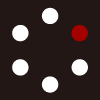
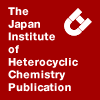
HETEROCYCLES
An International Journal for Reviews and Communications in Heterocyclic ChemistryWeb Edition ISSN: 1881-0942
Published online by The Japan Institute of Heterocyclic Chemistry
e-Journal
Full Text HTML
Received, 8th November, 2012, Accepted, 13th December, 2012, Published online, 25th December, 2012.
DOI: 10.3987/REV-12-759
■ Synthesis of Alkaloids Using Radical Cyclizations
Tsuyoshi Taniguchi and Hiroyuki Ishibashi*
School of Pharmaceutical Sciences, Institute of Medical, Pharmaceutical and Health Sciences, Kanazawa University, Kakuma, Kanazawa, Ishikawa 920-1192, Japan
Abstract
This review focuses on radical cyclizations for the synthesis of a variety of alkaloids. Emphasis has been placed on radical cascades that afford synthesis of (−)-cephalotaxine and (±)-stemonamide.WHAT ARE RADICALS?
Radicals are highly reactive atoms, molecules, or ions bearing an unpaired electron and are important in fields of organic chemistry including polymer chemistry, material chemistry and electrochemistry as well as life science. Radical chemistry has been an accepted area of research for almost 110 years and its chemistry dates back to 1900, when Gomberg investigated the formation and reactions of the triphenylmethyl radical.
Radical cyclizations are reactions of a radical with one π−orbital of an unsaturated bond such as C-C double bond and serve for the construction of cyclic compounds.1 Two types, exo and endo modes, exist for radical cyclizations. The most popular one is the 5-exo-trig mode of cyclization (Scheme 1).
In the course of our studies on olefin cyclizations of sulfur-substituted carbocations, we found that the cation 2, generated formally from 1, underwent olefin cyclization to give products via cyclized intermediate 32 but that the compound 4 by treated with the same Lewis acid (SnCl4) gave no cyclized compound. In contrast, when compound 4 was treated with Bu3SnH in the presence of AIBN, it underwent cyclization smoothly to give product 5 (Scheme 2).3
These types of carbamoylmethyl radicals, generated from the corresponding α-halogenated acetamides, had not been described in the literature and the only reported examples were Pd-catalyzed cyclizations of α−haloacetamides.4 Reactions of 6 and 8 with Bu3SnH/AIBN are summarized in Scheme 3.5
The results indicate that methylthio-substituted radicals 7a-c (R = Me, CH2Ph or Ph) preferentially gave cyclization products, whereas the radical 9 gave only a small quantity of the cyclization product 10. Treatment of 9 under the cyclization conditions preferentially gave the reduction product (compare 7a and 9). These results are somewhat surprising considering the general observation of so-called ‘stabilized’ radicals being less reactive than ‘non-stabilized’ radicals towards C-C bond-forming reactions with unsaturated bonds,6 since radicals 7a-c are stabilized by a captodative effect.7 The next matter is also intriguing. Treatment of 6d with Bu3SnH gave only the reduction product instead of the cyclization product. This is probably a similar reason for the difficulty in obtaining γ-lactone using radical cyclization from a compound in which NH is substituted with oxygen (O).8
A variety of alkaloids, mesembranol,9 pretazettine,10 pancracine,11 physostigmine,12 chilenine,13 mappicine ketone,14 ipalbidine,15 aphanorphine,16 and tetrahydropalmatine,17 were synthesized using 5-exo-trig and 6-exo-trig cyclizations of a carbamoylmethyl radical and aryl radical (Scheme 4).
