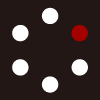
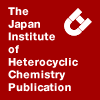
HETEROCYCLES
An International Journal for Reviews and Communications in Heterocyclic ChemistryWeb Edition ISSN: 1881-0942
Published online by The Japan Institute of Heterocyclic Chemistry
e-Journal
Full Text HTML
Received, 24th June, 2012, Accepted, 25th July, 2012, Published online, 6th August, 2012.
DOI: 10.3987/COM-12-S(N)54
■ ASSEMBLY OF THE SOUTHERN MACROCYCLIC HALF OF (+)-SPIRASTRELLOLIDE A THROUGH CYCLIC ACETAL TETHERED RING-CLOSING METATHESIS AND 1,3-ANTI-MUKAIYAMA-ALDOL
Yu Tang,* Jin-Haek Yang, Jia Liu, Chao-Chao Wang, Ming-Can Lv, Yi-Biao Wu, Xue-Liang Yu, Changhong Ko, and Richard Hsung*
Department of Chemistry, School of Pharmacy, University of Wisconsin, 777 Highland Aenue, Madison, WI 53705, U.S.A.
Abstract
We describe herein details of our efforts in syntheses of A-ring and BC-ring of (+)-spirastrellolide A. While the former would constitute a facile 12-step synthetic endeavor starting from 1,5-pentanediol, the latter would showcase a cyclic acetal-tethered ring-closing metathesis [RCM] method that was developed in our lab for de novo synthesis of spiroketals. Constructing the entire Southern Half of the macrocycle would require 1,3-anti-Mukaiyama aldol addition for connecting A-ring and BC-ring specifically at C10 and C11, thereby culminating a 17-step approach for the C1-23 fragment linearly from (+)-2,3-(O)-iso-propylidene-L-threitol. Also discussed here is the possibility of pursuing a more convergent approach toward the assembly of the Southern Half through first connecting A-ring and C-ring via acetal formation that would first link together the free C13-OH with C17 at the spiro-BC-ring junction. An ensuing application of our cyclic acetal-tethered RCM strategy to close B-ring would adopt this cyclic acetal intermediate.INTRODUCTION
Roberge and Andersen et al. in 2003 unveiled the rough structure of (+)-spirastrellolide A, a macrocyclic lactone that is rich in spiroketal motifs.1a Subsequently in 2004, they revised and completed the structural assignment of (+)-spirastrellolide A including all relative stereochemistry sans C46.1b In 2007, with the isolation of (+)-spirastrellolide B, the absolute configuration of the macrocyclic core was established through X-ray crystallography.1c In the same year, (+)-spirastrellolide C-G were also identified from the same marine sponge Spirastrella coccinea.1d (+)-Spirastrellolide A possess the ability to initiate premature entry into mitosis and untimely mitotic arrest in cells, and more importantly, it exhibits a potent inhibitory activity against protein phosphatase 2A [IC50 = 1 nM]. The level of inhibitory selectivity is also outstanding in favor of PP2A over PP1 by a factor of 50 in terms of IC50.2 In addition, (+)-spirastrellolide A does not inhibit PP2C! Its biological activities, therefore, resemble other known Ser/Thr phosphatase inhibitors fostriecin and okadaic acid.3
Development of protein phosphatase inhibitors has lagged behind the interest in kinase inhibitors because of the perceived notion that kinases are more highly regulated and specific.4 However, there has been a renewed interest in recent years because reversible protein phosphorylation is critical "as the other half" of checkpoints in cell cycles, and protein phosphatases assume an equally important role in regulating cellular signal transductions and should not be ignored. Designing phosphatase inhibitors can lead to new paradigms in developing cancer therapeutics.4 As a result, this family of natural products has attracted an elegant array of synthetic efforts5 with the very first total synthesis being reported by Paterson.6 We became interested in spirastrellolide A because we have been developing cyclic acetal tethered methods7a-c such as RCM7d-g,8 as an unconventional approach to constructing spiroketals.9 We report here our efforts toward the Southern Half of macrocyclic core of (+)-spirastrellolide A.
RESULTS AND DISCUSSION
1. Retrosynthetic Analysis.
Our synthetic analysis would first involve a disconnection at C40-41 that will be reconnected through Julia-Kocienski olefination, which was also documented in Smith5j [Scheme 1]. Consequently, this would leave behind the central macrocyclic core [labeled as G-ring] that can be further divided into three major fragments: 1 [C1 through C10], 2 [C11 through C23], and 3 [C24 through C40]. A macrolactonization could link C1 and C37 between fragments 1 and 3. A methyl ketone enolate anti-aldol will be used to connect fragments 1 and 2 at C10 and C11,5e,10 and another anti-aldol would link together fragments 2 and 3 at C23 and C24 but will require the addition of the C25-oxo group. Synthesis of fragment 2 could feature our cyclic acetal tethered RCM.7d-g
2. A-Ring Synthesis.
Our synthesis of A-ring10 would call for the commercially readily available 1,5-pentanediol as the starting point. As shown in Scheme 2, 1,5-pentanediol could be quickly transformed into aldehyde 4 in 47% overall yield via mono-benzylation and standard Swern oxidation. Standard HEW-modified Wittig-olefination followed by DIBAL-H reduction afforded exclusively E-allylic alcohol 5 in 95% overall yield. Sharpless asymmetric epoxidation employing D-(-)-diisopropyl tartrate provided epoxy alcohol 6 in 96% enantiomeric excess. It is noteworthy that we have provided here a completely different approach for establishing the C3 stereochemistry in A-ring of (+)-spirastrellolide A, as both Paterson5a-d,6 and De Brabander5g employed an asymmetric Brown-allylation.11a
A directed-reductive ring-opening of the epoxide proceeded regioselectively and a subsequent diol protection using 2,2-dimethoxy propane gave acetonide 7. With the optically enriched acetonide 7 in hand, we proceeded to complete the pyran synthesis. Debenzylation followed by Doering–Parikh oxidation11b gave aldehyde 8. Wittig olefination and hydrolytic removal of the acetonide group occurred concomitantly with the pyran formation yielded pyran 10 in 87% overall yield as a single diastereomer. The relative syn stereochemical relationship at C3 and C7 was confirmed through NOE. Although kinetic control has been noted,12 the high level of stereoselectivity is likely a result of thermodynamically controlled O-1,4-addition, leading to complete chirality transfer from C3 to C7. Subsequent Piv-protection of C1-OH in 10 gave methyl ketone 11, which would then complete our asymmetric synthesis of A-ring, and set up the critical C10 and C11 connection via an anti-aldol [Scheme 1].
3. BC-Ring Construction.
Synthesis of the Key Cyclic Acetal. Unlike the A-ring synthesis that featured asymmetric catalysis, to prepare BC-ring, we elected the Hanessian’s chiron-pool concept and commenced with commercially available (+)-2,3-(O)-iso-propylidene-L-threitol,10b,13a thereby borrowing the desired C21 and C22 stereochemistry. Mono-protection of the hydroxyl group in (+)-2,3-(O)-iso-propylidene-L-threitol with TBDPSCl followed by Doering–Parikh oxidation11b gave aldehyde 12 in 68% yield [Scheme 3]. A five-carbon chain extension of aldehyde 12 was accomplished with a five-step sequence: (i) Brown’s asymmetric allylation to set up the C20 stereochemistry in a reagent controlled manner;11a (ii) standard O-methylation to give methyl ether 13; (iii) classical 9-BBN hydroboration of 13; (iv) Doering–Parikh oxidation,11b or modified-Moffat protocol, to afford aldehyde 14; and (v) vinyl Grignard addition to yield allylic alcohol 15. Stereochemistry at C20 was confirmed using the classical Mosher ester analysis11c,d after the Brown’s asymmetric allylation step using 12.
At this juncture, we recognizing the potential of a more facile route to the key vinyl cyclic acetal, and thus, we proceeded to remove the acetonide group in allylic alcohol 15, and a subsequent MnO2 oxidation gave enone 16 that contains the free diol at C21 and C22 [Scheme 4]. However, attempts to access cyclic acetal 19 from 16 through oxocarbenium ion 17 via acid promoted condensation with 1,6-heptadiene-4-ol failed. From these attempts,14 we were only able to observe and/or isolate bicyclic acetal 18 in 81% yield when using Tf2NH.15-17 The formation of 18 is clearly a result of trapping of vinyl oxocarbenium ion 17 by the free C22-OH in a facile intramolecular manner. Given that 19 and 18 are both derived through 17 in a reversible manner, we tried but again failed at longer reaction time and higher temperatures to force 18 equilibrating toward 19.
After much experimentation, we ultimately suc ceeded in synthesizing the key vinyl cyclic acetal 25 via the route shown in Scheme 5. Lindgren oxidation18 of aldehyde 14 afforded acid 20. Removal of the acetonide group led to a selective lactone formation involving only C21-OH, and subsequent capping of C22-OH with PivCl gave lactone 21 in 64% overall yield. The ensuing addition of vinyl magnesium bromide followed by treatment of the resulting lactol mixture 22a/b with Tf2NH17 in the presence of alcohol 2419a,b afforded the desired vinyl cyclic acetal 25 in 56% yield, albeit still accompanied with the elimination product 26 that was not easily separable.
Exploring Conditions for the Cyclic Acetal Formation. Although we have developed our own unique protocols for the acetal formation using simple pyranyl systems7,8 and that there are ample reports for more robust carbohydrate systems,20 it is worthy to note that this particular cyclic acetal formation took some efforts to investigate. As summarized in Table 1, formation of cyclic acetal using lactol 27 [epi at C22], which contains the anomeric vinyl group at C17, proved to be challenging. A range of acids as well as solvents and temperatures were screened. While most frequently used Lewis acids and Brønsted acids in anomeric substitutions20 led to the over addition product 30, Tf2NH [entry 5] proved to be an excellent Brønsted acid at –78 oC, leading to 29 as the sole product in 89% yield as a single diastereomer with the oxo-butenyl group being axial.13b The ability of Tf2NH in leading to the desired outcome in an array of reaction pathways have been well noted,15,16 albeit poorly understood other than the fact that represents one softest anionic complex.17
Cyclic Acetal-Tethered RCM. At last, the key ring-closing metathesis [RCM] of vinyl cyclic acetal 25 led to BC-ring 32 in 95% yield using Grubbs' Generation-II Ru-catalyst [Scheme 6].21 It is noteworthy that NOE experiments of 32 revealed its the desired relative stereochemistry, which closely resemble those reported for the same region in spirastrellolide A.1 More importantly, the current synthesis of the C11-C23 fragment 32 took only 12 steps from (+)-2,3-(O)-iso-propylidene-L-threitol.13a This chiron approach is much shorter and synthetically more practical comparing to the previous 18-step route from D-glucose, which also led to epi-stereochemistry at C22.13b We should point out that our synthetic study represented the first in the area of spirastrellolide synthesis when we pursued the 18-step C22-epi-synthesis, and the correct assignment had not been reported by Roberge and Andersen et al. Our intent at the time was to simply showcase our strategy.
4. Attempted Convergent Approach to C1-C23.
To examine the concept of connecting A-ring and BC-ring at C10 and C11 through a diastereoselective aldol addition, and to explore a possibly more convergent route, we prepared aldehyde 33a19a,b and 33b.19c By employing Mukaiyama's conditions,22 methyl ketone 11 was first converted to its respective TMS-enol ether using LDA and TMSCl, and the resulting TMS-enol ether was added to aldehyde 33a [or 33b] followed by the addition of a stoichiometric amount of BF3-Et2O at –78 oC to give the aldol product 34 in 72% yield with a diastereomeric ratio of 9:1 [Scheme 7]. The major isomer was initially assigned as the desired C11,13-anti product based on Evans' non-chelation 1,3-asymmetric induction model.23 By using aldehyde 33b under the same conditions, the yield for the respective aldol product 35 was comparable but the dr was only 3:1. It is noteworthy that following Evans boron enolate conditions24 via di-n-butylboron enolate derived from 11 and aldehyde 33a in CH2Cl2 at –78 oC afforded 34 with 29% yield but in ~ 1:1 ratio.
A directed reduction of hydroxyl ketone 34 and 35 using Me4NBH(OAc)3 led to diols 36 and 37, respectively. The diastereoselectivity here is modest with the dr being 5:1 for both diols 36 and 37, the major isomer indeed favored C9 and C11 anti in relative stereochemistry. This anti-selectivity was unambiguously confirmed through NOE experiments using C9,11-anti acetonide 38 that could be prepared from C13-TBS-protected C9,11-anti-diol 36. To be ascertain, C9,11-syn acetonide 38’ was also prepared in an analogous sequence to obtain a contrasting NOE outcome [Scheme 7].25 On the other hand, by using C13-PMB-protected C9,11-anti-diol 37, we were able to finally unambiguously assign the C11,13-anti relative stereochemistry through NOE of the corresponding PMP acetal 40, which could be accessed via DDQ oxidation of 37.
While syntheses of C9,11-anti acetonide 38 was quintessential in confirming key stereochemical assignments, we were also attempting to explore a more convergent route to the Southern Half by first connecting the A-ring and C-ring through cyclic acetal formation, linking first together C13-OH with C17. However, that possibility did not work well. As shown in Scheme 8, attempted synthesis of vinyl cyclic acetal 41 using alcohol 39 [via desilylation of 38] and lactol 22b was not successful under standard conditions as well as other conditions. Thus, this thwarted our efforts to assembly the entire ABC tricycle 42 through closing of the B-ring at the very end via the cyclic acetal tethered RCM strategy.
5. Completing the Southern Macrocyclic Half.
To complete the synthesis of the Southern Half of the macrocycle G-ring, BC-ring 32 was transformed to aldehyde 43 via removal of the PMB protecting group and SO3-Pyr/DMSO oxidation [Scheme 9]. To connect the C10-C11 bond, aldehyde 43 was subjected to the same Mukaiyama aldol conditions21 employing TMS-enol ether derived in situ from methyl ketone 11. It was once again important here that TMS-enol ether was first added to aldehyde 43 prior to the addition of a stoichiometric amount of BF3-Et2O at –78 oC. The aldol product 44 was obtained as a single isomer in 62% yield with C11-C13 relative stereochemistry assigned as anti based on the study described above in the preparation of 40.
This assignment is again also consistent with Evans non-chelation 1,3-asymmetric induction dipole directed model.22 Directed reduction led to diols 45 and 46 with a 3:1 ratio in favor of the C9,11-anti isomer. Although the selectivity was low, both isomers would sever a real purpose. Acetonide formation of both anti and syn diol isomers 45 and 46 gave the desired C9,11-anti acetonide 42, and C9,11-syn acetonide 47, respectively. With both syn and anti isomers in hand, we could unambiguously assign the C9 and C11 relative stereochemistry in both 42 and 47 through the Rychnovsky-Evans C-13 analysis,26,27 thereby completing our endeavor in the assembly of the entire Southern macrocyclic Half of (+)-spirastrellolide A.
CONCLUSION
We have described here details of our efforts toward the construction of both A-ring and BC-ring of (+)-spirastrellolide A. The A-ring synthesis constituted a facile 12-step linear sequence starting from 1,5-pentanediol, and the BC-ring synthesis would ultimately showcase a cyclic acetal-tethered RCM strategy that was developed in our lab for de novo synthesis of spiroketals. Assembly of the entire Southern Half of this unique macrocycle would also require 1,3-anti-Mukaiyama aldol addition for connecting A-ring and BC-ring specifically between C10 and C11, thereby culminating a successful and practical 17-step approach linearly from (+)-2,3-(O)-iso-propylidene-L-threitol. An attempt toward an even more convergent synthesis of the Southern Half was also carried out. However, our efforts failed in connecting A-ring and C-ring through an acetal formation that would have linked together the free C13-OH with C17 at the spiro-BC-ring junction. This cyclic acetal intermediate was to be subjected to our cyclic acetal-tethered RCM strategy to close the B-ring. Nevertheless, the current 17-step approach should prove to be highly practical for an ultimate total synthesis of (+)-spirastrellolide A.
EXPERIMENTAL
Synthesis of Allyl Alcohol 5.
