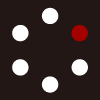
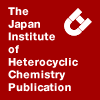
HETEROCYCLES
An International Journal for Reviews and Communications in Heterocyclic ChemistryWeb Edition ISSN: 1881-0942
Published online by The Japan Institute of Heterocyclic Chemistry
e-Journal
Full Text HTML
Received, 6th October, 2012, Accepted, 31st October, 2012, Published online, 7th November, 2012.
DOI: 10.3987/REV-12-756
■ Chemical Pharma-Sciences that Incorporate Non-Covalent Bonded Interactions
Yoshimitsu Nagao*
Graduate School of Pharmaceutical Sciences, University of Tokushima, Sho-machi, Tokushima 770-8505, Japan
Abstract
Molecular structure characteristics of organosulfur drugs and biologically active organosulfur compounds and the asymmetric Pummerer-type reactions are discussed in terms of non-covalent bonded S···X (X = O, N, S, halogens, etc.) interactions based on the data of X-ray crystallographic analysis, density functional theory (DFT) calculation, some spectroscopic analyses, and cold-spray ionization mass spectrometric (CSI-MS) analysis.CONTENTS
1. Introduction
2. Thiadiazoline and related compounds
3. β-Lactam antibiotics
4. Thiazole and related compounds
5. Sulfoxides and sulfones
6. Disulfide and related compounds
7. Various considerations on the non-covalent bonded interactions
8. Conclusion
1. INTRODUCTION
The author and his group have been conducting both basic and applied research in the pharmaceutical sciences based on the molecular structure characteristics involving non-covalent bonded interactions,1 prochiral σ-symmetry, dipole-dipole repulsion, active amide structures, etc., as shown in Figure 1.2-4 With respect to basic research, we have pursued the development of new reactions and establishment of new concepts by employing our own reagents and characteristic substrates.2-4 In terms of applied research related to the basic research described above, we have been pursuing the development of new lead and seed compounds that could lead to new drugs and have performed research on new pharmacophores by using chemical and pharmaceutical evaluations of the drug molecules used currently and molecular design of new drugs. Thus, we have used the term “chemical pharma-sciences” to describe our chemistry-based research in the pharmaceutical sciences, as shown in Figure 1.2-4 Here I would like to review mainly our research in terms of non-covalent bonded interactions.1
A number of organosulfur compounds have been found in heterocyclic natural products5 and exploited as medicines, dietary supplements, and agricultural chemicals.6 In fact, there are approximately 275 organosulfur drugs among the total 1130 drugs used currently at the hospitals and clinical pharmacies in Japan.7
Intramolecular non-covalent bonded interactions including hypervalent bond interactions between sulfur and oxygen (nitrogen, sulfur) atoms have been observed in a large number of organosulfur compounds.8 In these molecules, the distances between the non-covalent bonded S···O, S···N, and S···S atoms are significantly shorter than the sum (3.32, 3.35, and 3.60 Å) of the corresponding van der Waals radii in the crystalline structures.9 These kinds of interactions can also be classified into several categories such as 1-3, 1-4, 1-5, and 1-6 types according to the size of the quasi-ring involving S···X (X = O, N, S, halogens, and other heteroatoms) moieties, as shown in Figure 2.8h With respect to the biological significance of the intramolecular non-covalent bonded S···O attraction, the pioneering studies were performed by Goldstein and colleagues (vide infra).6a,h,8m,10 Our recent efforts have been focused on the establishment and exploitation of various non-covalent bonded interactions (attraction or repulsion) such as intra- and
intermolecular non-covalent bonded S···X (X = O, N, S, halogens, π, etc.) attraction, Zn2+···X complex (X = O, N, S, halogens) attraction, CH···O hydrogen bonding, CH···π attraction, π···π attraction, and dipole(C=O)-dipole(C=S) repulsion in the development of new reactions and new lead compounds with the ultimate goal of new drug discovery.2-4,6p,q,t-v,8u,w,x,11
2. THIADIAZOLINE AND RELATED COMPOUNDS