5-ENDO-TRIG AND 4-EXO-TRIG CYCLIZATIONS
Bn3SnH-Mediated radical cyclization of 11 gave bicyclic γ-lactam 13. Compound 12 alsogave γ-lactam 13.18 Formation of 13 from 11 was a 5-exo-trig radical cyclization, which is very easy to undergo. The latter was a 5-endo-trig type cyclization, which is recognized to be very difficult to undergo (Scheme 5).19
5-Endo-trig cyclization was useful for the synthesis of β-non-substituted γ-lactams, and the synthesis of (±)-cotinine (14) is illustrated in Scheme 6.20
In order to examine the effect of the carbonyl group in the enamide 12 for the success of 5-endo-trig cyclization, we examined the reaction of enamide 15 (Scheme 7). Treatment of 15 with Bu3SnH in the presence of AIBN gave the 5-endo-trig cyclization product 16a,b and the reduction product. However, the positional isomer 17 gave only the reduction product 18 when treated with Bu3SnH and AIBN. No 5-endo-trig cyclization product was isolated. Thus, it was shown that the carbonyl group incorporated into the five-membered ring is essential for effecting the 5-endo-trig cyclization, though the exact role of the carbonyl group is obscure at present.
During the study on 5-endo-trig cyclizations of carbamoylmethyl radicals, we found that the cyclization of enamide 20 afforded the tricyclic β-lactam 21 (Scheme 8).18 No γ-lactam was detected in the reaction mixture. The difference in the mode of cyclization between enamides 12 and 20 may be explained in terms of the electronic stability between the radical intermediates 19 and 23, generated by a ring closure of the corresponding carbamoylmethyl radical. The exclusive formation of the β-lactam 21 from 20 suggests that the benzylic radical intermediate 23 might be more stable than the α−acylamino radical 22 which affords γ-lactam.
Inspection of molecular models indicates that the radical intermediate 23 should be stabilized due to excellent overlapping of the p-orbital of the radical center with the neighboring aromatic π-system.18 In sharp contrast to the case of 20, which gave only the β-lactam 21, enamide 24 provided the γ-lactam 25. No β-lactam-like compound was isolated. Molecular models indicate that the p-orbital of the seven-membered benzylic radical 27 is almost perpendicular to the neighboring aromatic π-system in its most conformationally stable form, so that the α-acylamino radical 26 was relatively stable in preference to the benzylic radical 27, leading to the observed γ-lactam 25.18
Synthesis of monobactam was then examined. We found that the phenyl group alone was insufficient to stabilize the radical intermediate such as 23 (Scheme 9) formed by 4-exo-trig cyclization of bromide 28.21 The methyl or phenylthio group (see 29 or 30) as an additional radical stabilizing group gave exclusively β-lactam.22 The phenylthio group alone provided β-lactam.23 Preparation of synthetic intermediate (31) for (±)-PS-5 is illustrated in Scheme 10.23
ARYL RADICAL CYCLIZATION ONTO ENAMIDE OLEFINS: 6-ENDO-SELEVTIVE CYCLIZATIONS
A 5-exo-trig cyclization is generally preferred over a 6-endo-trig ring closure in systems having an alkenic bond at the 5-position relative to the aryl radical center. For example, aryl bromides 32 (X = CH2, O, NCOR) (Scheme 11), upon treatment with Bu3SnH/AIBN, gave almost exclusively the 5-exo cyclization products 33. This is also the case for the cyclization of enamide 34, which gave only the five-membered lactam 36 via the radical intermediate 35 by 5-exo cyclization, though the reaction is expected to give more stable α−acylamino radical 37 by 6-endo cyclization. However, we found that the bromide 38 underwent an aryl radical cyclization in a 6-endo-trig manner to give exclusively six-membered lactam 39.24 The possibility of a consecutive 5-exo cyclization and neophyl-like rearrangement of the resulting radical 40 could be ruled out by the effect of various Bu3SnH concentrations addition times and reaction temperatures.25
The most plausible explanation for the results with 34 and 38 may be derived from consideration of the rotation of the enamide. In the conformers 41 (for 34) and 38 (for 42), severe steric repulsions between acyl and C=C groups, respectively, are evident (Scheme 12). The conformers 34 and 42, therefore, predominate, and the resulting radicals attack on the more proximate Cα-position of 34 and Cβ-position of 42 to give the observed 5-exo cyclization product 36 and the 6-endo cyclization product 39, respectively. NOE difference spectroscopy also indicated that compound 38 exists only in the conformer 42.