To a slurry of NaH (9.22 g, 230 mmol) in anhyd THF was added dropwise 1,5-pentanediol at 0 °C. After stirring this slurry for 30 min, benzyl bromide (25.6 mL, 211.2 mmol) was added. The reaction mixture was refluxed for 12 h before being quenched with dropwise addition of H2O. The organic phase was extracted with EtOAc, washed with sat aq NaCl, and dried over Na2SO4. The solvent was concentrated under reduced pressure and the crude residue was purified by flash silica gel column chromatography [33% EtOAc in hexane] to provide mono-benzylated diol as colorless oil (21.0 g) in 56% yield. Rf = 0.30 [33% EtOAc in hexane]; 1H NMR (500MHz, CDCl3) δ 1.41-1.48 (m, 2H), 1.58 (tt, J = 6.5, 6.5 Hz, 2H), 1.64 (tt, J = 7.0, 7.0 Hz, 2H), 2.02 (brs, 1H), 3.48 (t, J = 6.5 Hz, 2H), 3.62 (t, J = 7.0 Hz, 2H), 4.51 (s, 2H), 7.26-7.36 (m, 5H); 13C NMR (125MHz, CDCl3) δ 22.7, 29.7, 32.8, 63.1, 70.6, 73.2, 127.8, 127.9, 128.7, 138.8; IR (neat) cm-1 3393br, 3031s, 2936s, 2861s, 2360s, 2342s, 1455s, 1363s, 1098m; mass spectrum (APCI): m/z (% relative intensity) 195 (M+H) + (75), 191 (8), 184 (9), 168 (10), 145 (19), 139 (15), 117 (30), 101 (100), 100 (51).
To a solution of oxalyl chloride (10.5 mL, 118 mmol) in CH2Cl2 was added a solution of DMSO (17 mL, 238.0 mmol) in CH2Cl2 at – 78 °C. After the addition, the mixture was stirred for 30 min. before the above mono-benzylated diol (21.0 g, 108.0 mmol) was added to dropwise over 20 min period. The mixture was stirred for an additional 1 h at –78 °C before Et3N (80.0 mL, 570.0 mmol) was added through a syringe, and the mixture was slowly warmed up to rt. Subsequently, the reaction mixture was washed with H2O and sat aq NH4Cl. The organic phase was extracted with EtOAc, dried over Na2SO4, and concentrated under reduced pressure. The crude residue was purified by flash silica gel column chromatography [20% EtOAc in hexane] to aldehyde 4 as yellow oil (18.0 g) in 87% yield. Rf = 0.33 [20% EtOAc in hexane]; 1H NMR (400MHz, CDCl3) δ 1.59-1.67 (m, 2H), 1.68-1.76 (m, 2H), 2.42 (td, J = 2.0, 7.2 Hz, 2H), 3.47 (t, J = 6.4 Hz, 2H), 4.51 (s, 2H), 7.24-7.35 (m, 5H), 9.72 (t, J = 2.0 Hz, 1H); 13C NMR (125MHz, CDCl3) δ 19.2, 29.4, 43.8, 70.0, 73.2, 127.8, 127.9, 128.6, 138.7, 202.7; IR (neat) cm-1 2936s, 2860s, 1720s; mass spectrum (APCI): m/z (% relative intensity) 215 (M+Na)+ (11), 171 (8), 145 (41), 121 (20), 115 (55), 101 (100).
To a solution of aldehyde 4 (18.0 g, 94.0 mmol) in CH2Cl2 was added Wittig reagent Ph3P=CHCO2Et (50.0 g, 141.0 mmol). After stirring at rt for 5 h, excess of CH2Cl2 was evaporated under reduced pressure and the residue was diluted with a small amount of hexane. The slurry was filtered through a pad of CeliteTM to remove triphenylphosphine oxide. The filtrate was concentrated under reduced pressure. The crude residue was purified by flash silica gel column chromatography [10% EtOAc in hexane] to provide Wittig olefination product as colorless oil (24.0 g) in 98% yield and ≥25:1 E:Z selectivity. Rf = 0.30 [10% EtOAc in hexane]; 1H NMR (400MHz, CDCl3) δ 1.27 (t, J = 7.0 Hz, 3H), 1.51-1.59 (m, 2H), 1.60-1.67 (m, 2H), 2.20 (ddt, J = 2.0, 7.2, 14.4 Hz, 2H), 3.46 (t, J = 6.0 Hz, 2H), 4.17 (q, J = 7.0 Hz, 2H), 4.50 (s, 2H), 5.81 (dt, J = 1.6, 15.6 Hz, 1H), 6.95 (dt, J = 6.8, 15.6 Hz, 1H), 7.24-7.36 (m, 5H); 13C NMR (125 MHz, CDCl3) δ 14.6, 25.0, 29.5, 32.2, 60.4, 70.2, 73.2, 121.8, 127.8, 127.9, 128.7, 138.8, 149.2, 167.0; IR (neat) cm-1 2980m, 2937s, 2859s, 2360br, 1719s, 1654s; mass spectrum (APCI): m/z (% relative intensity) 263 (M+H) + (7), 218(15), 217(100), 200(7), 199(52), 175(7), 171(10), 157(10).
To a solution of the above Wittig olefination product (24.0 g, 92.0 mmol) in THF was added DIBAL-H (1.0 M in toluene, 239.0 mL, 230.0 mmol) at –78 °C. The mixture was gradually warmed up to –50 °C and stirred for an additional 1.5 h before being quenched with aq HCl (1.0 M, 150 mL). The reaction mixture was extracted with EtOAc, and combined organic layers was dried over Na2SO4 and concentrated under reduced pressure. The crude residue was purified with flash silica gel column chromatography [33% EtOAc in hexane] to provide allyl alcohol 5 (20.0 g) in 98 % yield. Rf = 0.35 [33% EtOAc in hexane]; 1H NMR (400 MHz, CDCl3) δ 1.44-1.51 (m, 2H), 1.60-1.67 (m, 2H), 1.80 (s, 1H), 2.04-2.09 (q, J = 7.2 Hz, 2H), 3.47 (t, J = 6.4 Hz, 2H), 4.08 (d, J = 4.0 Hz, 2H), 4.50 (s, 2H), 5.60-5.73 (m, 2H), 7.25-7.37 (m, 5H); 13C NMR (125 MHz, CDCl3) δ 26.0, 29.5, 32.2, 63.8, 70.4, 73.1, 127.8, 127.9, 128.6, 129.6, 132.9, 138.8; IR (neat) cm-1 3393brs, 2934s, 2858s, 2361s, 1455s, 1363s; mass spectrum (ESI): m/z (% relative intensity) 243 (M+Na)+ (100).
Sharpless Asymmetric Epoxidation of Allyl Alcohol 5.
To a suspension of dried and pulverized Molecular Sieve 4Å in anhyd CH2Cl2 (6 mL) was added D-(-)-di-isopropyl tartrate (0.051 mL, 0.24 mmol) followed by slow addition of Ti(Oi-Pr)4 (0.048 mL, 0.16 mmol) at –20 °C. After which the above allyl alcohol 5 (176.6 mg, 0.802 mmol) was added and the mixture was stirred for 5 min. before TBHP (3.4 M, 0.71 mL, 2.40 mmol) was added slowly and the resulting solution was stirred at – 20 °C for 1.5 h. The reaction was then quenched with aq NaOH (1.0 N) and was stirred for an additional 1 h at 0 °C before it was warmed up to rt. The reaction mixture was filtered through a pad of CeliteTM. The organic phase was extracted with CH2Cl2, dried over Na2SO4 and concentrated under reduced pressure. The crude residue was purified by flash silica gel column chromatography [50% EtOAc in hexane] to provide the desired epoxy alcohol 6 (164.4 mg) in 87% yield and 96% ee. The enantiomeric excess was determined using chiral HPLC. The retention times of the two enantiomers were 25.789 (S) min and 27.861 (R) min respectively. Rf = 0.10 [30% EtOAc in hexane]; [α]D23 = 36.6 [c = 0.89, CH2Cl2]; 1H NMR (500 MHz, CDCl3) δ 1.42-1.60 (m, 4H), 1.60-1.69 (m, 2H), 2.20 (brt, J = 5.0 Hz, 1H), 2.91 (dt, J = 2.5, 4.0 Hz, 1H), 2.95 (dt, J = 2.5, 5.5 Hz, 1H), 3.49 (t, J = 6.5 Hz, 2H), 3.62 (ddd, J = 4.5, 7.0, 11.5 Hz, 1H), 3.89 (ddd, J = 2.5, 5.5, 13.0 Hz, 1H), 4.51 (s, 2H), 7.28-7.30 (m, 1H), 7.30-7.35 (m, 4H); 13C NMR (125 MHz, CDCl3) δ 22.7, 29.5, 31.4, 56.0, 58.7, 61.9, 70.1, 72.9, 127.6, 127.7, 128.4, 138.5; IR (neat) cm-1 3434brs, 3030m, 2936s, 2861m, 1100s; mass spectrum (APCI): m/z (% relative intensity) 237.2 (M+H)+ (100); m/z calcd for C14H20O3Na+ 259.1310, found 259.1305.
Preparation of Acetonide 7.
To a solution of epoxy alcohol 6 (1.80 g, 7.50 mmol) in THF (10 mL) was added Red-Al (8.0 mL, 26.3 mmol) dropwise at ¬–10 °C. The mixture was warmed up to rt and stirred for an additional 3 h. Subsequently, sat aq NH4Cl (20 mL) was added and the resulting mixture was stirred for another 1 h. The reaction mixture was extracted with Et2O, dried over Na2SO4, and concentrated under reduced pressure. The crude residue was purified by flash silica gel column chromatography [91% EtOAc in hexane] to the desired diol (1.76 g) in 98% yield. Rf = 0.33 [91% EtOAc in hexane]; 1H NMR (400MHz, CDCl3) δ 1.39-1.57 (m, 4H), 1.59-2.04 (m, 4H), 2.81 (brs, 1H), 2.91 (brs, 1H), 3.50 (t, J = 6.4 Hz, 2H), 3.78-3.85 (m, 3H), 4.50 (s, 2H), 7.25-7.37 (m, 5H); 13C NMR (125MHz, CDCl3) δ 22.5, 29.9, 37.8, 38.5, 62.1, 70.6, 72.3, 73.2, 127.8, 128.0, 128.7, 138.8; IR (neat) cm-1 3366brs, 2933s, 2858s, 2360s, 2341s; mass spectrum (ACPI): m/z (% relative intensity) 239 (M+H)+ (7), 221(17), 203(24), 185(12), 143(13), 131(16), 129(32), 117(7), 113(100), 111(9).
To a solution of the above diol (161.0 mg, 0.68 mmol) in acetone was added molecular sieve 4Å and dimethoxy-propane (0.25 mL, 2.03 mmol) followed by p-TsOH·H2O (12.8 mg, 0.068 mmol). The mixture was stirred for 12 h before it was quenched with sat aq NaHCO3 (10 mL). The reaction mixture was extracted with EtOAc, dried over Na2SO4, and concentrated under reduced pressure. The crude residue was purified by flash silica gel column chromatography [17% EtOAc in hexane] to provide acetonide 7 (170.9 mg) in 91% yield. Rf = 0.35 [17% EtOAc in hexane]; 1H NMR (400 MHz, CDCl3) δ 1.38 (s, 3H), 1.38-1.43 (m, 2H), 1.44 (s, 3H), 1.46-1.58 (m, 4H), 1.63 (tt, J = 6.8, 6.8 Hz, 2H), 3.47 (t, J = 6.8 Hz, 2H), 3.82 (ddd, J = 1.6, 5.2, 12.0 Hz, 2H), 3.95 (td, J = 2.8, 12.4 Hz, 1H), 4.54 (s, 2H), 7.26-7.37 (m, 5H); 13C NMR (125 MHz, CDCl3) δ 19.5, 21.8, 29.9, 30.3, 31.5, 36.5, 60.3, 69.0, 70.5, 73.1, 98.4, 127.7, 127.9, 128.6, 138.9; IR (neat) cm-1 2992s, 2940s, 2862s, 2360s; mass spectrum (ESI): m/z (% relative intensity) 301.7 (M+Na)+ (100); m/z calcd for C17H26O3Na+ 301.1780, found 301.1774.
Synthesis of Aldehyde 8
A suspension of acetonide 7 (587.5 mg, 2.10 mmol) and 10% Pd/C (45.0 mg) in EtOAc was stirred under a balloon of hydrogen at rt for 1 h. The reaction mixture was filtered through a pad of CeliteTM and the filtrate was concentrated under reduced pressure. The crude oil was used for the next step without further purification. Rf = 0.50 [83% EtOAc in hexane]; 1H NMR (400MHz, CDCl3) δ 1.33 (s, 3H), 1.33-1.40 (m, 2H), 1.40 (s, 3H), 1.42-1.58 (m, 6H), 2.22 (brs, 1H), 3.58 (t, J = 6.4 Hz, 2H), 3.80-3.88 (m, 2H), 3.93-3.99 (td, J = 2.8, 12.0 Hz, 1H); 13C NMR (125MHz, CDCl3) δ 19.4, 21.4, 30.2, 31.5, 32.7, 36.3, 60.2, 62.7, 69.1, 98.4.
To a solution of the above crude alcohol in CH2Cl2/DMSO (2.5 mL, 10.6 mmol) were added Et3N (1.60 mL, 11.3 mmol) and SO3·pyridine complex (1.4 g, 8.5 mmol) at 0 °C. The resulting mixture was stirred at 0 °C for 1.5 h before it was quenched with H2O and extracted with CH2Cl2. The combined organic layers were washed with sat aq NaCl, dried over Na2SO4, and concentrated under reduced pressure. The crude residue was purified with flash silica gel column chromatography [33% EtOAc in hexane] to provide aldehyde 8 (203.4 mg) in 52% yield. Rf = 0.45 [33% EtOAc in hexane]; 1H NMR (400 MHz, CDCl3) δ 1.38 (s, 3H), 1.39-1.44 (m, 1H), 1.45 (s, 3H), 1.47-1.83 (m, 5H), 2.46 (td, J = 1.6, 6.4 Hz, 2H), 3.81-3.88 (m, 2H), 3.93-3.99 (td, J = 2.8, 12.0 Hz, 1H), 9.77 (t, J = 1.6 Hz, 1H); 13C NMR (125 MHz, CDCl3) δ 18.0, 19.5, 30.2, 31.5, 36.0, 44.0, 60.2, 68.8, 98.5, 202.8; IR (neat) cm-1 3300m, 2993s, 2938s, 2869s, 1718s.
Preparation of A-Ring 11.
To a solution of aldehyde 8 (23.0 mg, 0.12 mmol) in THF (1 mL) was added Wittig reagent Ph3P=CHCOMe (99.3 mg, 0.31 mmol) at rt and the mixture was refluxed for 5 h. The solution was then cooled down to rt and concentrated under reduced pressure. The crude residue was purified with flash column chromatography [33% EtOAc in hexane] to provide the desired E-enone (26.4 mg, 0.12 mmol) in 95% yield. Rf = 0.37 [33% EtOAc in hexane]; 1H NMR (400MHz, CDCl3) δ 1.38 (s, 3H), 1.39-1.43 (m, 2H), 1.45 (s, 3H), 1.46-1.79 (m, 6H), 2.25 (s, 3H), 3.81-3.88 (m, 2H), 3.96 (dt, J = 2.8, 12.0 Hz, 1H), 6.08 (dt, J = 1.6, 16.0 Hz, 1H), 6.80 (dt, J = 7.2, 16.0 Hz, 1H); 13C NMR (125MHz, CDCl3) δ 19.5, 23.8, 27.1, 30.3, 31.5, 32.6, 36.2, 60.2, 68.8, 98.5, 131.8, 148.3, 198.9; IR (neat) cm-1 3750m, 3675m, 3649m, 3629m, 2992s, 2940s, 2865s, 2340s, 1698s, 1673s, 1626s.