In the course of the development of new angiotensin II (AII) receptor antagonists by Wakunaga Pharmaceutical Co.,6k,p,12 the Nagao group recognized a non-covalent bonded 1,5-type S···O close contact (2.542, 2.610, and 2.588 Å) in the X-ray crystalline structures of three (acylimino)thiadiazoline derivatives (1-3) which have potent AII receptor antagonistic activities.6p Thus, it could be speculated that the intramolecular non-covalent bonded 1,5-type S···O interactions observed in the molecules of 1-3 would influence not only the flat conformation of the (acylimino)thiadiazoline moiety of these compounds but also their AII receptor antagonistic activities.6p In fact, the binding affinity of the oxadiazoline derivative (4) to the AII receptor (AT1) was eight times lower than that of the thiadiazoline
derivative (free form of 1), as shown in Figure 3.6p The ab initio calculations of several rotational isomers of the synthesized simple (acylimino)thiadiazoline derivatives at the various calculation levels revealed that the rotational isomers bearing the S···O interaction are most stable, which were also supported by their X-ray crystallographic analyses.6p The results caused us to consider a hypothesis that this fairly stable S···O interaction might be one of the important factors in causing the potent AII antagonism of these thiadiazoline derivatives. Before our discovery of the intramolecular non-covalent bonded S···O interaction of pharmacologically important (acylimino)thiadiazoline derivatives, as described above, Gibson and his group8o revealed a hypervalent S···O interaction (ca. 2.45-2.7 Å) in the crystal structure of 2-(2-oxoalkylidene)-3,5-diaryl-2,3-dihydro-1,3,4-thiadiazoles (5-8). However, these are not drugs.
Thus, it is apparent that there are many organosulfur drugs, candidate compounds with potential for new drugs, and biologically active natural products having potential for intramolecular non-covalent bonded 1,4-, 1,5-, and 1,6-type S···O and S···N interactions (vide infra). With this in mind, coupled with our studies on the AII receptor antagonists, we envisaged inter- and intramolecular non-covalent bonded S···X (X = O, N, S, halogens, other heteroatoms, π, etc.) interactions providing a promising gateway for the design and development of new organosulfur drugs and new reactions.2-4 Specifically, the mimic heterocyclic moiety involving these intramolecular non-covalent bonded 1,4-, 1,5-, and 1,6-type S···X interactions, as shown in Figure 2, seemed to be regarded as the corresponding 4-, 5-, and 6-membered heterocycles consisting of both S and X atoms in the molecular recognition.6p The (acylimino)thiadiazoline moiety of 1-3 can be available as a mimic-fused [3.3.0]heterocyclic moiety, as shown in Figure 4.6p
Based on the difference of the binding affinity to the AII receptor between thiadiazoline and oxadiazoline derivatives (vide supra), we investigated the molecular structure characteristics of the trifluoroacetyl and trifluorothioacetyl derivatives (9-11) of aminothiadiazoles and aminooxadiazole. The X-Ray crystallographic structures of 9 and 10 indicated that they were dimeric structures bearing intermolecular hydrogen bondings and an intramolecular non-covalent bonded 1,5-type S···S or S···O interaction, as shown in Figure 5.6u In contrast, the X-ray crystallographic structure of 11 was shown to be dimeric and to involve ordinal intra- and intermolecular hydrogen bondings without any close contact.6u Similar dimeric structures due to intermolecular hydrogen bondings and an intramolecular non-covalent bonded 1,5-type S···O interaction were recognized in the X-ray crystallographic structures of tetrahydrofurancarboxamide (12) and thymidine derivative (13), as shown in Figure 6.13 Interestingly, hydrogen bondings in the dimeric structure of compound (12) bearing the non-covalent bonded S···O interaction in CDCl3 proved to be stronger than that in the compound (13) by their 1H NMR analyses (di-tert-butyl nitroxide radical-induced broadening effect).14 However, the existence of the dimeric structures of 12 and 13 was indicated by the chart of its cold-spray ionization mass spectrum (CSI-MS).13
Interesting intramolecular non-covalent bonded interactions between divalent selenium and halogen atoms have been independently reported by the Tomoda group,15 the Nakanishi group,16 and other groups.17
We demonstrated remarkable examples of intramolecular double non-covalent bonded O···S···X (X= F, Cl, Br, and I) interactions in new 2-halophenyl-5-acylimino-thiadiazoline derivatives (14-17) obtained by our synthetic methods (Figure 7).2,18 These particular compounds were characterized in terms of X-ray crystallographic analyses as well as computational evaluations of the model compounds using quantum chemical calculations based on the DFT.
