, 28.3, 31.7, 32.1, 32.4, 34.4, 123.1, 127.5, 129.7, 137.2, 146.6, 153.6, 160.0, 161.6, 162.6. C76H100N16 [M + Na] requires m/z 1259.8209; (FTMS + c ESI) MS found m/z 1259.8235; IR vmax/cm-1 = 3511, 2956 (C-H), 2926 (C-H), 2858 (C-H), 2674, 2490, 1622, 1585, 1518, 1496.
Synthesis of 2,9-Bis(dimethyl-1,2,4-triazin-3-yl)-1,10-phenanthroline (Camphor-BTPhen, 9e)
To a suspension of 1,10-phenanthroline-2,9-dicarbohydrazonamide 8 (0.98 g, 3.3 mmol) in THF (50 mL) was added camphor-quinone (1,21 g, 7.26 mmol, 2.2 eq). Et3N (6 mL, 42.7 mmol) was added and the mixture was heated under reflux for 24 h. After allowing the solution to cool to room temperature, the solvent was evaporated and the remaining solid residue was triturated with Et2O (20 mL). After cooling to 0 °C in an ice-bath, the insoluble solid was filtered and washed with Et2O (20 mL) and allowed to dry in a vacuum oven (40 oC) to afford a mixture of three regioisomers of ligand 9e as a pale brown solid (0.49 g, 39%).
mp 208-213 °C; C22H18N8 [MH]+ requires m/z 395.1727; (FTMS + p ESI) MS found m/z 395.1723; IR vmax/cm-1 = 3450, 2960 (C-H), 2875 (C-H), 1754, 1594, 1520.
Synthesis of 2,9-Bis(diphenyl-1,2,4-triazin-3-yl)-1,10-phenanthroline (Ph2-BTPhen, 9f)
To a suspension of 1,10-phenanthroline-2,9-dicarbohydrazonamide 8 (0.50 g, 1.7 mmol) in dioxane (50 mL) was added diphenylethane-1,2-dione (0.86 g, 4.1 mmol, 2.4 eq). Et3N (3 mL, 21.3 mmol) was added and the mixture was heated under reflux for 3 days. The solution was allowed to cool to room temperature and filtered and the remaining solid residue was washed with DCM (50 mL). The filtrate was evaporated and the solid was triturated with Et2O (100 mL). The insoluble solid was filtered and washed with Et2O (100 mL) and allowed to dry in a vacuum oven (40 oC) to afford the ligand 9f as a pale yellow solid (0.65 g, 59%).
mp 270-273 °C; δH (CDCl3) = 7.41 (m, 12H), 7.72 (d, J = 6.8 Hz, 4H), 7.91 (d, J = 6.8 Hz, 4H), 7.99 (s, 2H), 8.52 (d, J = 8.3 Hz, 2H), 8.99 (d, J = 8.3 Hz, 2H) ppm. δC (CDCl3) = 123.2, 127.7, 128.6, 128.7, 129.6, 129.9, 130.4, 135.5, 137.4, 138.3, 146.5, 152.9, 156.1, 156.4, 160.8 ppm. C84H52N16 [M + Na] requires m/z 1307.4453; (FTMS + c ESI) MS found m/z 1307.4460; IR vmax/cm-1 = 3054, 1581, 1492, 1444, 1379, 1361, 1215, 1181, 1147, 1102.
Synthesis of 2,9-Bis(dimethyl-1,2,4-triazin-3-yl)-1,10-phenanthroline (BrPh-BTPhen, 9g)
To a suspension of 1,10-phenanthroline-2,9-dicarbohydrazonamide 8 (0.26 g, 0.9 mmol) in THF (50 mL) was added 1,2-bis(4-bromophenyl)ethane-1,2-dione (0.75 g, 2.1 mmol, 2.3 eq). Et3N (1.5 mL, 10.7 mmol) was added and the mixture was heated under reflux for 2 days. The solution was allowed to cool to room temperature and filtered and the remaining solid residue was washed with DCM (25 mL). The filtrate was evaporated and the solid was triturated with Et2O (100 mL). The insoluble solid was filtered and washed with MeOH (10 mL) and Et2O (100 mL) and allowed to dry in air to afford the ligand 9g as a pale brown solid (0.51 g, 61%).
mp 312-315 °C; δH (CDCl3) = 7.54-7.68 (m, 8H), 7.81-7.87 (m, 8H), 7.99 (s, 2H), 8.54 (d, J = 8.3 Hz, 2H), 9.02 (d, J = 8.3 Hz, 2H) ppm. δC (CDCl3) = 123.1, 124.9, 126.2, 127.8, 129.9, 131.1, 132.2, 132.3, 134.4, 137.4, 146.5, 152.4, 154.7, 155.2, 160.7 ppm. C42H23Br4N8 [MH]+ requires m/z 954.8779; (FTMS + p ESI) MS found m/z 954.8779; IR vmax/cm-1 = 1662, 1587, 1485, 1380, 1170, 1109.