To a solution of the above E-enone (81.2 mg, 0.36 mmol) in CH2Cl2 was added p-TsOH·H2O (20.8 mg, 0.11 mmol) at 0 °C. The mixture was stirred for 1 h before it was quenched with sat aq NaHCO3. The reaction mixture was extracted with CH2Cl2, and the combined organic layers were dried over Na2SO4 and concentrated under reduced pressure. The crude residue was purified by flash silica gel column chromatography [67% EtOAc in hexane] to provide pyran 10 (60.3 mg) as a colorless oil in 91% yield. Rf = 0.33 [67% EtOAc in hexane]; 1H NMR (400 MHz, CDCl3) δ 1.17-1.35 (m, 2H), 1.51-1.65 (m, 4H), 1.66-1.76 (m, 1H), 1.81-1.87 (m, 2H), 2.16 (s, 3H), 2.47 (dd, J = 4.8, 16.0 Hz, 1H), 2.57 (brs, 1H), 2.67 (dd, J = 7.6, 16.0 Hz, 1H), 3.60 (dddd, J = 2.0, 3.6, 8.4, 12.8 Hz, 1H), 3.76 (dt, J = 5.6, 5.6 Hz, 2H), 3.83 (dddd, J = 2.0, 4.8, 8.0, 12.8 Hz, 1H); 13C NMR (125 MHz, CDCl3) δ 23.5, 31.1, 31.3, 31.4, 38.2, 50.3, 61.5, 74.2, 78.5, 207.5; IR (neat) cm-1 3852m, 3675m, 3649m, 3629m, 3376brs, 2934s, 2860s, 2340s, 1716s, 1670s; mass spectrum (APCI): m/z (% relative intensity) 187 (M+H) + (100), 169 (24), 151(27), 129(84), 111(18); mass spectrum (ESI): m/z calcd for C10H18O3Na+ 209.1154, found 209.1148.
To a solution of pyran 10 (60.3 mg, 0.32 mmol) in CH2Cl2 was added pyridine (0.11 mL, 1.30 mmol). After stirring at rt for 0.5 h and pivaloyl chloride (0.08 ml, 0.65 mmol) was added. The mixture was stirred for an additional 1 h before it was quenched with H2O. The organic phase was extracted with CH2Cl2, dried over Na2SO4, and concentrated under reduced pressure. The crude residue was purified with flash silica gel column chromatography [25% EtOAc in hexane] to provide A-ring 11 or the C1-C11 fragment (76.2 mg) as yellowish oil in 87% yield. Rf = 0.40 [25% EtOAc in hexane]; 1H NMR (400 MHz, CDCl3) δ 1.19 (s, 9H), 1.21 (m, 2H), 1.53-1.63 (m, 3H), 1.75 (dd, J = 7.0, 13.0 Hz, 2H), 1.82-1.85 (m, 1H), 2.18 (s, 3H), 2.41 (dd, J = 5.0, 15.0 Hz, 1H), 2.66 (dd, J = 7.5, 15.0 Hz, 1H), 3.42 (dddd, J = 1.5, 7.0, 10.5, 13.0 Hz, 1H), 3.75 (dddd, J = 2.0, 5.0, 8.0, 11.0 Hz, 1H), 4.12 (ddd, J = 6.0, 10.5, 11.0 Hz, 2H); 13C NMR (125 MHz, CDCl3) δ 23.7, 27.4, 31.2, 31.5, 31.6, 35.6, 39.0, 50.6, 61.3, 74.7, 178.7, 207.8 [one carbon missing due to overlap]; IR (neat) cm-1 3420brs, 2929s, 2857s, 2360s, 2341s 1710s; mass spectrum (APCI): m/z (% relative intensity) 271 (M+H)+ (100), 253 (71), 213 (85), 169 (50), 151 (89), 133(19), 129 (6), 111 (42); m/z calcd for C15H26O4Na+ 293.1729, found 293.1725.
Synthesis Homo-Allyl Methyl Ether 13 from (+)-2,3-(O)-Iso-Propylidene-L-Threitol.
TBDPS-Silyl Protection. To a solution of commercially available (+)-2,3-(O)-iso-propylidene-L-threitol (1.03 g, 6.30 mmol) in THF (30 mL) was added NaH (252.0 mg, 6.3 mmol) at –10 oC. The mixture was gradually warmed up to rt and stirred for 1 h before being cooled back down to –10 oC and TBDPSCl (1.77 mL, 6.90 mmol) was added. After 2 h at rt, the reaction was quenched with H2O (20 mL). The organic solvent was evaporated and the aqueous fraction was extracted with CH2Cl2 (3 × 20 mL). The organic phases were combined, washed with sat aq NaCl, dried over Na2SO4, filtered, and concentrated under reduced pressure. The crude residue was purified by silica gel flash column chromatography [gradient eluent: 8-25% EtOAc in hexane] to provide the desired TBDPS-silyl ether in 83% yield (2.09 g) as yellow oil. Rf = 0.60 [30% EtOAc in hexane]; 1H NMR (300 MHz, CDCl3) δ 1.09 (s, 9H), 1.42 (s, 3H), 1.45 (s, 3H), 2.00 (brds, 1H), 3.68 (dd, J = 11.7, 4.4 Hz, 1H), 3.76 (dd, J = 10.6, 6.2 Hz, 1H), 3.84 (dt, J = 9.7, 4.9 Hz, 2H), 4.03 – 3.95 (m, 1H), 4.12 (ddd, J = 12.1, 11.3, 5.7 Hz, 1H), 7.53 – 7.32 (m, 6H), 7.69 (t, J = 6.1 Hz, 4H); mass spectrum (ESI): m/z (% relative intensity) 423.2 (M+Na)+ (100), 401.1 (M+H)+ (100); m/z calcd for C23H32O4SiNa+ 423.1968, found 423.1966.
SO3-Pyridine Oxidation. To a solution of the above silyl ether (2.09 g, 5.20 mmol), anhyd DMSO (7.38 mL, 104.0 mmol) and anhyd Et3N (3.62 mL, 25.9 mmol) in CH2Cl2 (21 mL) was added SO3-pyridine (3.31 g, 20.8 mmol) at –10 oC. The solution was stirred at –10 oC for 2 h and was quenched with H2O at -10 oC. The organic phase was separated and the aqueous fraction was extracted with CH2Cl2 (3 × 20 mL). The combined organic phases were dried over Na2SO4, filtered, and concentrated under reduced pressure. The residue was purified by silica gel flash column chromatography [gradient eluent: 10-30% EtOAc in hexane] to provide aldehyde 12 as colorless oil in 82% yield (1.69 g). 12: Rf = 0.35 [50% EtOAc in hexane]; 1H NMR (300 MHz, CDCl3) δ 1.09 (s, 9H), 1.45 (s, 3H), 1.52 (s, 3H), 3.84 (dd, J = 4.2, 11.1 Hz, 4H), 3.90 (dd, J = 4.5, 11.1 Hz, 1H), 4.22 (dt, J = 4.2, 6.9 Hz, 1H), 4.48 (dd, J = 1.8, 7.2 Hz, 1H), 7.41-7.48 (m, 6H), 7.69-7.74 (m, 4H), 9.83 (d, J = 1.5 Hz, 1H).
Asymmetric Allylation of Aldehyde 12. To a solution of (-)-(Ipc)2BOMe (1.80 g, 5.69 mmol) in Et2O (15 mL) was added allylmagnesium bromide (1.0 M in Et2O, 4.93 mL, 4.93 mmol) at 0 oC. The solution was warmed up to rt and stirred for an additional 1 h to give a white suspension. The suspension was cooled to 0 oC and allowed to settle for 0.5 h. The upper supernatant was transferred to a solution of aldehyde 12 (1.51 g, 3.79 mmol) in ether (10 mL) via cannula at –78 oC and the mixture was stirred at –78 oC for 3 h before it was quenched with aq NaOH (3.0 M, 20 mL) and 30% H2O2 (8 mL) at –78 oC. The mixture was reflux overnight. The organic phase was separated and the aqueous fraction was extracted with Et2O (3 × 20 mL). The combined organic phases were dried over Na2SO4, filtered, and concentrated under reduced pressure. The residue was purified by silica gel flash column chromatography [gradient eluent: 10-20% EtOAc in hexane] followed by removing isopinocampheol (Ipc-OH) byproduct through Kugelrohr distillation at 50 oC [1.0 mmHg] to provide the pure homoallyl alcohol as colorless oil in 72% yield (1.20 g). Rf = 0.60 [25% EtOAc in hexane]; [α]D23 = - 3.83 [c 0.31, CHCl3]; 1H NMR (500 MHz, CDCl3) δ 1.09 (s, 9 H), 1.41 (s, 3H), 1.42 (s, 3H), 2.23 (ddd, J = 7.5, 7.5, 14.5 Hz, 1H), 2.41 (m, 1H), 2.52 (d, J = 3.0 Hz, 1H), 3.78 (m, 1H), 3.80 (d, J = 4.5 Hz, 2H), 3.82 (dd, J = 7.0, 7.0 Hz, 1H), 4.08 (ddd, J = 4.5, 4.5, 7.0 Hz, 1H), 5.15 (d, J = 10.5 Hz, 1H), 5.16 (d, J = 17.0 Hz, 1H), 5.92 (dddd, J = 7.0, 7.0, 10.5, 17.0 Hz, 1H), 7.41-7.44 (m, 6H), 7.70-7.72 (m, 4H); 13C NMR (125 MHz, CDCl3) δ 19.2, 26.8, 27.0, 27.1, 37.7, 64.7, 71.4, 79.1, 80.5, 109.0, 118.0, 127.8, 129.9, 132.9, 134.4, 135.7; IR (film) cm-1 3470 brs, 3072m, 2933s, 2859m, 1112s; mass spectrum (ESI): m/z (% relative intensity) 463.2 (M+Na)+ (100); m/z calcd for C26H36O4SiNa+ 463.2281, found 463.2275.
Methyl Ether Formation. To a solution of the above homoallylic alcohol (1.20 g, 2.72 mmol) in THF (14 mL) was added NaH (163.2 mg, 4.08 mmol) at –10 oC. The solution was warmed up to rt and stirred for an additional 1 h before MeI (0.34 mL, 5.44 mmol) was added. The mixture was stirred at rt for 12 h and quenched with H2O (15 mL). The organic phase was evaporated and the aqueous fraction was extracted with CH2Cl2 (3 × 20 mL). The combined organic phases were dried over Na2SO4, filtered, and concentrated under reduced pressure. The residue was purified by silica gel flash column chromatography [gradient eluent: 2-3% EtOAc in hexane] to provide methyl ether 13 as colorless oil in 59% yield (725.0 mg). 13: Rf = 0.70 [16% EtOAc in hexane]; [α]D23 = - 7.54 [c 0.93, CHCl3]; 1H NMR (500 MHz, CDCl3) δ 1.10 (s, 9 H), 1.45 (s, 3H), 1.46 (s, 3H), 2.33-2.45 (m, 2H), 3.39 (s, 3H), 3.40 (m, 1H), 3.79 (dd, J = 4.0, 11.0 Hz, 1H), 3.90 (dd, J = 3.5, 11.0 Hz, 1H), 4.08 (dt, J = 4.0, 7.0 Hz, 1H), 4.12 (dd, J = 5.0, 8.0 Hz, 1H), 5.11 (d, J = 10.0 Hz, 1H), 5.15 (dd, J = 1.5, 17.0 Hz, 1H), 5.91 (ddt, J = 7.0, 10.0, 17.0 Hz, 1H), 7.40-7.45 (m, 6H), 7.73-7.76 (m, 4H); 13C NMR (125 MHz, CDCl3) δ 19.3, 26.9, 27.2, 27.3, 34.7, 58.1, 64.8, 77.9, 79.6, 81.5, 109.2, 117.2, 127.7, 129.7, 133.3, 134.6, 135.5; IR (film) cm-1 3072m, 2933s, 2861m, 1108s; mass spectrum (ESI): m/z (% relative intensity) 477.2 (M+Na)+ (100); m/z calcd for C27H38O4SiNa+ 477.2432, found 477.2418.
Synthesis of Methoxy Aldehyde 14.
Hydroboration of Methyl Ether 13. To a solution of methyl ether 13 (91.6 g, 0.20 mmol) in THF (2 mL) was added 9-BBN (0.5 M, 0.81 mL, 0.4 mmol) at 0 oC. The solution was warmed up to rt and stirred for 5 h before aq NaOH (3.0 M, 2 mL) and 30% H2O2 (1 mL) were added. The mixture was refluxed for 2 h. The organic phase was evaporated and the aqueous fraction was extracted with CH2Cl2 (3 × 10 mL). The combined organic phases were dried over Na2SO4, filtered, and concentrated under reduced pressure. The residue was purified by silica gel flash column chromatography [gradient eluent: 30-40% EtOAc in hexane] to provide the desired alcohol as colorless oil in 71% yield (66.9 mg). Rf = 0.30 [30% EtOAc in hexane]; [α]D23 = -18.0 [c 0.75, CHCl3]; 1H NMR (500 MHz, CDCl3) δ 1.07 (s, 9H), 1.42 (s, 3H), 1.43 (s, 3H), 1.60-1.75 (m, 4H), 1.89 (brs, 1H), 3.35 (m, 1H), 3.39 (s, 3H), 3.64 (m, 2H), 3.76 (dd, J = 4.5, 10.5 Hz, 1H), 3.87 (dd, J = 3.7, 11.0 Hz, 1H), 4.01 (ddd, J = 4.0, 4.0, 8.0 Hz, 1H), 4.12 (dd, J = 5.0, 7.5 Hz, 1H), 7.38-7.41 (m, 6H), 7.69-7.72 (m, 4H); 13C NMR (125 MHz, CDCl3) δ 19.3, 26.6, 26.8, 27.1, 27.2, 28.5, 58.1, 62.9, 64.7, 78.0, 79.4, 81.6, 109.1, 127.7, 129.7, 133.3, 135.7; IR (film) cm-1 3425brs, 3072m, 2936s, 2864m, 1109s; mass spectrum (ESI): m/z (% relative intensity) 495.3 (M+Na)+ (100); m/z calcd for C27H40O5SiNa+ 495.2537, found 495.2541.
SO3·Pyridine Oxidation. To a solution of the above alcohol (66.9 mg, 0.14 mmol), anhyd DMSO (0.20 mL, 2.82 mmol), and anhyd Et3N (0.11 mL, 0.79 mmol) in CH2Cl2 (2 mL) was added SO3·pyridine (89.8 mg, 0.56 mmol) at –10 oC. The solution was stirred at –10 oC for 2 h and was quenched with H2O at –10 oC. The organic phase was separated and the aqueous fraction was extracted with CH2Cl2 (3 × 5 mL). The combined organic phases were dried over Na2SO4, filtered, and concentrated under reduced pressure. The residue was purified by silica gel flash column chromatography [gradient eluent: 5-15% EtOAc in hexane] to provide aldehyde 14 as colorless oil in 99% yield (65.5 mg). 14: Rf = 0.70 [30% EtOAc in hexane]; [α]D23 = - 23.5 [c 5.56, CHCl3]; 1H NMR (500 MHz, CDCl3) δ 1.08 (s, 9H), 1.42 (s, 6H), 1.92 (dt, J = 7.0, 7.5 Hz, 2H), 2.54 (dt, J = 1.5, 7.0 Hz, 2H), 3.32 (s, 3H), 3.33 (m, 1H), 3.77 (dd, J = 4.5, 11.5 Hz, 1H), 3.87 (dd, J = 3.5, 11.0 Hz, 1H), 4.00 (ddd, J = 4.0, 4.0, 7.0 Hz, 1H), 4.10 (dd, J = 5.0, 12.0 Hz, 1H), 7.39-7.42 (m, 6H), 7.69-7.72 (m, 4H), 9.75 (s, 1H); 13C NMR (125 MHz, CDCl3) δ 19.3, 22.8, 26.8, 27.1, 27.2, 39.6, 58.0, 64.6, 77.7, 79.5, 80.8, 109.3, 127.7, 129.7, 133.2, 135.7, 202.1; IR (film) cm-1 3071m, 2935s, 2861m, 1726s, 1108s; mass spectrum (ESI): m/z (% relative intensity) 493.2 (M+Na)+ (100); m/z calcd for C27H38O5SiNa+ 493.2386, found 493.2372.
Synthesis of Diol Enone 16 and Isolation of Bicyclic Acetal 18.