7-ENDO-SELECTIVE CYCLIZATIONS
We next examined the modes of cyclization of compounds 44 and 47 (Scheme 13). When enamide 44 was treated with Bu3SnH in the presence of ACN (1,1’-azobiscyclohexanecarbonitrile), a 3:1 mixture of 45 and 46 was obtained. Formation of 45 and 46 may be explained simply by 6-exo-trig and 7-endo-trig cyclizations of the aryl radical formed from 44, respectively. On the other hand, a similar cyclization of enamide 47 gave the seven-membered compound 48. An exo mode of cyclization also could be shifted to an endo mode by a positional change of the carbonyl group of enamide.
RADICAL CASCADE
A radical generated by the first radical cyclization can cause the second cyclization with another radical acceptor in the same molecule. This consecutive radical cyclization is called “radical cascade” or “tandem radical cyclization”. This reaction serves as a method for construction of polycyclic systems of natural products.
6-ENDO-TRIG/5-ENDO-TRIG RADICAL CASCADE
Synthesis of 13a-Methylphenanthroindolizidines.
When compound 49 (Scheme 14) was treated with Bu3SnH in the presence of ACN, the tricyclic compound 51 was obtained. Formation of 51 was rationalized in terms of 6-endo-trig cyclization of the aryl radical formed from 49 followed by 5-endo-trig cyclization of the resultant intermediacy of α-amidoyl radical 50. The present method was applied to the synthesis of 13a-methylphenanthroindolizidine alkaloids such as 55 from 52 (Scheme 15).26 It should be noted that 5-endo-trig cyclization did not occur in the absence of the carbonyl group in 50 or 53.
Synthesis of Cylindricine Skeletons
Compound 56, on treatment with Bu3SnH/ACN, afforded tricyclic compound 58 via α-amidoyl radical 57 (Scheme 16).27 The structure of 58 was unequivocally established by X-ray crystallographic analysis. Compound 58 is a basic structural element of the anti-cancer agent cylindricine A (59).
7-ENDO-TRIG/5-ENDO-TRIG RADICAL CASCADE
Synthesis of (−)-Cephalotaxine
Cephalotaxus alkaloids are a class of cytotoxic natural products first isolated from Asian plum yews Cephalotaxus drupacea and Cephalotaxus fortunei. (―)-Cephalotaxine (60) has attracted much attention due to its fascinating pentacyclic structure and the antileukemic activity of its ester derivatives such as homoharringtonine (61) (Scheme 17). Since the first total synthesis of (±)-cephalotaxine by Weinreb and Semmelhack, a number of approaches to the synthesis of (―)-cephalotaxine (60) have been reported and several efforts have culminated in a total synthesis of a racemic mixture of or optically active cephalotaxine. One of the most frequently used strategies is C4-C13 bond formation, i.e., formation of the C-ring of the cephalotaxine structure.
When compound 62 was treated with Bu3SnH/ACN in boiling toluene, a cephalotaxine skeleton 64 was obtained via a α-amidoyl radical 63 (Scheme 18).28 Although the yield of 64 was unsatisfactory, the feature of the cyclization was a simultaneous construction the C ring of the cephalotaxine skeleton followed by the D ring.
We then applied this method to the synthesis of (-)-cephalotaxine (77) (60) (Scheme 20). Scheme 19 shows the synthesis of optically active cyclopentanone 70. Triflate 66 was prepared from diethyl D-tartrate via tosylate 65, and the following reaction with lithium phenylacetylide gave acetylene 67.
Bromination of 67 with lithium bromide followed by removal of acetonide afforded diol 68. Then diol 68 underwent Bu3SnH-mediated radical cyclization to give five-membered product 69. Protection of 69 with TBDPSCl followed by ozonolysis afforded the desired cyclopentanone 70 (Scheme 19).