General procedure for NMR titrations of C5-BTPhen, 9d.
A 0.5 mL, 0.01 M solution of C5-BTPhen (9d, 5 µmol) in CD3CN was made up in an NMR tube. A 0.01 M solution of Ln(NO3)3∙xH2O was added in 25-50 µL aliquots (0.25-0.5 µmol per aliquot) and the 1H NMR spectrum was recorded after each addition.
C5-BTPhen: δH (CD3CN) = 0.94 (t, J = 6.9 Hz, 6H), 0.95 (t, J = 6.9 Hz , 6H) 1.46 (m, 16H), 1.96 (quint, J = 4.9 Hz, 8H), 3.00 (t, J = 7.3 Hz, 4H), 3.10 (t, J = 7.3 Hz, 4H), 8.09 (s, 2H), 8.62 (d, J = 8.4 Hz), 8.81 (d, J = 8.4 Hz, 2H) ppm.
La(C5-BTPhen)2(NO3)x: δH (CD3CN) = 0.62 (quint, J = 7.4 Hz, 4H), 0.70 (t, J = 7.2 Hz, 6H), 0.80 (quint, J = 7.4 Hz, 4H), 0.89 (t, J = 7.2 Hz, 6H), 0.93 (quint, J = 7.4 Hz, 4H), 1.33 (m, 8H), 1.76 (quint, J = 7.4 Hz, 4H), 2.39 (t, J = 7.3 Hz, 4H), 2.74 (t, J = 7.3 Hz, 4H), 8.49 (s, 2H), 9.04 (d, J = 8.4 Hz, 2H), 9.11 (d, J = 8.4 Hz, 2H) ppm.
Ln(C5-BTPhen)2(NO3)x: δH (CD3CN) = 0.52 (quint, J = 7.4 Hz, 4H), 0.79 (t, J = 7.2 Hz, 6H), 0.80-0.87 (m, 10H), 1.06 (quint, J = 7.4 Hz, 4H), 1.30 (m, 8H), 1.69 (quint, J = 7.4 Hz, 4H), 2.28 (t, J = 7.3 Hz, 4H), 2.72 (t, J = 7.3 Hz, 4H), 8.55 (s, 2H), 8.96 (d, J = 8.4 Hz, 2H), 9.15 (d, J = 8.4 Hz, 2H) ppm.
ACKNOWLEDGEMENTS
The authors would like to acknowledge the EPSRC, M-Base and Acinet-I3 for financial support. Use of the Chemical Analysis Facility (CAF) at the University of Reading is gratefully acknowledged.
References
1. F. W. Lewis, M. J. Hudson, and L. M. Harwood, Synlett, 2011, 2609. CrossRef
2. M. J. Hudson, L. M. Harwood, F. W. Lewis, and D. M. Laventine, Inorg. Chem., 2012, 51 (DOI: 10.1021/ic3008848). CrossRef
3. M. J. Hudson, C. E. Boucher, D. Braekers, J. F. Desreux, M. G. B. Drew, M. R. S. Foreman, L. M. Harwood, C. Hill, C. Madic, F. Marken, and T. G. A. Youngs, New J. Chem., 2006, 30, 1171. CrossRef
4. M. R. S. Foreman, M. J. Hudson, M. G. B. Drew, C. Hill, and C. Madic, Dalton Trans., 2006, 1645. CrossRef
5. F. W. Lewis, L. M. Harwood, M. J. Hudson, M. G. B. Drew, J. F. Desreux, G. Vidick, N. Bouslimani, G. Modolo, A. Wilden, M. Sypula, T. H. Vu, and J. P. Simonin, J. Am. Chem. Soc., 2011, 133, 13093. CrossRef
6. C. W. N. Cumper, R. F. A. Ginman, and A. I. Vogel, J. Chem. Soc., 1962, 1188. CrossRef
7. G. Donnay, J. D. H. Donnay, and M. J. C. Harding, Acta Cryst., 1965, 19, 688. CrossRef
8. P. Jones, G. B. Villeneuve, C. Fei, J. DeMarte, A. J. Haggarty, K. T. Nwe, D. A. Martin, A. M. Lebuis, J. M. Finkelstein, B. J. Gour-Salin, T. H. Chan, and B. R. Leyland-Jones, J. Med. Chem., 1998, 41, 3062. CrossRef
9. A. Zhang, E. Kuraoka, and M. J. Kumagai, Radioanalytical and Nuclear Chemistry, 2007, 274, 455. CrossRef
10. S. Usuda, R. Liu, Y. Wei, Y. Xu, H. Yamazaki, and Y. Wakui, Ion Exchange, 2009, 21, 35. CrossRef