Vinyl Grignard Addition to Aldehyde 14. To a solution of aldehyde 14 (534.3 mg, 1.14 mmol) in Et2O (10 mL) was added vinyl magnesium bromide (1.0 M in Et2O, 2.28 mL, 2.28 mmol) dropwise at –78 oC. The solution was stirred for 3 h at –78 oC and quenched with sat aq NaHCO3 (10 mL). The organic phase was separated and the aqueous fraction was extracted with Et2O (3 × 10 mL). The combined organic phases were dried over Na2SO4, filtered, and concentrated under reduced pressure. The residue was purified by silica gel flash column chromatography [gradient eluent: 15-20% EtOAc in hexane] to provide allyl alcohol 15 as a mixture of diastereomers in 68% yield (385.2 mg). 15: Rf = 0.30 [25% EtOAc in hexane]; 1H NMR (300 MHz, CDCl3) δ 1.08 (s, 9H), 1.43 (s, 6H), 1.63-1.81 (m, 4H), 1.90 (brs, 1H), 3.33 (m, 1H), 3.38 (s, 3H), 3.75 (dd, J = 4.5, 11.1 Hz, 1H), 3.88 (dd, J = 3.3, 11.1 Hz, 1H), 4.02 (ddd, J = 4.2, 4.2, 8.1 Hz, 1H), 4.12 (dd, J = 7.2, 7.2 Hz, 2H), 5.11 (dd, J = 1.2, 10.5 Hz, 1H), 5.24 (dt, J = 17.1, 1.5 Hz, 1H), 5.88 (dddd, J = 1.6, 8.0, 11.5, 17.1 Hz, 1H), 7.36-7.45 (m, 6H), 7.69-7.74 (m, 4H); mass spectrum (APCI): m/z (% relative intensity) 499.1 (M+H)+ (100); m/z calcd for C29H42O5SiNa+ 521.2699, found 521.2694.
Removal of Acetonide. A solution of the above allylic alcohol (200.0 mg, 0.40 mmol) in mixture of AcOH (5.60 mL) and H2O (2.40 mL) was heated to 70 oC for 1.5 h. Then the solution was cooled to rt and sat aq NaHCO3 was added slowly until pH is about 7. The mixture was diluted with EtOAc (20 mL). Then organic solvents were separated and the aqueous fraction was extracted with EtOAc (3 × 10 mL). The combined organic phases were washed with sat aq NaCl, dried over Na2SO4, filtered, and concentrated under reduced pressure. The residue was purified by silica gel flash column chromatography [gradient eluent: 40-60% EtOAc in hexane] to provide the pure triol intermediate in 86% yield (233.8 mg). Rf = 0.25 [50 % EtOAc in hexane]; 1H NMR (500 MHz, CDCl3) δ 1.08 (s, 9H), 1.54-1.80 (m, 4H), 2.75-2.95 (br, 3H), 3.38 (m, 1H), 3.38 (s, 3H), 3.67 (dt, J = 6.5, 1.5 Hz, 1H), 3.77 (dd, J = 5.5, 10.0 Hz, 1H), 3.81 (dd, J = 5.5, 10.0 Hz, 1H), 3.90 (dt, J = 1.0, 5.5 Hz, 1H), 4.13 (m, 1H), 5.09 (d, J = 10.5 Hz, 1H), 5.22 (dd, J = 17.5 Hz, 1H), 5.86 (dddd, J = 3.5, 6.0, 9.5, 16.5 Hz, 1H), 7.38-7.44 (m, 6H), 7.67-7.70 (m, 4H); 13C NMR (125 MHz, CDCl3) δ 19.2, 25.5, 26.9, 32.0, 58.3, 66.2, 69.9, 71.7, 72.8, 82.2, 114.6, 127.9, 129.9, 132.8, 135.6, 141.1; IR (film) cm-1 3415brs, 3073m, 2935s, 2861m, 1109s; mass spectrum (APCI): m/z (% relative intensity) 459.2 (M+H)+ (100); m/z calcd for C26H38O5SiNa+ 481.2386, found 481.2390.
MnO2 Oxidation and Formation of Bicyclic Acetal 18. To a solution of the above triol (0.77 mmol) in CH2Cl2 (10 mL) was added MnO2 (10.0 equiv 7.7 mmol) at rt. The solution was sonicated at rt for 6 h. The mixture was filtered through CeliteTM and the residue was washed with CH2Cl2 several times. Then the filtrate was collected and concentrated under reduced pressure. The residue was purified by silica gel flash column chromatography [gradient eluent: 10-15% EtOAc in hexane] to provide diol enone 16, which was used right away. To a solution of 16 (7.76 mg, 0.017 mmol) and 1,6-heptadiene-3-ol (3.90 mg, 0.034 mmol) in CH2Cl2 (0.5 mL) was added Tf2NH (0.1 M in CH2Cl2, 0.34 mL, 0.034 mmol) at –78 oC. The solution was stirred at –78 oC for 5 min before quenched with Et3N (0.2 mL) at –78 oC. The mixture was warmed to rt and filtered through Celite.TM After concentrating the filtrate under reduced pressure, the resulting crude residue (in 81% yield) showed a clean and pure NMR spectrum that could be assigned as bicyclic acetal 18. 18: Rf = 0.70 [10% EtOAc in hexane]; 1H NMR (500 MHz, CDCl3) δ 1.07 (s, 9H), 1.93-1.95 (m, 4H), 3.42 (m, 1H), 3.46 (s, 3H), 3.54 (dd, J = 9.5, 9.5 Hz, 1H), 3.66 (dd, J = 5.0, 10.0 Hz, 1H), 3.99 (d, J = 5.0, 8.5 Hz, 1H), 4.65 (m, 1H), 5.20 (d, J = 11.0 Hz, 1H), 5.45 (d, J = 17.5 Hz, 1H), 5.87 (dd, J = 10.5, 17.5 Hz, 1H), 7.38-7.43 (m, 6 H), 7.64-7.66 (m, 4H); mass spectrum (APCI): m/z (% relative intensity) 439.2 (M+H)+ (100); m/z calcd for C27H34O4SiNa+ 461.2124, found 461.2120.
Synthesis of Acid 20.
To a solution of aldehyde 14 (4.20 g, 8.90 mmol), 2-methyl-2-butene (4.7 mL, 44.5 mmol), and NaH2PO4 (2.46 g, 19.7 mmol) in the mixture of t-BuOH (30 mL) and H2O (15 mL) was added NaClO2 (3.22 g, 35.6 mmol) at –10 oC in 3 portions. The solution was warmed up to rt and stirred for 1 h to give a pale green solution. Then the reaction was quenched with sat aq Na2S2O3 (30 mL). The organic phase was separated and the aqueous fraction was extracted with CH2Cl2 (3 × 30 mL). The combined organic phases were dried over Na2SO4, filtered, and concentrated under reduced pressure. The residue was purified by silica gel flash column chromatography [gradient eluent: 30-80% EtOAc in hexane] to provide the carboxylic acid 20 as colorless oil in 98% yield (4.29 g). 20: Rf = 0.40 [50% EtOAc in hexane]; [α]D23 = - 15.9 [c 4.37, CHCl3]; 1H NMR (500 MHz, CDCl3) δ 1.08 (s, 9H), 1.42 (s, 6H), 1.86-1.94 (m, 2H), 2.49 (ddd, J = 7.0, 16.5, 16.5 Hz, 1H), 2.52 (ddd, J = 7.0, 16.5, 16.5 Hz, 1H), 3.37 (s, 3H), 3.38 (m, 1H), 3.77 (ddd, J = 1.5, 4.5, 11.5 Hz, 1H), 3.88 (ddd, J = 1.5, 4.0, 11.0 Hz, 1H), 4.02 (m, 1H), 4.11 (m, 1H), 7.38-7.43 (m, 6H), 7.70-7.73 (m, 4H), 11.1 (brs, 1H); 13C NMR (125 MHz, CDCl3) δ 19.3, 21.5, 25.1, 27.1, 27.2, 29.6, 58.2, 64.6, 77.8, 79.5, 80.6, 109.3, 127.7, 129.7, 133.2, 135.7, 179.5; IR (film) cm-1 3010brs, 3071m, 2934s, 2861m, 1710s, 1109s; mass spectrum (ESI): m/z (% relative intensity) 509.2 (M+Na)+ (100); m/z calcd for C27H38O6SiNa+ 509.2330, found 509.2335.
Synthesis of Pival-Protected Lactone 21.
Lactone Formation. To a solution of acid 20 (4.20 g, 8.63 mmol) in CH2Cl2 (45 mL) was added p-TsOH-H2O (4.92 g, 25.9 mmol) at 0 oC. The solution was warmed up to rt and stirred for 3 h before quenched with sat aq NaHCO3 (30 mL). The organic phase was separated and the aqueous fraction was extracted with CH2Cl2 (3 × 30 mL). The combined organic phases were dried over Na2SO4, filtered, and concentrated under reduced pressure. The residue was purified by silica gel flash column chromatography [gradient eluent: 40-50% EtOAc in hexane] to provide the unprotected lactone as colorless oil in 83% yield (3.08 g). Rf = 0.20 [50% EtOAc in hexane]; [α]D23 = 48.3 [c 5.24, CHCl3]; 1H NMR (300 MHz, CDCl3) δ 1.09 (s, 9H), 1.82 (ddd, J = 3.9, 6.9, 12.9 Hz, 1H), 2.04-2.16 (m, 1H), 2.36 (dt, J = 18.6, 6.3 Hz, 1H), 2.55 (ddd, J = 17.1, 6.3, 6.3 Hz, 1H), 3.31 (s, 3H), 3.68 (dd, J = 6.0, 10.8 Hz, 1H), 3.79-3.85 (m, 2H), 3.90-3.97 (m, 1H), 4.46 (d, J = 6.0 Hz, 1H), 7.36-7.37 (m, 6H), 7.70-7.72 (m, 4H); 13C NMR (75 MHz, CDCl3) δ 19.2, 20.8, 23.2, 26.8, 56.4, 63.9, 70.6, 72.9, 80.0, 127.7, 129.8, 133.0, 135.4, 170.9; IR (film) cm-1 3440brs, 3071m, 2936s, 2861m, 1738s, 1110s; mass spectrum (ESI): m/z (% relative intensity) 451.2 (M+Na)+ (100); m/z calcd for C24H32O5SiNa+ 451.1911, found 451.1912.
Pivalation. To a solution of the above C22-unprotected lactone (805.7 mg, 1.88 mmol), pyridine (1.6 mL, 18.8 mmol) in CH2Cl2 (10 mL) was added (CH3)3COCl (0.46 mL, 3.8 mmol) followed by DMAP (45.9 mg, 0.38 mmol) at rt. The solution was stirred for overnight and quenched with H2O (10 mL). The organic phase was separated and the aqueous fraction was extracted with CH2Cl2 (3 × 10 mL). The combined organic phases were dried over Na2SO4, filtered, and concentrated under reduced pressure. The residue was purified by silica gel flash column chromatography [gradient eluent: 10-20% EtOAc in hexane] to provide the pivalate-protected lactone 21 as colorless oil in 77% yield (737.0 mg). 21: Rf = 0.65 [50% EtOAc in hexane]; [α]D23 = 26.8 [c 3.45, CHCl3]; 1H NMR (300 MHz, CDCl3) δ 1.08 (s, 9H), 1.24 (s, 9H), 2.06 (dddd, J = 5.1, 5.7, 9.0, 13.8 Hz, 2H), 2.46 (dt, J = 6.0, 17.0 Hz, 1H), 2.69 (ddd, J = 6.6, 9.0, 17.1 Hz, 1H), 3.39 (s, 3H), 3.42 (m, 1H), 3.88 (d, J = 6.6 Hz, 2H), 4.55 (dd, J = 2.4, 6.6 Hz, 1H), 5.33 (dt, J = 2.4, 6.6 Hz, 1H), 7.43-7.48 (m, 6H), 7.69-7.74 (m, 4H); 13C NMR (75 MHz, CDCl3) δ 19.0, 23.1, 26.6, 26.9, 27.0, 38.8, 56.7, 61.4, 71.3, 72.3, 78.6, 127.7, 129.7, 132.7, 135.4, 170.4, 177.5; IR (film) cm-1 3071m, 2936s, 2862m, 1741s, 1151s; mass spectrum (APCI): m/z (% relative intensity) 513.3 (M+H)+ (100); m/z (ESI) calcd for C29H40O6SiNa+ 535.2486, found 535.2489.
Synthesis of Vinyl Ketone and Lactol Mixture 22a/b.
To a solution of the Piv-protected lactone 21 (131.2 mg, 0.256 mmol) in THF (2 mL) was added vinyl magnesium bromide (1.0 M in THF, 0.51 mL, 0.51 mmol) dropwise at –78 oC. The solution was stirred for 1 h at –78 oC and quenched with sat aq NaHCO3 (5 mL) and diluted with CH2Cl2 (5 mL). The organic phase was separated and the aqueous fraction was extracted with CH2Cl2 (3 × 5 mL). The combined organic phases were dried over Na2SO4, filtered, and concentrated under reduced pressure. The residue was purified by silica gel flash column chromatography [gradient eluent: 15-20% EtOAc in hexane] to provide an inseparable mixture of vinyl ketone and lactol 22a/b as colorless oil in 83% yield (114.7.0 mg) and the recovered starting material (19.5 mg).
22a/b: Rf = 0.65 [50 % EtOAc in hexane]; [α]D23 = 9.79 [c 3.36, CHCl3]; 1H NMR (300 MHz, CDCl3) δ 1.05 (s, 9H), 1.25 (s, 9H), 1.45 (d, J = 2.7 Hz, 1H), 1.98-2.06 (m, 2H), 2.62-2.80 (m, 2H), 3.17-3.21 (m, 2H), 3.28 (s, 3H), 3.81 (m, 1H), 3.90 (d, J = 4.5 Hz, 2H), 5.29 (dt, J = 2.5, 7.0 Hz, 1H), 5.83 (d, J = 11.5 Hz, 1H), 6.24 (d, J = 18.0 Hz, 1H), 6.37 (dd, J = 11.0, 18.0 Hz, 1H), 7.40-7.48 (m, 6H), 7.68-7.71 (m, 4H); 13C NMR (75 MHz, CDCl3) δ 18.9, 22.3, 26.6, 27.1, 33.7, 38.9, 57.3, 64.1, 71.3, 78.9, 103.6, 127.7, 127.7, 128.1, 129.8, 129.8, 132.4, 132.6, 135.4, 135.5, 136.3, 177.6, 201.0; IR (film) cm-1 3506brs, 3071m, 2932s, 2858m, 1731s, 1112s; mass spectrum (ESI): m/z (% relative intensity) 563.3 (M+Na)+ (100); m/z calcd for C31H44O6SiNa+ 563.2799, found 563.2810.
Synthesis of Vinyl Cyclic Acetal 25.
To a solution of the vinyl ketone and lactol mixture 22a/b (2.60 mg, 0.0048 mmol) in CH2Cl2 (0.1 mL) was added MS 4Å (10.0 mg), alcohol 24 (4.80 mg, 0.019 mmol) followed by Tf2NH (0.5 M in toluene, 0.012 mL, 0.0024 mmol) at –78 oC. The solution was stirred at –78 oC for 2 min before quenched with Et3N (0.05 mL) at –78 oC. The mixture was warmed to rt and filtered through Celite.TM After evaporating the solvent under reduced pressure, the resulting crude residue was purified by silica gel flash column chromatography [gradient eluent: 10-25% EtOAc in hexane] to provide the key vinyl cyclic acetal 25 in 56% yield based on starting material recovered. 25: Rf = 0.80 [25% EtOAc in hexane]; [α]D23 = 23.5 [c 0.46, CHCl3]; 1H NMR (300 MHz, CDCl3) δ 0.95 (d, J = 6.9 Hz, 3H), 1.04 (s, 9H), 1.28 (s, 9H), 1.37-1.59 (m, 3H), 1.71-1.81 (m, 2H), 1.91-2.00 (m, 2H), 2.90 (m, 1H), 3.23-3.28 (m, 2H), 3.32 (s, 3H), 3.69-3.75 (m, 2H), 3.81 (m, 1H), 3.83 (s, 3H), 4.04 (m, 1H), 4.07 (d, J = 11.5 Hz, 1H), 4.19 (d, J = 11.5 Hz, 1H), 4.87 (d, J = 17.1 Hz, 1H), 4.92 (d, J = 9.9 Hz, 1H), 5.18 (d, J = 12.9 Hz, 1H), 5.46 (d, J = 17.1 Hz, 1H), 5.59 (m, 1H), 5.67 (m, 1H), 5.78 (dd, J = 10.5, 17.1 Hz, 1H), 6.86 (d, J = 8.4 Hz, 2H), 7.14 (d, J = 8.7 Hz, 2H), 7.38-7.43 (m, 6 H), 7.70-7.72 (m, 4H); 13C NMR (75 MHz, CDCl3) δ 15.4, 19.0, 23.0, 26.5, 27.3, 31.5, 34.8, 38.8, 40.3, 55.1, 56.2, 63.7, 66.4, 71.5, 71.8, 73.6, 74.4, 77.1 97.0, 113.5, 114.4, 115.8, 127.5, 127.6, 129.0, 129.5, 133.3, 135.5, 139.5, 140.5, 158.8, 170.4; IR (film) cm-1 3071m, 2932s, 2859m, 1731s, 1513m, 1112s; mass spectrum (ESI): m/z (% relative intensity) 795.6 (M+Na)+ (100); m/z calcd for C46H64O6SiNa+ 795.4263, found 795.4251.