We next examined the synthesis of a radical of precursor and its radical cascade (Scheme 20). Condensation of amine 71 with cyclopentanone 70 in the presence of Ti(OiPr)4 afforded the corresponding imine, which was acylated with acryloyl chloride to give enamide 72. On treatment with Bu3SnH in the presence of ACN in boiling chlorobenzene, a radical cascade of enamide 72 occurred to give desired pentacyclic compound 73. The 1H NMR spectrum of 73 showed it to be a single isomer having a stereochemistry as depicted in Scheme 20. Removal of the two TBDPS groups with TBAF gave 74. Treatment of 74 with DMSO in the presence of TFAA afforded oxidized compound 75. By using the known procedure, ketone 75 was treated with methoxytrimethylsilane to give methoxy ketone 76. Compound 76 was reduced with alane to give (−)-cephalotaxine (60),29 [α]D –185 (c = 0.175, CHCl3) [lit,30 [α]D –182 (c = 0.21, CHCl3)].
Synthesis of Stemonamide and Isostemonamide
The Stemona alkaloids (–)-stemonamide (77) and (–)-isostemonamide (78) and their reduced compounds, (±)-stemonamine (79) and (±)-isostemonamine (80) (Scheme 21), were isolated from the roots of Stemona japonica, which have been used in Chinese and Japanese folk medicines as cough medicines and insecticides. Their tetracyclic structure including contiguous spirocyclic quaternary centers provides attractive target molecules for total synthesis.
The above radical cascade involving a 7-endo-trig selective cyclization of an alkyl radical onto an olefinic bond of enamides could be applied to the radical cascade of an alkyl radical derived from 81 (Scheme 22). Treatment of 81 with Bu3SnH/ACN gave a 1:1 mixture of radical cascade products 83 and 84 via the α–amidoyl radical 82 (Scheme 22).
The mixture of compounds 83 and 84 was then subjected to an aldol reaction with benzaldehyde to give an inseparable mixture of α,β-unsaturated ketones 85a,b (Scheme 23). A subsequent addition reaction of 85a,b with lithium ethyl propiolate afforded the adducts 86 and 87 in 50% and 48% isolated yields, respectively. X-Ray crystallographic analysis of 86 and 87 confirmed their structures, indicating that the phenyl groups of the mixture 85a,b have stereochemistries as depicted in Scheme 23. Formation of 86 and 87 might be a result of an attack of lithium ethyl propiolate on the convex faces of 85a and 85b, respectively.
Treatment of 86 with magnesium methoxide in boiling MeOH afforded methyl tetronate (β-methoxy α,β-unsaturated lactone) 88 (Scheme 24). α-Methylation of the α,β-unsaturated bond of 88 with LDA/methyl iodide failed. Hence, an alternative method of α-methylation was examined. Iodination of 88 with N-iodosuccinimide (NIS) in the presence of trifluoromethanesulfonic acid (TfOH) gave iodide 89. Treatment of compound 89 with trimethylboroxine in the presence of PdCl2(dppf)2 (Suzuki-Miyaura coupling) afforded methylated compound 90 in high yield.
Similarly, iodination of compound 91, prepared from 87 with magnesium methoxide, by bis(trimethylpyridine)iodonium hexafluorophosphate in the presence of TfOH gave 92 Iodination NIS/TfOH gave an unsatisfactory result. Suzuki-Miyaura coupling of 92 with trimethylboroxine afforded compound 93 (Scheme 25).
Oxidative cleavage of alkene 91 with OsO4/NaIO4 gave ketone 94. α-Methylenation of ketone 94 with Eschenmoser's salt in the presence of various bases such as KH and LDA afforded unsaturated ketone 95, but the yield was low. Similar α-methylenation using paraformaldehyde/N-methylanilinium trifluoroacetate also gave an unsatisfactory result. We therefore examined another route to 96. Treatment of ketone 94 with tert-butoxybis(dimethylamino)methane (Bredereck’s reagent) gave enaminone 95, whose reduction with DIBAL followed by methylation with MeI afforded 96. Similarly, compound 93 was converted to α -methylenated ketone 100 (Scheme 26).