Minor Diene 26: Rf = 0.80 [25% EtOAc in hexane]; [α]D23 = 27.3 [c 0.40, CHCl3]; 1H NMR (300 MHz, CDCl3) δ 1.07 (s, 9H), 1.24 (s, 9H), 2.16 (ddd, J = 3.0, 7.5, 17.7, 1H), 2.52 (ddd, J = 2.4, 2.4, 17.7 Hz, 1H), 3.40 (s, 3H), 3.47 (dt, J = 5.7, 7.5 Hz, 1H), 3.90 (d, J = 6.0 Hz, 2H), 4.18 (dd, J = 3.6, 7.5 Hz, 1H), 4.78 (brt, J = 4.2 Hz, 1H), 5.02 (d, J = 10.8 Hz, 1H), 5.38 (d, J = 17.1 Hz, 1H), 5.47 (dt, J = 3.6, 6.0 Hz, 1H), 6.07 (dd, J = 10.8, 17.1 Hz, 1H), 7.30-7.47 (m, 6 H), 7.70-7.73 (m, 4H); 13C NMR (75 MHz, CDCl3) δ 19.0, 22.4, 26.4, 26.5, 27.1, 56.5, 61.7, 70.4, 71.6, 74.5, 99.0, 112.7, 127.6, 129.6, 131.3, 133.1, 135.4, 149.9, 170.4; IR (film) cm-1 3069m, 2960s, 2856m, 1732s, 1279m, 1157s; mass spectrum (ESI): m/z (% relative intensity) 545.3 (M+Na)+ (100); m/z calcd for C31H42O5SiNa+ 545.2694, found 545.2691.
Lactol 27.
Rf = 0.32 [50% EtOAc in hexane]; 1H NMR (500 MHz, CDCl3) δ 1.08 (s, 9H), 1.85 (m, 1H), 1.91 (m, 1H), 2.60 (ddd, J = 6.5, 9.0, 17.0 Hz, 1H), 2.71 (br, 1H), 2.73 (dddd, J = 6.0, 9.0, 17.0, 17.0 Hz, 1H), 3.28 (s, 3H), 3.38 (ddd, J = 4.5, 4.5, 8.5 Hz, 1H), 3.94 (ddd, J = 0.5, 5.0, 11.0 Hz, 1H), 3.61 (ddd, J = 3.5, 3.5, 7.5 Hz, 1H), 4.01 (ddd, J = 1.0, 3.5, 11.5 Hz, 1H), 4.53 (d, J = 11.5 Hz, 1H), 4.72 (d, J = 11.5 Hz, 1H), 5.80 (dd, J = 1.0, 10.5 Hz, 1H), 6.22 (d, J = 17.5 Hz, 1H), 6.35 (dd, J = 10.5, 17.5 Hz, 1H), 7.27-7.45 (m, 11H), 7.72 (m, 4H); 13C NMR (125 MHz, CDCl3) δ 19.2, 22.7, 26.9, 57.1, 64.2, 70.9, 72.3, 76.9, 79.0, 80.2, 96.5, 127.6, 127.9, 128.4, 129.8, 133.0, 133.2, 135.6, 135.7, 136.6, 138.4; IR (film) cm-1 3459brs, 3069m, 2932s, 2859s, 1681m, 1428m, 1113s; mass spectrum (APCI): m/z (% relative intensity) 529.3 (M-H2O+H)+ (100); m/z calcd for C33H42O5SiNa+ 569.2699, found 569.2697.
Cyclic Acetal 29.
To a solution of lactol 27 (5.00 mg, 0.0094 mmol) in CH2Cl2 (0.1 mL) were added MS 4Å (10.0 mg), 3-butene-1-ol (6.77 mg, 0.094 mmol) followed by Tf2NH (2.64 mg, 0.0094 mmol) at –78 °C. The solution was stirred at –78 oC for 5 min before quenched with Et3N (0.1 mL) at –78oC. The mixture was warmed to rt and filtered through CeliteTM. After evaporation of the solvent under reduced pressure, the resulting crude residue was purified by flash column chromatography on silica gel [Gradient eluent: 2% to 10% EtOAc in hexane] to provide cyclic acetal 29 in 89% yield (5.0 mg). Rf = 0.80 [25% EtOAc in hexane]; [α]D23 = 28.9 [c 0.36, CHCl3]; 1H NMR (500 MHz, CDCl3) δ 1.06 (s, 9H), 1.45 (ddd, J = 4.0, 14.0, 14.0 Hz, 1H), 1.74 (ddd, J = 4.0, 14.0, 24.0 Hz, 1H), 1.88 (ddd, J = 4.0, 4.0, 14.0 Hz, 1H), 1.95 (dddd, J = 4.0, 4.0, 8.0, 8.0 Hz, 1H), 2.26 (m, 2H), 3.23 (s, 3H), 3.25 (ddd, J = 5.0, 10.5, 10.5 Hz, 1H), 3.37 (ddd, J = 7.0, 7.0, 9.5 Hz, 1H), 3.40 (ddd, J = 7.0, 7.0, 9.5 Hz, 1H), 3.76 (d, J = 9.5 Hz, 1H), 3.92 (m, 3H), 4.73 (d, J = 11.5 Hz, 1H), 4.79 (d, J = 11.5 Hz, 1H), 5.00 (dd, J = 11.0 Hz, 1H), 5.05 (d, J = 17.5 Hz, 1H), 5.17 (dd, J = 1.5, 11.0 Hz, 1H), 5.31 (dd, J = 2.0, 17.5 Hz, 1H), 5.70 (dd, J = 11.0, 17.5 Hz, 1H), 5.78 (ddt, J = 10.5, 17.5, 7.0 Hz, 1H), 7.27-7.41 (m, 11H), 7.70 (m, 4H); 13C NMR (125 MHz, CDCl3) δ 18.2, 24.1, 26.9, 34.0, 34.3, 56.0, 60.5, 64.5, 73.1, 73.6, 74.7, 80.9, 97.3, 116.2, 127.2, 127.6, 127.9, 128.2, 129.5, 133.6, 133.8, 135.6, 135.7, 138.8, 139.3; IR (film) cm-1 3071w, 2929s, 2857s, 1456m, 1104s; mass spectrum (ESI): m/z (% relative intensity) 623.3 (M+Na)+ (100); m/z calcd for C37H48O5SiNa+ 623.3169, found 623.3172.
Cyclic Acetal 30.
Rf = 0.80 [25% EtOAc in hexane]; [α]D23 = 46.1 [c 0.33, CHCl3]; 1H NMR (500 MHz, CDCl3) δ 1.06 (s, 9H), 1.48 (ddd, J = 4.0, 13.0, 13.0 Hz, 2H), 1.65 (dddd, J = 4.0, 13.0, 13.0, 13.0 Hz, 2H), 1.81 (m, 2H), 1.95 (m, 2H), 2.29 (ddd, J = 6.0, 6.0, 6.0 Hz, 1H), 3.15 (ddd, J = 4.5, 10.5, 10.5 Hz, 1H), 3.21 (s, 3H), 3.39 (m, 5H), 3.49 (dd, J = 7.0, 14.0 Hz, 1H), 3.68 (d, J = 9.5 Hz, 1H), 3.92 (m, 4H), 4.74 (s, 2H), 5.00 (d, J = 10.5 Hz, 1H), 5.02 (dd, J = 1.0, 11.0 Hz, 1H), 5.06 (d, J = 18.0 Hz, 1H), 5.07 (dd, J = 1.5, 17.5 Hz, 1H), 5.79 (ddt, J = 10.0, 17.0, 7.0 Hz, 2H), 7.27-7.41 (m, 11H), 7.69 (m, 4H); 13C NMR (125 MHz, CDCl3) δ 19.2, 23.9, 26.9, 32.3, 34.2, 34.4, 36.1, 56.0, 59.2, 64.4, 66.7, 70.2, 73.1, 73.7, 74.8, 80.7, 98.0, 116.3, 116.4, 127.2, 127.6, 129.2, 129.5, 133.6, 133.8, 135.3, 135.5, 135.6, 139.2; IR (film) cm-1 3071m, 2931s, 2858s, 1428m, 1105s; mass spectrum (ESI): m/z (% relative intensity) 695.6 (M+Na)+ (100); m/z calcd for C41H56O6SiNa+ 695.3744, found 695.3740.
Diene 31.
Rf = 0.80 [25% EtOAc in hexane]; [α]D23 = -5.87 [c 0.92, CHCl3]; 1H NMR (300 MHz, CDCl3) δ 1.08 (s, 9H), 2.17 (t, J = 3.9 Hz, 2H), 3.40 (s, 3H), 3.74 (ddd, J = 3.0, 5.1, 10.2 Hz, 1H), 3.79 (ddd, J = 7.5, 7.5, 7.5 Hz, 1H), 3.93 (dd, J = 5.4, 11.4 Hz, 1H), 4.01 (dd, J = 3.3, 11.4 Hz, 1H), 4.35 (dd, J = 14.5, 6.9 Hz, 1H), 4.56 (d, J = 11.8 Hz, 1H), 4.71 (dd, J = 3.9, 3.9 Hz, 1H), 4.84 (d, J = 11.8 Hz, 1H), 4.97 (d, J = 10.8 Hz, 1H), 5.40 (d, J = 17.1 Hz, 1H), 6.01 (dd, J = 10.8, 17.1 Hz, 1H), 7.43 (m, 11H), 7.75 (m, 4H); 13C NMR (75 MHz, CDCl3) δ 19.1, 24.0, 26.7, 56.4, 63.4, 71.6, 72.5, 73.9, 78.0, 99.4, 112.5, 127.5, 127.6, 127.8, 128.2, 129.5, 131.6, 133.3, 135.6, 138.3, 148.9; IR (film) cm-1 3070m, 2931s, 2858s, 1428m, 1112s; mass spectrum (APCI): m/z (% relative intensity) 529.2 (M+H)+ (100); m/z calcd for C33H40O4SiNa+ 551.2594, found 551.2596.
BC-Ring 32 via RCM of Cyclic Acetal 25.
To a 0.01 M solution of cyclic acetal 25 (34.8 mg, 0.45 mmol) in toluene was added Grubbs Generation-II Ru-catalyst (0.30 equiv) at rt and the mixture was stirred for 8 h until cyclic acetal 25 was consumed. The suspension was concentrated under reduced pressure and the residue was purified with silica gel flash column chromatography [isocratic eluent: 15% EtOAc in hexane] to provide C11-C23 fragment 32, colorless oil, in 95% yield. The product yield was based on the amount of cyclic acetal compound 32 and the ratio between cyclic acetal and side product was figured out by 1H NMR analysis. 32: Rf = 0.50 [20% EtOAc in hexane]; [α]D23 = - 9.88 [c 0.24, CHCl3]; 1H NMR (500 MHz, CDCl3) δ 0.84 (d, J = 9.0 Hz, 3H), 1.02 (s, 9H), 1.26 (s, 9H), 1.52-1.75 (m, 4H), 1.85 (m, 1H), 2.00 (m, 2H), 2.94 (ddd, J = 2.5, 10.5, 10.5 Hz, 1H), 3.27 (s, 3H), 3.28-3.34 (m, 2H), 3.51 (ddd, J = 5.0, 9.0, 9.0 Hz, 1H), 3.57 (dd, J = 2.5, 9.5 Hz, 1H), 3.76 (ddd, J = 3.5, 10.5, 10..5 Hz, 1H), 3.79 (s, 3H), 3.92 (d, J = 9.0, 11.0 Hz, 1 H), 4.12 (d, J = 11.5 Hz, 1H), 4.16 (d, J = 11.5 Hz, 1H), 5.48 (dd, J = 2.5, 10.0 Hz, 1H), 5.54 (ddd, J = 3.0, 3.0, 8.5 Hz, 1H), 5.61 (dd, J = 1.5, 10.0 Hz, 1H), 6.84 (d, J = 8.5 Hz, 2H), 7.14 (d, J = 8.5 Hz, 2H), 7.32-7.37 (m, 6 H), 7.67-7.68 (m, 4H); 13C NMR (125 MHz, CDCl3) δ 16.9, 19.4, 23.9, 26.9, 27.7, 33.3, 33.8, 34.5, 39.2, 55.5, 56.5, 64.3, 67.0, 71.2, 71.9, 72.7, 74.6, 93.5, 113.9, 127.8, 128.6, 129.7, 130.0, 130.9, 134.7, 135.9, 159.3, 177.8; IR (film) cm-1 3070m, 2959s, 2859m, 1731s, 1513m, 1101s; mass spectrum (ESI): m/z (% relative intensity) 767.4 (M+Na)+ (100); m/z calcd for C44H60O6SiNa+ 767.3950, found 767.3947.
Mukaiyama Aldol Reaction
Silyl Enol Ether Formation Using 11. To a cooled solution of i-Pr2NH (41.6 μL, 0.294 mmol) in THF (2 mL) was added n-BuLi (1.6 M in hexane, 172.0 μL, 0.274 mmol) at 0 oC. The solution was stirred for 10 min to give the LDA solution (0.5 M in THF) for next step. To the LDA solution was added pyran 11 via cannula at –78 oC and the mixture was stirred for 30 min. Chlorotrimethylsilane (35.0 μL.294 mmol) was then added the lithium enolate and the reaction mixture was stirred for 30 min. The reaction mixture was gradually warmed up to 0 oC and was quenched with sat aq NaHCO3 solution (3 mL). The organic phase was extracted with EtOAc, dried over Na2SO4, and concentrated under reduced pressure. The crude trimethylsilyl enol ether was used for the next step without further purification.
Aldol Addition. To a cooled solution of the above trimethylsilyl enol ether (0.098 mmol) with either aldehyde 33a [or 33b] (0.049 mmol) in CH2Cl2 (1 mL) was added BF3-Et2O (1.08 μL, 0.062 mmol) at –78 oC dropwise. The reaction was stirred at –78 oC for 1 h before sat aq NaHCO3 (3 mL) was added to quench the reaction. After the mixture was warmed up to room temperature, the organic phase was separated and the aqueous fraction was extracted with CH2Cl2 (3 × 3 mL). The combined organic phases were dried over Na2SO4 and concentrated under reduced pressure. The crude residue was purified with flash silica gel column chromatography [25% EtOAc in hexane] to provide respective Mukaiyama-aldol addition product 34 and 35. 34: For the major isomer: Rf = 0.65 [30% EtOAc in hexane]; 1H NMR (500 MHz, CDCl3) δ 0.08 (s, 3H), 0.11 (s, 3H), 0.90 (s, 9H), 0.99 (d, J = 7.0 Hz, 3H), 1.19 (s, 9H), 1.21 (m, 2H), 1.44 (dt, J = 3.0, 9.5 Hz, 2H), 1.49-1.61 (m, 4H), 1.74 (dd, J = 2.0, 13.0 Hz, 2H), 1.84 (m, 1H), 2.37-2.46 (m, 2H), 2.60 (m, 1H), 2.66 (dd, J = 8.0, 15.0 Hz, 1H), 3.20 (brd, J = 4.0 Hz, 1H), 3.38-3.42 (m, 1H), 3.75-3.80 (m, 1H), 3.94 (ddd, J = 4.0, 4.0, 8.0 Hz, 1H), 4.10 (ddd, J = 6.0, 11.0, 11.0 Hz, 1H), 4.13 (ddd, J = 7.0, 10.5, 10.5 Hz, 1H), 4.23 (ddd, J = 4.0, 8.0, 12.5 Hz, 1H), 5.01 (d, J = 9.0 Hz, 1H), 5.02 (d, J = 18.0 Hz, 1H), 5.77 (ddd, J = 7.0, 10.0, 17.0 Hz, 1H); 13C NMR (125 MHz, CDCl3) δ -4.5, -4.7, 13.2, 17.3, 23.0, 25.5, 26.8, 27.3, 30.7, 30.9, 34.9, 39.6, 39.9, 43.0, 51.0, 60.9, 64.0, 71.8, 74.1, 74.5, 114.9, 140.6, 178.0, 209.6; IR (neat) cm-1 3751m, 2956s, 2936s, 2870s, 2342s, 1719s, 1702s; mass spectrum (MALDI): m/z (% relative intensity) 535.5 (M+Na)+ (100); m/z calcd for C28H52O6SiNa+ 535.3431, found 535.3430. 35: For the major isomer: Rf = 0.20 [25% EtOAc in hexane]; 1H NMR (500 MHz, CDCl3) δ 1.03 (d, J = 7.0 Hz, 3H), 1.19 (s, 9H), 1.20-1.32 (m, 2H), 1.48-1.61 (m, 6H), 1.73 (q, J = 6.5, 13.0 Hz, 2 H), 1.83 (m, 1H), 2.38 (dd, J = 5.0, 15.0 Hz, 1H), 2.56 (m, 2H), 2.64 (dd, J = 8.0, 15.0 Hz, 1H), 3.26 (d, J = 3.5 Hz, 1H), 3.39 (dddd, J = 1.0, 6.5, 6.5, 12.5 Hz, 1H), 3.69 (dddd, J = 1.5, 4.0, 4.0, 8.0 Hz, 1H), 3.75 (m, 1H), 3.81 (s, 3H), 4.11 (ddd, J = 7.0, 7.0, 12.5 Hz, 2H), 4.26 (m, 1H), 4.51 (d, J = 10.5 Hz, 1H), 4.58 (d, J = 10.5 Hz, 1H), 5.03 (ddd, J = 1.5, 1.5, 7.5 Hz, 1H), 5.06 (ddd, J = 1.5, 1.5, 17.5 Hz, 1H), 5.81 (ddd, J = 7.5, 10.5, 17.5 Hz, 1H), 6.87 (d, J = 9.0 Hz, 2H), 7.28 (d, J = 8.5 Hz, 2H); 13C NMR (125 MHz, CDCl3) δ 14.1, 23.5, 27.3, 31.2, 35.4, 37.5, 38.8, 40.4, 50.1, 51.2, 55.4, 61.0, 64.8, 72.2, 74.4, 79.0, 113.9, 114.8, 129.6, 131.0, 140.8, 159.3, 178.6, 210.4; IR (neat) cm-1 3871m, 3751m, 3677m, 3651m, 3494brs, 2956s, 2936s, 2870s, 2342s, 1719s, 1702s, 1616s; mass spectrum (MALDI): m/z (% relative intensity) 541(M+Na+) (100), 518 (M+) (4), 409 (4), 321 (10), 273 (39); m/z calcd for C30H46O7Na+ 541.3141, found 541.3144.
Directed Reduction for Synthesis of 1,3-Anti-Diols.
To a cooled solution of Me4NB(OAc)3H (26.0 mg, 0.099 mmol) in anhyd CH3CN (0.07 mL) was added anhyd HOAc (0.07 mL) at rt. After stirring for 30 min, the solution was cooled to –30 oC and a solution of the above ketone 34 or 35 (0.017 mmol) in CH3CN (0.5 mL) was added via cannula. The mixture was stirred at –30 oC for 6 h before being warmed up to rt and stirred for another 6 h. After which, aq sodium potassium tartrate solution (0.5 M, 1 mL) was added to the reaction followed by sat aq NaHCO3 (5 mL) and CH2Cl2 (5 mL). The organic phase was separated and the aqueous fraction was extracted with CH2Cl2 (3 × 3 mL). The combined organic phases were dried over Na2SO4 and concentrated under reduced pressure. The crude residue was purified by flash silica gel column chromatography to provide the pure respective 1,3-anti-diol 36 or 37. 36 with TBS protection: Rf = 0.40 [25% EtOAc in hexane]; 1H NMR (400 MHz, CDCl3) δ 0.08 (s, 3H), 0.12 (s, 3H), 0.90 (s, 9H), 0.99 (d, J = 6.8 Hz, 3H), 1.20 (s, 9H), 1.41-1.69 (m, 10H), 1.75-1.85 (m, 4H), 2.37 (qdd, J = 0.8, 6.8, 12.8 Hz, 1H), 3.46 (dddd, J = 1.6, 6.0, 6.0, 13.2 Hz, 1H), 3.59 (dddd, J = 2.8, 2.8, 10.0, 10.0 Hz, 1H), 3.88 (br, 1H), 3.93 (ddd, J = 4.4, 4.4, 7.2 Hz, 1H), 4.00 (m, 1H), 4.06 (dddd, J = 2.8, 2.8, 10.0, 10.0 Hz, 1H), 4.13 (t, J = 6.0 Hz, 2H), 5.01 (dd, J = 0.8, 10.0 Hz, 1H), 5.02 (dd, J = 1.2, 11.6 Hz, 1H), 5.78 (ddd, J = 7.2, 10.4, 17.2 Hz, 1H); mass spectrum (MALDI): m/z (% relative intensity) 537.5 (M+Na)+ (23); 515.5 (M+H)+ (100); m/z calcd for C28H54O6SiNa+ 537.3587, found 537.3690. 37 with PMB protection: Rf = 0.35 [33% EtOAc in hexane]; 1H NMR (500 MHz, CDCl3) δ 1.03 (d, J = 6.5 Hz, 3H), 1.19 (s, 9H), 1.21-1.35 (m, 2H), 1.46-1.62 (m, 6H), 1.65-1.72 (m, 2H), 1.75 (m, 2H), 1.82 (m, 1H), 2.60 (m, 1H), 3.32 (m, 1H), 3.59 (brdt, J = 2.0, 7.0 Hz, 1H), 3.68 (dddd, J = 3.5, 5.0, 8.5, 12.0 Hz, 1H), 3.80 (s, 3H), 4.02 (m, 1H), 4.08 (m, 1H), 4.12-4.18 (m, 2H), 4.27 (m, 1H), 4.40 (dt, J = 7.5, 11.0 Hz, 1H), 4.48 (d, J = 11.0 Hz, 1H), 4.57 (d, J = 10.5 Hz, 1H), 5.03 (dd, J = 1.0, 11.5 Hz, 1H), 5.08 (dd, J = 1.5, 17.5 Hz, 1H), 5.41 (q, J = 5.0 Hz, 1H), 5.82 (ddd, J = 7.0, 10.5, 17.5 Hz, 1H), 6.86 (d, J = 8.5 Hz, 2H), 7.28 (d, J = 8.5 Hz, 2H); 13C NMR (125 MHz, CDCl3) δ 14.1, 19.9, 25.4, 29.8, 33.7, 34.5, 37.5, 38.5, 39.8, 44.0, 55.0, 62.0, 66.5, 74.0, 75.5, 76.0, 79.9, 114.0, 115.0, 129.8, 131.4, 141.2, 159.9, 179.2; mass spectrum (MALDI): m/z (% relative intensity) 543.5 (M+Na)+ (100); m/z calcd for C30H48O7Na+ 543.3298, found 543.3301.
Standard Conditions as Described for the Acetonide Formation.
C13-TBS-Protected C9,11-Anti-Acetonide 38: Rf = 0.42 [10% EtOAc in hexane]; 1H NMR (400 MHz, CDCl3) δ 0.09 (s, 6H), 0.89 (s, 9H), 0.99 (d, J = 8.5 Hz, 1H), 1.18 (s, 9H), 1.19 (m, 2H), 1.31 (s, 3H), 1.33 (s, 3H), 1.41 (m, 1H), 1.48-1.62 (m, 10H), 1.72-1.83 (m, 3H), 2.33 (m, 1H), 3.74 (m, 1H), 3.46 (m, 1H), 3.76 (ddd, J = 5.0, 5.0, 9.5 Hz, 1H), 3.86 (m, 1H), 4.06 (m, 1H), 3.16 (m, 1H), 4.21 (m, 1H), 5.02 (dd, J = 2.5, 22.0 Hz, 1H), 5.03 (dd, J = 1.0, 14.5 Hz, 1H), 5.79 (ddd, J = 9.0, 15.0, 22.0 Hz, 1H); 13C NMR (100 MHz, CDCl3) δ 0.2, 14.5, 18.4, 23.9, 25.1, 25.6, 26.2, 27.4, 32.1, 35.2, 36.0, 38.9, 39.6, 40.5, 42.9, 43.6, 61.5, 62.9, 64.2, 72.6, 74.1, 74.7, 100.3, 114.8, 140.7, 179.6; mass spectrum (APCI): m/z (% relative intensity) 555.4 (M+H)+ (100); m/z calcd for C31H58O6SiNa+ 577.3900, found 577.3895.
Standard TBAF-Desilylation Conditions. 39: Rf = 0.40 [25% EtOAc in hexane]; 1H NMR (400 MHz, CDCl3) δ 1.05 (d, J = 6.8 Hz, 3H), 1.19 (s, 9H), 1.34 (s, 3H), 1.35 (s, 3H), 1.50-1.78 (m, 12H), 1.80-1.86 (m, 2H), 2.21 (dd, J = 6.8, 6.8 Hz, 1H), 2.75 (brs, 1H), 3.33 (m, 1H), 3.39 (m, 1H), 3.70 (m, 1H), 4.07-4.12 (m, 3H), 4.18-4.26 (m, 1H), 5.07 (d, J = 16.8 Hz, 1H), 5.08 (d, J = 11.2 Hz, 1H), 5.83 (ddd, J = 8.0, 11.6, 15.6 Hz, 1H); mass spectrum (APCI): m/z (% relative intensity) 441.1 (M+H)+ (100); m/z calcd for C25H44O6Na+ 463.3036, found 463.3034.
Standard DDQ Conditions [See Below]. PMP-Acetal 40. Rf = 0.30 [25% EtOAc in hexane]; 1H NMR (500 MHz, CDCl3) δ 1.08 (d, J = 7.0 Hz, 3H), 1.19 (s, 9H), 1.40-1.56 (m, 4H), 1.62-1.68 (m, 3H), 1.75-1.79 (m, 2H), 1.80-1.90 (m, 2H), 2.10 (m, 1H), 2.28 (m, 1H), 2.36 (m, 1H), 3.32 (ddd, J = 5.5, 5.5, 10.5 Hz, 1H), 3.45 (m, 1H), 3.61 (m, 1H), 3.79 (s, 3H), 3.86 (m, 1H), 4.04 (ddd, J = 5.5, 5.5, 11.0 Hz, 1H), 4.14 (ddd, J = 6.5, 6.5, 11.5 Hz, 1H), 4.20 (br, 1H), 4.37 (ddd, J = 7.0, 7.0, 11.0 Hz, 1H), 4.53 (q, J = 5.0 Hz, 1H), 5.05 (dd, J = 1.0, 9.5 Hz, 1H), 5.06 (dd, J = 1.0, 23.5 Hz, 1H), 5.72 (s, 1H), 5.91 (ddd, J = 8.0, 10.5, 25.0 Hz, 1H), 6.87 (d, J = 9.0 Hz, 2H), 7.41 (d, J = 8.5 Hz, 2H); 13C NMR (125 MHz, CDCl3) δ 15.5, 23.9, 27.5, 31.6, 36.0, 38.3, 39.0, 42.8, 43.4, 55.6, 61.0, 65.6, 70.1, 74.7, 75.5, 76.0, 94.3, 113.8, 115.1, 127.6, 132.1, 140.6, 160.0, 178.9; mass spectrum (APCI): m/z (% relative intensity) 519.3 (M+H)+; m/z calcd for C30H46O7Na+ 541.3141, found 541.3145.
Synthesis of Aldehyde 43.
PMB Deprotected Via DDQ. To a solution of 32 (130.4 mg, 0.17 mmol) in CH2Cl2 : H2O (10:1) was added DDQ (0.25 mmol, 1.5 equiv) at rt. After being stirred for 1.5 h, the mixture was quenched by sat aq NaHCO3. The mixture was extracted with CH2Cl2, dried over MgSO4, filtered and concentrated in vacuo. The residue was purified by silica gel flash column chromatography [gradient eluent: 33-50% EtOAc in hexane] to provide the desired primary alcohol (94.8 mg, 0.15 mmol) in 88% yield as a colorless oil. Rf = 0.30 [33% EtOAc in hexane]; [α]D23 = + 3.50 [c 0.84, CH2Cl2]; 1H NMR (500 MHz, CDCl3) δ 0.87 (d, J = 9.0 Hz, 3H), 1.04 (s, 9H), 1.25 (s, 9H), 1.51-1.69 (m, 3H), 1.72-1.89 (m, 3H), 2.01-2.12 (m, 2H), 2.96 (ddd, J = 4.0, 10.0, 10.0 Hz, 1H), 3.25 (s, 3H), 3.45 (dt, J = 2.8, 9.6 Hz, 1H), 3.61 (t, J = 4.4 Hz, 2H), 3.69 (dd, J = 2.8, 9.6 Hz, 1H), 3.80 (dd, J = 4.4, 10.8 Hz, 1H), 3.88 (dd, J = 8.0, 10.8 Hz, 1H), 5.45 (ddd, J = 2.4, 4.4, 7.2 Hz, 1H), 5.49 (dd, J = 2.8, 10.0 Hz, 1H), 5.64 (dd, J = 2.0, 10.0 Hz, 1H), 7.32-7.37 (m, 6 H), 7.67-7.68 (m, 4H); 13C NMR (125 MHz, CDCl3) δ 16.8, 19.4, 24.0, 27.0, 27.6, 33.7, 34.2, 35.0, 39.2, 56.4, 60.1, 63.8, 71.6, 72.5, 72.7, 74.5, 93.8, 127.8, 128.6, 129.8, 133.9, 134.7, 135.9, 177.9; IR (neat) cm-1 (neat) 3524w, 3073w, 2958s, 2933s, 2858brs, 2361s, 2342s, 1731s, 1699w, 1160s, 1107s; mass spectrum (MALDI): m/z (% relative intensity) 647.3 (M+Na)+ (100); m/z calcd for C36H52O7SiNa+ 647.3375, found 647.3352.