Finally, RhCl3-mediated isomerization of the double bond of exo-methylene ketone 96 gave (±)-stemonamide (77) (mp 232-233 °C, lit.31 mp 240-241 °C) along with 100 in 31% and 63% yields, respectively (Scheme 27).32 1H NMR spectra of the unexpected compound 100 showed it to be a single stereoisomer. It is presumed that an attack of RhCl3 on the β-face of 9-H of 95 brings about isomerization of the double bond to give (±)-77, whereas when RhCl3 attacks the opposite side (α-face) of 9-H, reduction of the double bond with RhCl3 takes place to give 100. Therefore, the methyl group of 100 seemed to be a β-orientation.
On the other hand, RhCl3 attacked on the β-face of 9-H of 99 to afford (±)-isostemonamide (78) (mp 223-224 °C, lit.31 mp 225-227 °C) quantitatively.32
Synthesis of (±)-Stemonamine and (±)-Isostemonamine
(±)-Stemonamine (79) and (±)-Isostemonamine (80) can easily be interconverted to each other and, hence (±)-stemonamine (79) was obtained by reduction of thioamide 101, prepared from stemonamide (77), at low temperatures (0 °C to room temperature) in EtOH with Raney Ni (Scheme 28).32b
Similarly, (±)-isostemonamine (80) was obtained by reduction of thioamide 102, prepared from isostemonamide (78).
If the reduction of thioamide 101, prepared from stemonamide (77), was carried out in refluxing EtOH, a mixture of (±)-stemonamine (79) and (±)-isostemonamine (80) was obtained in 56% and 40% yields, respectively.
CONCLUSIONS
We found that carbamoylmethyl radicals generated from the corresponding α-chloro(bromo)acetamides work well as reactive species to give various alkaloids and that an exo mode of cyclization onto olefins of enamides can be shifted to an endo mode by a positional change of their carbonyl group of enamides and disclosed the new synthesis of several alkaloids including (−)-cephalotaxine using a radical cascade initiated by endo-selective cyclization. Further studies directed toward the synthesis of other alkaloids using this strategy are now in progress in our laboratory.
ACKNOWLEDGEMENTS
This work was supported by a Grant-in-Aid for Scientific Research from the Ministry of Education, Culture, Sports, Science and Technology of Japan.
References
1. B. Giese, “Radicals in Organic Synthesis: Formation of Carbon-Carbon Bond,” Pergamon Press, Oxford, 1986.
2. H. Ishibashi, M. Ikeda, H. Maeda, K. Ishiyama, M. Yoshida, S. Aksai, and Y. Tamura, J. Chem. Soc., Perkin Trans. 1, 1987, 1099. CrossRef
3. H. Ishibashi, T. Sato, M. Irie, S. Harada, and M. Ikeda, Chem. Lett., 1987, 795. CrossRef
4. M. Mori, N. Kanda, I. Oda, and Y. Ban, Tetrahedron, 1985, 41, 5465. CrossRef
5. T. Sato, Y. Wada, M. Nishimoto, H. Ishibashi, and M. Ikeda, J. Chem. Soc., Perkin Trans. 1, 1989, 879. CrossRef
6. Y. Ueno, R. K. Khare, and M. Okawara, J. Chem. Soc., Perkin Trans. 1, 1983, 2637. CrossRef