SO3·Pyridine Oxidation. To a solution of the above primary alcohol (94.8 mg, 0.15 mmol) in DMSO/CH2Cl2 were added SO3·pyridine (96.8 mg, 0.61 mmol) and Et3N (0.12 mL, 0.76 mmol) sequentially in this order at 0 ºC and the resulting mixture was stirred for 2 h at that temperature. The residue was purified by silica gel flash column chromatography [isocratic: 25% EtOAc in hexane] to provide C11-C23 fragment aldehyde 43 (101.2 mg, 0.132 mmol) in 90% yield. 43: Rf = 0.50 [25% EtOAc in hexane]; [α]D23 = + 7.50 [c 0.24, CH2Cl2]; 1H NMR (400 MHz, CDCl3) δ 0.86 (d, J = 7.2 Hz, 3H), 1.04 (s, 9H), 1.24 (s, 9H), 1.55 (dd, J = 4.4, 13.2 Hz, 1H), 1.61-1.69 (m, 1H), 1.73 (ddd, J = 3.2, 3.2, 8.4, 1H), 1.98 (m, 1H), 2.07 (m, 1H), 2.37 (ddd, J = 3.2, 8.4, 16.0 Hz, 1H), 2.48 (ddd, J = 1.2, 3.6, 16.0 Hz, 1H), 2.96 (dt, J = 4.4, 10.0 Hz, 1H), 3.24 (s, 3H), 3.67 (dd, J = 2.4, 9.6 Hz, 1H), 3.77 (ddd, J = 3.6, 8.4, 9.6 Hz, 1H), 3.86 (dd, J = 4.0, 7.2 Hz, 2H), 5.47 (ddd, J = 2.8, 5.6, 8.0 Hz, 1H), 5.53 (dd, J = 2.8, 10.0 Hz, 1H), 5.64 (dd, J = 1.6, 10.0, Hz, 1H), 7.35-7.41 (m, 6H), 7.66-7.69 (m 4H), 9.61 (dd, J = 1.2, 3.6 Hz, 1H); 13C NMR (100 MHz, CDCl3) δ 16.6, 19.5, 23.6, 27.1, 27.7, 33.7, 34.1, 39.2, 46.8, 56.4, 60.1, 69.7, 71.7, 72.0, 74.4, 93.9, 127.8, 128.9, 129.6, 129.8, 133.8, 135.8, 177.7, 201.1; IR (neat) cm-1 (neat) 3457w, 3074w, 2962s, 2935s, 2860brs, 2724w, 2362w, 2345w, 1733s, 1163s, 1108s; mass spectrum (MALDI): m/z (% relative intensity) 645.3 (M+Na)+ (100); m/z calcd for C36H50O7SiNa+ 645.3218, found 645.3250.
Assembly of The C1-C23 Fragment 44.
Mukaiyama Aldol: To a cooled mixture of the silyl enol ether prepared as described above using pyran 11 (0.189 mmol) and aldehyde 43 (101.0 mg, 0.13 mmol) in CH2Cl2 (1 mL) was added BF3-Et2O (47.3 mL, 0.37 mmol) at –78 oC dropwise. The reaction was stirred at –78 oC for 1 h and then sat aq NaHCO3 (3 mL) was added to quench the reaction. After the mixture was warmed up to room temperature, the organic phase was separated and the aqueous fraction was extracted with CH2Cl2. The combined organic phases were dried over Na2SO4 and concentrated under reduced pressure. The residue was purified with silica gel flash column chromatography [gradient eluent: 2-10% EtOAc in hexane] to provide the desired C1-C23 fragment 44 (62.4 mg, 0.069 mmol) in 62% yield (colorless oil) as a single isomer. 44: Rf = 0.50 [33% EtOAc in hexane]; [α]D23 = - 15.2 [c 0.43, CH2Cl2]; 1H NMR (500 MHz, CDCl3) δ 0.086 (d, J = 7.0 Hz, 3H), 1.02 (s, 9H), 1.18 (s, 9H), 1.24 (s, 9H), 1.40-1.47 (m, 1H), 1.50-1.71 (m, 8H), 1.72-1.80 (m, 4H), 1.86 (m, 1H), 2.02 (m, 1H), 2.10 (dd, J = 2.5, 8.0 Hz, 1H), 2.42 (dd, J = 5.5, 16.0, 1H), 2.54 (d, J = 5.5 Hz, 1H), 2.65 (dd, J = 7.5, 15.5 Hz, 1H), 2.94 (ddd, J = 4.5, 10.0, 10.0 Hz, 1H), 3.03 (d, J = 3.0 Hz, 1H), 3.23 (s, 3H), 3.41 (dddd, J = 1.0, 7.5, 7.5, 7.5 Hz, 1H), 3.53 (td, J = 1.0, 8.0 Hz, 1H), 3.69 (dd, J = 2.0, 9.5 Hz, 1H), 3.76 (dddd, J = 5.5, 5.5, 5.5, 5.5 Hz, 1H), 3.86 (dd, J = 4.0, 11.0 Hz, 1H), 3.88 (dd, J = 4.0, 9.5 Hz, 1H), 4.12 (brd-t, J = 6.5 Hz, 2H), 4.24 (m, 1H), 5.45 (ddd, J = 2.0, 4.0, 4.0 Hz, 1H), 5.47 (dd, J = 2.5, 10.0 Hz, 1H), 5.63 (d, J = 10.0 Hz, 1H), 7.35-7.41 (m, 6H), 7.66-7.69 (m 4H); 13C NMR (125 MHz, CDCl3) δ 16.9, 19.5, 23.7, 24.0, 27.1, 27.5, 27.7, 31.5, 31.6, 33.7, 33.9, 35.6, 39.0, 39.2, 39.3, 50.5, 51.2, 56.4, 61.3, 63.9, 64.3, 71.4, 71.7, 72.6, 74.4, 74.5, 74.9, 93.8, 127.8, 128.5, 129.7, 133.9, 134.8, 135.9, 178.0, 178.7, 209.4; IR (neat) cm-1 2957brs, 2933s, 2860brs, 2361s, 2342s, 1728s, 1157s; mass spectrum (ESI): m/z (% relative intensity) 915.7 (M+Na)+ (80), 910.7 (60), 640.6 (50), 563.5 (100); m/z calcd for C51H76O11SiNa+ 915.5055, found 915.5050.
Completion of Assembly of C9,11-Anti-Acetonide 42.
Directed Reduction. To a solution of tetramethylammonium triacetoxyborohydride (85.1 mg, 0.036 mmol) in anhyd acetonitrile (1.8 mL) and anhyd acetic acid (1.8 mL) at –30 ºC was added a solution of β–hydroxy ketone 44 (40.5 mg, 0.045 mmol) in anhyd acetonitrile (1 mL). After the stirring for 12 h, the reaction mixture was quenched with sat aq NaHCO3 with an additional stirring of 30 min. The mixture was extracted with CH2Cl2. The combined organic layers are dried over Na2SO4, filtered, concentrated under reduced pressure and the desired diol 45 [C9,11-anti] was isolated by silica gel flash column chromatography [gradient eluent: 25-50% EtOAc in hexane]. However, the undesired diol 46 [C9,11-syn] was also isolated. The combined yield was 91% with the anti:syn ratio being 3:1. The dr reflects the isolated ratio. We tried to figure out the ratio according to the crude proton NMR but it was not clear by integration.
C9,11-Anti-Diol 45: Rf = 0.40 [25% EtOAc in hexane]; [α]D23 = - 7.69 [c 0.52, CH2Cl2]; 1H NMR (400 MHz, CDCl3) δ 0.86 (d, J = 9.0 Hz, 3H), 1.02 (s, 9H), 1.19 (s, 9H), 1.23 (s, 9H), 1.45-1.60 (m, 10H), 1.63-1.70 (m, 3H), 1.72-1.80 (m, 5H), 1.85 (m, 1H), 2.02 (m, 1H), 2.18 (ddd, J = 2.0, 5.6, 5.6 Hz, 1H), 2.96 (ddd, J = 4.8, 4.8, 4.8 Hz, 1H), 3.22 (s, 3H), 3.35 (dddd, J = 6.0, 6.0, 11.6, 11.6 Hz, 1H), 3.56-3.61 (m, 2H), 3.77 (dd, J = 2.8, 9.6 Hz, 1H), 3.87 (d, J = 5.2 Hz, 1H), 3.88 (d, J = 6.8 Hz, 1H), 4.07 (ddd, J = 5.6, 5.6, 11.2 Hz, 1H), 4.14-4.22 (m, 2H), 4.32 (ddd, J = 6.0, 6.0, 10.8 Hz, 1H), 5.40 (ddd, J = 2.4, 5.2, 5.2 Hz, 1H), 5.48 (dd, J = 2.4, 10.0 Hz, 1H), 5.65 (dd, J = 2.0, 9.6 Hz, 1H), 7.34-7.40 (m, 6H), 7.66-7.68 (m, 4H); 13C NMR (125 MHz, CDCl3) δ 17.0, 19.5, 21.3, 23.9, 27.1, 27.5, 27.7, 31.5, 31.8, 33.4, 33.8, 35.9, 39.3, 39.5, 43.3, 44.6, 56.3, 60.7, 61.1, 63.6, 65.4, 65.8, 71.4, 71.9, 72.9, 74.6, 74.8, 75.7, 93.9, 127.8, 128.4, 129.8, 134.0, 135.0, 135.9, 178.0, 178.9; IR (neat) cm-1 2934 brs, 2859brs, 2361s, 2341s, 1728s, 1157s, 1105s; mass spectrum (MALDI): m/z (% relative intensity) 917.7 (M+Na)+ (100); m/z calcd for C51H78O11SiNa+ 917.5206, found 917.5192.
C9,11-Syn-Diol 46: Rf = 0.50 [25% EtOAc in hexane]; [α]D23 = - 4.18 [c 0.41, CH2Cl2]; 1H NMR (500 MHz, CDCl3) δ 0.85 (d, J = 9.0 Hz, 3H), 1.02 (s, 9H), 1.19 (s, 9H), 1.24 (s, 9H), 1.40-1.90 (m, 18H), 2.02 (m, 2H), 2.92 (dt, J = 6.0, 12.5 Hz, 1H), 3.24 (s, 3H), 3.46-3.53 (m, 2H), 3.58 (m, 1H), 3.70 (dd, J = 3.5, 12.5 Hz, 1H), 3.91 (brd, J = 8.0 Hz, 2H), 3.97-4.03 (m, 2H), 4.15 (m, 1H), 4.17 (dd, J = 15.0, 15.0 Hz, 2H), 5.47 (br-dd, J = 2.4, 10.0 Hz, 2H), 5.64 (dd, J = 2.0, 10.0 Hz, 1H), 7.33-7.39 (m, 6H), 7.65-7.68 (m, 4H); 13C NMR (125 MHz, CDCl3) δ 17.0, 19.4, 23.5, 23.9, 27.0, 27.4, 27.7, 31.3, 32.0, 34.0, 35.6, 38.9, 39.2, 41.0, 43.0, 56.4, 61.2, 64.1, 68.5, 70.1, 71.4, 71.7, 72.3, 72.6, 74.6, 75.2, 76.9, 79.0, 93.6, 127.7, 128.5, 129.7, 133.9, 134.8, 135.9, 178.5, 178.7; IR (neat) cm-12934 brs, 2859brs, 2361s, 2341s, 1728s, 1157s, 1105s; mass spectrum (MALDI): m/z (% relative intensity) 917.8 (M+Na)+ (100); m/z calcd for C51H78O11SiNa+ 917.5206, found 917.5164.
Acetonide Protection. To a solution of C9,11-anti-diol 45 (18.3 mg, 0.021 mmol) in acetone (4.8 mL) was added a catalytic amount of pyridinium p-toluene sulfonate (1.76 mg, 0.007 mmol) and 2,2-dimethoxypropane (10.0 µL, 0.063 mmol) and the resulting mixture was stirred over 1 h. The reaction was quenched by sat aq NaHCO3 and the organic layer was extracted with CH2Cl2. The combined organic layer was dried over Na2SO4, filtered, and concentrated under reduced pressure. The desired C9,11-anti-acetonide 42 was isolated by silica gel flash column chromatography [gradient eluent: 20-50% EtOAc in hexane] in 85% yield (15.7 mg, 0.018 mmol). Also, C9,11-syn-diol 46 (7.20 mg, 0.008 mmol) was transformed into the corresponding C9,11-syn-acetonide 47 using the same reaction protocol in 90% yield (6.95 mg, 0.007 mmol).
C9,11-Anti-Acetonide 42. Rf = 0.50 [20% EtOAc in hexane]; [α]D23 = - 2.70 [c 0.08, CH2Cl2]; 1H NMR (400 MHz, CDCl3) δ 0.84 (d, J = 7.2 Hz, 3H), 1.00, (s, 9H), 1.10 (s, 3H), 1.11 (s, 3H), 1.19 (s, 9H), 1.26 (s, 9H), 1.37-1.60 (m, 8H), 1.60-1.95 (m, 9H), 1.98 (m, 1H), 2.90 (ddd, J = 4.4, 9.6, 9.6 Hz, 1H), 3.17 (s, 3H), 3.32 (ddd, J = 2.0, 10.0, 10.0 Hz, 1H), 3.35-3.39 (m, 1H), 3.44 (m, 1H), 3.57 (dd, J = 3.2, 9.6 Hz, 1H), 3.76 (dd, J = 2.8, 11.2 Hz, 1H), 3.92 (dd, J = 9.6, 11.2 Hz, 1H), 3.95-4.04 (m, 2H), 4.13 (ddd, J = 6.8, 6.8, 10.8 Hz, 1H), 4.22 (ddd, J = 6.8, 6.8, 10.4 Hz, 1H), 5.47 (dd, J = 2.4, 10.0 Hz, 1H), 5.52 (ddd, J = 3.2, 3.2, 9.6 Hz, 1H), 5.58 (dd, J = 2.0, 10.0 Hz, 1H), 7.33-7.39 (m, 6H), 7.64-7.69 (m, 4H); 13C NMR (125 MHz, CDCl3) δ 17.3, 19.4, 23.6, 23.9, 25.3, 26.0, 27.0, 27.5, 27.8, 32.1, 32.3, 33.9, 34.9, 36.0, 39.0, 39.2, 39.8, 42.0, 42.9, 56.2, 61.5, 62.7, 63.7, 64.5, 70.4, 71.9, 72.8, 74.1, 74.6, 74.7, 92.9, 100.1, 127.8, 128.5, 129.8, 133.8, 134.0, 136.0, 177.8, 178.7; IR (neat) cm-1 3454brs, 3048s, 2934s 2858m, 2363s, 2341s, 1661w, 1730s, 1284s, 1159s, 1106s, 1032s, 996s, 935s, 740s, 704s; mass spectrum (MALDI): m/z (% relative intensity) 957.5 (M+Na)+ (100); m/z calcd for C54H81O11SiNa+ 957.5519, found 957.5507. C9,11-Syn-Acetonide 47: Rf = 0.50 [20% EtOAc in hexane]; [α]D23 = + 2.54 [c 0.14, CH2Cl2]; 1H NMR (500 MHz, CDCl3) δ 0.81 (d, J = 7.0 Hz, 3H), 0.99 (s, 9H), 1.00 (s, 3H), 1.18 (s, 9H), 1.24 (s, 3H), 1.27 (s, 9H), 1.30-1.57 (m, 9H), 1.60-1.86 (m, 8H), 2.01 (m, 1H), 2.90 (ddd, J = 4.5, 10.5, 10.5 Hz, 1H), 3.20 (s, 3H), 3.33-3.42 (m, 2H), 3.37 (dd, J = 1.5, 10.0 Hz, 1H), 3.56 (dd, J = 3.0, 9.5 Hz, 1H), 3.77 (dd, J = 2.5, 10.5 Hz, 1H), 3.94 (m, 1H), 3.98 (dd, J = 10.0, 10.0 Hz, 1H), 4.09 (ddd, J = 2.0, 9.5, 9.5, Hz, 1H), 4.15 (m, 2H), 5.47 (dd, J = 2.0, 10.0 Hz, 1H), 5.58 (br-dd, J = 2.0, 10.0 Hz, 2H), 7.32-7.38 (m, 6H), 7.65-7.69 (m, 4H); 13C NMR (125 MHz, CDCl3) δ 17.2, 19.4, 20.2, 23.7, 23.9, 26.9, 27.5, 27.9, 30.4, 31.5, 31.9, 33.8, 35.2, 35.8, 38.2, 39.0, 39.3, 42.0, 43.2, 56.4, 61.5, 64.6, 64.7, 65.7, 69.1, 71.9, 72.5, 74.2, 74.5, 74.6, 92.9, 98.6, 127.8, 128.5, 129.8, 133.8, 134.2, 136.0, 177.0, 177.8; IR (neat) cm-1 3454brs, 3048s, 2934s 2858m, 2363s, 2341s, 1730s, 1284s, 1159s, 1106s, 1032s, 935s, 740s, 703s; mass spectrum (MALDI): m/z (% relative intensity) 957.6 (M+Na)+ (100); m/z calcd for C54H81O11SiNa+ 957.5519, found 957.5516.
ACKNOWLEDGEMENTS
We thank NSF [CHE1012198], American Chemical Society-Petroleum Research Foundation in the form of an AC-Grant. YT thanks Natural Science Foundation of China for generous funding [No. 21172169 and No. 21172168].
References
1. For isolations of spirastrellolide A, see: (a) D. E. Williams, M. Roberge, R. Van Soest, and R. J. Andersen, J. Am. Chem. Soc., 2003, 125, 5296; CrossRef (b) D. E. Williams, M. Lapawa, X. Feng, T. Tarling, M. Roberge, and R. J. Andersen, Org. Lett., 2004, 6, 2607; CrossRef for isolations of spirastrellolide B, see: (c) K. Warabi, D. E. Williams, B. O. Patrick, M. Roberge, and R. J. Andersen, J. Am. Chem. Soc., 2007, 129, 508; CrossRef for C-G, see: (d) D. E. Williams, R. A. Keyzers, K. Warabi, K. Desjardine, J. L. Riffell, M. Roberge, and R. J. Andersen, J. Org. Chem., 2007, 72, 9842; CrossRef also see: (e) M. Suzuki, R. Ueoka, K. Takada, S. Okada, S. Ohtsuka, Y. Ise, and S. Matsunaga, J. Nat. Prod., 2012, 75, 1192. CrossRef
2. M. Roberge, B. Cinel, H. J. Anderson, L. Lim, X. Jiang, L. Xu, C. M. Bigg, M. T. Kelly, and R. J. Andersen, Cancer. Res., 2000, 60, 5052.
3. For a leading reference, see: A. McCluskey, A. T. R. Sim, and J. A. Sakoff, J. Med. Chem., 2002, 45, 1151. CrossRef
4. (a) For a leading review, see: C. J. Oliver and S. Shenolikar, Frontiers in Bioscience, 1998, 3, 961; also see: (b) I. Paterson and E. A. Anderson, Science, 2005, 310, 451; CrossRef (c) F. E. Koehn and G. T. Carter, Nat. Rev. Drug Discovery, 2005, 4, 206; CrossRef (d) I. Paterson and K.-S. Yeung, Chem. Rev., 2005, 105, 4237; CrossRef (e) D. J. Newmann and G. M. Cragg, J. Nat. Prod., 2007, 70, 461; CrossRef (f) R. E. Honkanen and T. Golden, Curr. Med. Chem., 2002, 9, 2055; CrossRef (g) C. F. B. Holmes, J. T. Maynes, K. R. Perreault, J. F. Dawson, and M. N. G. James, Curr. Med. Chem., 2002, 9, 1981. CrossRef
5. For elegant synthetic efforts toward spirastrellolide A, see: (a) I. Paterson, E. A. Anderson, S. M. Dalby, and O. Loiseleur, Org. Lett., 2005, 7, 4121; CrossRef (b) I. Paterson, E. A. Anderson, S. M. Dalby, and O. Loiseleur, Org. Lett., 2005, 7, 4125; CrossRef (c) I. Paterson, E. A. Anderson, and S. M. Dalby, Synthesis, 2005, 3225; CrossRef (d) I. Paterson, E. A. Anderson, S. M. Dalby, J. H. Lim, P. Maltas, and C. Moessner, Chem. Commun. 2006, 4186; CrossRef (e) A. Fürstner, M. D. B. Fenster, B. Fasching, C. Godbout, and K. Radkowski, Angew. Chem. Int. Ed., 2006, 45, 5506; CrossRef (f) A. Fürstner, M. D. B. Fenster, B. Fasching, C. Godbout, and K. Radkowski, Angew. Chem. Int. Ed., 2006, 45, 5510; CrossRef (g) Y. Pan and J. K. De Brabander, Synlett, 2006, 853; CrossRef (h) C. Wang and C. J. Forsyth, Org. Lett., 2006, 8, 2997; CrossRef (i) I. Paterson, E. A. Anderson, S. M. Dalby, J. H. Lim, O. Loiseleur, P. Maltas, and C. Moessner, Pure Appl. Chem., 2007, 79, 667; CrossRef (j) A. B. Smith, III. and D.-S. Kim, Org. Lett., 2007, 9, 3311; CrossRef (k) I. Paterson, E. A. Anderson, S. M. Dalby, J. Genovino, J. H. Lim, and C. Moessner, Chem. Commun., 2007, 1852; CrossRef (l) A. Fürstner, B. Fasching, G. W. O’Neil, M. D. B. Fenster, C. Godbout, and J. Ceccon, Chem. Commun., 2007, 3045; CrossRef (m) K. A. Keaton and A. J. Phillip, Org. Lett. 2008, 10, 1083; CrossRef (n) S. Chandrasekhar, C. Rambabu, and A. S. Reddy, Org. Lett., 2008, 10, 4355; CrossRef (o) A. B. Smith, III, H. Smits, and D.-S. Kim, Tetrahedron, 2010, 66, 6597; CrossRef (p) G. Sabitha, A. S. Rao, and J. S. Yadav, Synthesis 2010, 505; CrossRef (q) J. L.-Y. Chen and M. A. Brimble, J. Org. Chem., 2011, 76, 9417. CrossRef
6. For the first total synthesis by Paterson, see: (a) I. Paterson, E. A. Anderson, S. M. Dalby, J. H. Lim, J. Genovino, P. Maltas, and C. Moessner, Angew. Chem. Int. Ed., 2008, 47, 3016; CrossRef (b) I. Paterson, E. A. Anderson, S. M. Dalby, J. H. Lim, J. Genovino, P. Maltas, and C. Moessner, Angew. Chem. Int. Ed., 2008, 47, 3021; CrossRef (c) I. Paterson, P. Maltas, S. M. Dalby, J. H. Lim, and E. A. Anderson, Angew. Chem. Int. Ed., 2012, 51, 2749; CrossRef for a review and highlight, see: (d) M. V. Perkins, Angew. Chem. Int. Ed., 2008, 47, 2921; CrossRef (e) I. Paterson and S. M. Dalby, Nat. Prod. Rep., 2009, 26, 865. CrossRef
7. For cyclic acetal tethered cycloadditions, see: (a) S. K. Ghosh, R. P. Hsung, and J. Liu, J. Am. Chem. Soc., 2005, 127, 8260; CrossRef (b) J. Wang, R. P. Hsung, and S. K. Ghosh, Org. Lett., 2004, 6, 1939; CrossRef (c) S. K. Ghosh, Y. Wei, A. I. Gerasyuto, J. B. Feltenberger, J. Wang, and R. P. Hsung, Heterocycles, 2010, 82, 1379; CrossRef For cyclic acetal tethered RCM, see: (d) S. K. Ghosh, R. P. Hsung, and J. Wang, Tetrahedron Lett., 2004, 45, 5505; CrossRef (e) S. K. Ghosh, C. Ko, J. Liu, J. Wang, and R. P. Hsung, Tetrahedron, 2006, 62, 10485; CrossRef (f) R. Figueroa, R. P. Hsung, and C. C. Guevarra, Org. Lett., 2007, 9, 4857; CrossRef (g) R. Figueroa, J. B. Feltenberger, C. C. Guevarra, and R. P. Hsung, Science: China Chem., 2011, 54, 31. CrossRef
8. For elegant studies in the area of cyclic acetal-tethered RCM, see: (a) P. A. V. van Hooft, M. A. Leeuwenburgh, H. A. Overkleeft, G. A. van der Marel, C. A. A. van Boeckel, and J. H. van Boom, Tetrahedron Lett., 1998, 39, 6061; CrossRef (b) M. A. Leeuwenburgh, C. C. M. Appeldoorn, P. A. V. van Hooft, H. A. Overkleeft, G. A. van der Marel, and J. H. van Boom, Eur. J. Org. Chem., 2000, 873; CrossRef (c) M. J. Bassindale, P. Hamley, A. Leitner, and J. P. A. Harrity, Tetrahedron Lett., 1999, 40, 3247; CrossRef (d) E. C. Hansen, and D. Lee, J. Am. Chem. Soc., 2004, 126, 15074; CrossRef (e) M. Lejkowski, P. Banerjee, J. Runsink, and H. J. Gais, Org. Lett., 2008, 10, 2713; CrossRef also see: (f) E. C. Hansen and D. Lee, J. Am. Chem. Soc., 2003, 125, 9582; CrossRef (g) S. S. Kinderman, R. Doodeman, J. W. van Beijma, J. C. Russcher, K. C. M. F. Tjen, T. M. Kooistra, H. Mohaselzadeh, J. H. van Maarseveen, H. Hiemstra, H. E. Schoemaker and F. P. J. T. Rutjes, Adv. Syn. Catal., 2002, 344, 736; CrossRef (h) V. A. Keller, J. R. Martinellie, E. R. Strieter, and S. D. Burke, Org. Lett., 2002, 4, 467; CrossRef (i) E. A. Voight, C. Rein, and S. D. Burke, J. Org. Chem. 2002, 67, 8489; CrossRef (j) S. D. Burke and E. A. Voight, Org. Lett., 2001, 3, 237; CrossRef (k) S. D. Burke, N. Muller, and C. M. Beaudry, Org. Lett., 1999, 1, 1827; CrossRef (l) M. Scholl and R. H. Grubbs, Tetrahedron Lett., 1999, 40, 1425. CrossRef
9. For a review on chemistry of spiroketals, see: (a) J. E. Aho, P. M. Pihko, and T. K. Rissa, Chem. Rev., 2005, 105, 4406; CrossRef (b) K. T. Mead and B. N. Brewer, Curr. Org. Chem., 2003, 7, 227; CrossRef (c) M. A. Brimble and F. A. Fares, Tetrahedron, 1999, 55, 7661; CrossRef (d) M. T. Fletcher and W. Kitching, Chem. Rev., 1995, 95, 789; CrossRef (e) F. Perron and K. F. Albizati, Chem. Rev., 1989, 89, 1617. CrossRef
10. While this strategy was documented in Fürstner’s approach [reference 5e], we first unveiled our analysis in: (a) J. Liu, J.-H. Yang, C. Ko, and R. P. Hsung, Tetrahedron Lett., 2006, 47, 6121; CrossRef (b) J. Liu, Ph.D. Dissertation Thesis: University of Minnesota, 2006.
11. (a) P. K. Jadhav, K. S. Bhat, P. T. Perumal, and H. C. Brown, J. Org. Chem., 1986, 51, 432; CrossRef (b) J. R. Parikh and W. von E. Doering, J. Am. Chem. Soc., 1967, 89, 5505; CrossRef (c) J. A. Dale and H. S. Mosher, J. Am. Chem. Soc., 1973, 95, 512; CrossRef (d) I. Ohtani, T, Kusumi, Y. Kashman, and H. Kakisawa, J. Am. Chem. Soc., 1991, 113, 4092. CrossRef
12. H. Fuwa, N. Ichinokawa, K. Noto, and M. Sasaki, J. Org. Chem., 2012, 77, 2588. CrossRef
13. (a) J. Yang, J. Liu, and R. P. Hsung, Org. Lett., 2008, 10, 2525; CrossRef (b) J. Liu and R. P. Hsung, Org. Lett., 2005, 7, 2273. CrossRef
14. Lewis acids screened: BF3-OEt2, TiCl4, TMSOTf, In(OTf)3, and MgBr2. Brønsted acids screened: Tf2NH, p-TsOH, TFA, and K-10. Temp: –20 °C to rt.
15. For studies on the acidity of HNTf2, see: (a) C. Thomazeau, H. Olivier-Bourbigou, L. Magna, S. Luts, and B. Gilbert, J. Am. Chem. Soc., 2003, 125, 5264, and references cited therein; CrossRef (b) J. Foropoulus and D. D. DesMarteau, Inorg. Chem., 1984, 23, 3720. CrossRef
16. For some examples, see: (a) A. Sakakura, K. Suzuki, K. Nakano, and K. Ishihara, Org. Lett., 2006, 8, 2229; CrossRef (b) J. Sun and S. A. Kozmin, J. Am. Chem. Soc., 2005, 127, 13512; CrossRef (c) K. Inanaga, K. Takasu, and M. Ihara, J. Am. Chem. Soc., 2005, 127, 3668; CrossRef (d) L. Zhang and S. A. Kozmin, J. Am. Chem. Soc., 2004, 126, 10204; CrossRef (e) J. Cossy, F. Lutz, V. Alauze, and C. Meyer, Synlett, 2002, 45; CrossRef (f) K. Ishihara, Y. Hiraiwa, and H. Yamamoto, Synlett, 2001, 1851; CrossRef (g) N. Kuhnert, J. Peverley, and J. Roberston, Tetrahedron Lett., 1998, 39, 3215. CrossRef
17. C. Ko and R. P. Hsung, Org. Biomol. Chem., 2007, 5, 431. CrossRef
18. (a) B. O. Lindgren and T. Nilsson, Acta. Chem. Scand., 1973, 27, 888; CrossRef (b) B. S. Bal, W. E. Childers, Jr., and H. A. Pinnick, Tetrahedron, 1981, 37, 2091. CrossRef
19. For syntheses of 24 and 33a, see: (a) A. Zampella, V. Sepe, R. D'Orsi, G. Bifulco, C. Bassarello, and M. V. D'Auria, Tetrahedron: Asymmetry, 2003, 14, 1787; CrossRef (b) H. C. Brown and K. S. Bhat, J. Am. Chem. Soc., 1986, 108, 5919; CrossRef for the synthesis of key precursor to 33b, see: (c) K. E. Drouet and E. A. Theodorakis, Chem. Eur. J., 2000, 6, 1987. CrossRef
20. For leading reviews on the anomeric effect related to carbohydrate chemistry, see: (a) M. H. D. Postema, C-Glycoside Synthesis; CRC Press: Ann Arbor, MI, 1995; (b) M. H. D. Postema, C-Glycoside Synthesis, CRC Press: Ann Arbor, 1995; (c) K. A. Parker, Pure Appl. Chem., 1994, 66, 2135; CrossRef (d) D. E. Levy and C. Tang, The Chemistry of C-Glycosides. 1st ed.; Pergamon Press: 1995, Vol. 13; also see: (e) R. J. Woods, C. W. Andrews, and J. P. Bowen, J. Am. Chem. Soc., 1992, 114, 859; CrossRef (f) M. Miljkovic, D. Yeagley, P. Deslongchamps, and Y. L. Dory, J. Org. Chem., 1997, 62, 7597. CrossRef
21. For reviews, see: (a) R. H. Grubbs, S. J. Miller, and G. C. Fu, Acc. Chem. Res., 1995, 28, 446; CrossRef (b) R. R. Schrock, Tetrahedron, 1999, 55, 8141; CrossRef (c) M. Mori, in Topics in Organometallic Chemistry; A. Fürstner, ed.; Springer-Verlag: Berlin, Heidelberg, 1998, 1, 133; (d) A. Fürstner, Angew. Chem. Int. Ed., 2000, 39, 3012; CrossRef (e) T. M. Trnka and R. H. Grubbs, Acc. Chem. Res., 2001, 34, 18. CrossRef
22. T. Mukaiyama, K. Banno, and K. Naraska, J. Am. Chem. Soc., 1974, 96, 7503. CrossRef
23. (a) D. A. Evans, M. J. Dart, J. L. Duffy, M. G. Yang, and A. B. Livingston, J. Am. Chem. Soc., 1995, 117, 6619; CrossRef (b) D. A. Evans, M. J. Dart, J. L. Duffy, and M. G. Yang, J. Am. Chem. Soc., 1996, 118, 4322; CrossRef (c) D. A. Evans, J. D. Duffy, and M. J. Dart, Tetrahedron Lett., 1994, 35, 8537. CrossRef
24. (a) D. A. Evans, J. Bartroli, and T. L. Shih, J. Am. Chem. Soc., 1981, 103, 2127; CrossRef b) D. A. Evans, E. Vogel, and J. V. Nelson, J. Am. Chem. Soc., 1979, 101, 6120. CrossRef
25. J.-H. Yang, Ph.D. Dissertation Thesis: University of Wisconsin, 2009.
26. (a) S. D. Rychnovsky, B. Rogers, and G. Yang, J. Org. Chem., 1993, 58, 3511; CrossRef (b) S. D. Rychnovsky and D. J. Skalitzky, Tetrahedron Lett., 1990, 31, 945. CrossRef
27. D. A. Evans, D. L. Rieger, and J. R. Gage, Tetrahedron Lett., 1990, 31, 7099. CrossRef