7. H. G. Viehe, D.-I. R. Merenyi, L. Stella, and Z. Janousel, Angew. Chem., Int. Ed. Engl., 1979, 18, 917. CrossRef
8. D. P. Curran and C.-T. Chang, J. Org. Chem., 1989, 54, 3140. CrossRef
9. H. Ishibashi, T. S. So, K. Okochi, T. Sato, N. Nakamura, H. Nakatani, and M. Ikeda, J. Org. Chem., 1991, 56, 95. CrossRef
10. H. Ishibashi, N. Uemura, H. Nakatani, M. Okazaki, T. Sato, N. Nakamura, and M. Ikeda, J. Org. Chem., 1993, 58, 2360. CrossRef
11. M. Ikeda, M.Hamada, T. Yamashita, K. Matsui, T. Sato, and H. Ishibashi, J. Chem. Soc., Perkin Trans. 1, 1999, 1949. CrossRef
12. H. Ishibashi, T. Kobayashi, N. Machida, and O. Tamura, Tetrahedron, 2000, 56, 1469. CrossRef
13. H. Ishibashi, H. Kawanami, H. Iriyama, and M. Ikeda, Tetrahedron Lett., 1995, 36, 6733. CrossRef
14. I. Kato, M. Higashimoto, O. Tamura, and H. Ishibashi, J. Org. Chem., 2003, 68, 7983. CrossRef
15. M. Ikeda, J. Shikaura, N. Maekawa, K. Daibuzonoi, H. Teranishi, Y. Teraoka, N. Oda, and H. Ishibashi, Heterocycles, 1999, 50, 31. CrossRef
16. O. Tamura, T. Yanagimachi, and H. Ishibashi, Tetrahedron: Asymmetry, 2003, 14, 3033. CrossRef
17. H. Ishibashi, H. Kawanami, H. Nakagawa, and M. Ikeda, J. Chem. Soc., Perkin Trans. 1, 1997, 2291. CrossRef
18. J. E. Baldwin, J. Cutting, W. Dupont, L. Kruse, L. Silberman, and R. C. Thomas, J. Chem. Soc., Chem. Comm., 1976, 736.
19. T. Sato, N. Nakamura, K. Ikeda, M. Okada, H. Ishibashi, and M. Ikeda, J. Chem. Soc., Perkin Trans. 1, 1992, 2399. CrossRef
20. T. Sato, N. Chono, H. Ishibashi, and M. Ikeda, J. Chem. Soc., Perkin Trans. 1, 1995, 1115. CrossRef
21. H. Ishibashi, K. Kodama, C. Kameoka, H. Kawanami, and M. Ikeda, Tetrahedron, 1996, 52, 13867. CrossRef
22. H. Ishibashi, C. Kameoka, K. Kodama, H. Kawanami, M. Hamada, and M. Ikeda, Tetrahedron, 1997, 53, 9611. CrossRef
23. H. Ishibashi, C. Kameoka, H. Iriyama, K. Kodama, T. Sato, and M. Ikeda, J. Org. Chem., 1995, 60, 1276. CrossRef
24. H. Ishibashi, I. Kato, Y. Takeda, M. Kogure, and O. Tamura, Chem. Comm., 2000, 1527. CrossRef
25. K. A. Parker, D. M. Spero, and K. C. Inman, Tetrahedron Lett., 1986, 27, 2833. CrossRef
26. K. Takeuchi, A. Ishita, J. Matsuo, and H. Ishibashi, Tetrahedron, 2007, 63, 11101. CrossRef
27. T. Taniguchi, O. Tamura, M. Uchiyama, O. Muraoka, G. Tanabe, and H. Ishibashi, Synlett, 2005, 1179. CrossRef
28. T. Taniguchi, A. Ishita, M. Uchiyama, O. Tamura, O. Muraoka, G. Tanabe, and H. Ishibashi, J. Org. Chem., 2005, 70, 1922. CrossRef
29. T. Taniguchi and H. Ishibashi, Org. Lett., 2008, 10, 4129. CrossRef
30. L. Planas, J. Pérard-Viret, and J. Royer, J. Org. Chem., 2004, 69, 3087. CrossRef
31. A. S. Kende, J. I. Martin Hernando, and J. B. J. Milbank, Tetrahedron, 2002, 58, 61. CrossRef
32. a) T. Taniguchi, O. Muraoka, G. Tanabe, and H. Ishibashi, Org. Lett., 2008, 10, 4129; CrossRef b) T. Taniguchi and H. Ishibashi, Tetrahedron, 2008, 64, 8773. CrossRef