The dimeric crystalline structures of acetazolamide (18 : a diuretic drug based on inhibition to carbonic anhydrases I and II) and thiadiazolinethione (19 : a stromelysin inhibitor) bearing intramolecular non-covalent bonded S···O interactions (2.756 and 2.963 Å) were clarified by their X-ray crystallographic analyses (Figure 8).6v The existence of the dimeric structures of 18 and 19 in MeOH solution was suggested on the basis of their CSI-MS analyses.6v The intramolecular non-covalent bonded S···O interactions (2.37, 2.84, 2.72, and 2.98 Å) were precisely recognized in their complex crystalline structures of carbonic anhydrase I inhibitor [monomeric acetazolamide (18)] and stromelysin inhibitors [monomeric thiadiazolinethiones (19-21)] with the corresponding specific enzyme (Figure 9).6v
The computational evaluation of the possible seven monomeric conformers of 18 and model compounds (22 and 23) of 19-21 based on the DFT calculations at the B3LYP/6-31G(d) level demonstrated that the conformer bearing the intramolecular non-covalent bonded S···O interaction is most stable, as shown in Figure 10. Thus, the relative energy profile (△E kcal/mol) of the possible seven conformers can be affected by non-covalent bonded S···O attraction, dipole-dipole repulsion between the C=O group and C=N group, or conjugation of the HN-lonepair electron with the C=N group.
3. β-LACTAM ANTIBIOTICS
Ishiguro’s group reported an interesting 1,4-type S···O close contact (2.88 Å) between the S atom of the thiazolidine moiety and the O atom of the tetrahydofuranyl moiety in the X-ray crystalline structures of a new penem-type antibiotic, faropenem sodium (24).6n Characterization [crystal structure, intramolecular non-covalent bonded 1,5-type S···O interaction (2.976 Å), conformational analysis, and CH···O hydrogen bonding] of a novel 1β-methylcarbapenem antibiotic drug, biapenem (commercial name by
Meiji Seika Pharma Co., Ltd.: omegacin) (25), developed by the Lederle (Japan) and Nagao groups,6q,19 was clarified by its X-ray crystallographic, 1H NMR, and deuteration experiment analyses.6q This kind of intramolecular non-covalent bonded 1,5-type S···O interaction may be expected even in other carbapenem β-lactam antibiotics such as imipenem (26),20 meropenem (27),21 lenapenem (28),22 doripenem (29),23 and ertapenem sodium (30).24 We synthesized new 1β-methylcarbapenem antibiotics (31 and 32) having the potential for intramolecular non-covalent bonded S···O interaction based on the X-ray crystallographic analysis of a related compound (33).6t Both carbapenem antibiotics (31 and 32) exhibited significant antibacterial activities.6t
4. THIAZOLE AND RELATED COMPOUNDS
Thiazole, thiazoline, thiazolidine, and their related heterocyclic moieties are often involved in the molecules of antibiotic and anticancer drugs, marine and microbial bioactive natural products, and agricultural chemicals.5-7,10
Goldstein and his colleagues have extensively studied the intramolecular non-covalent bonded 1,4-type S···O interaction (2.985 Å) in a thiazole nucleoside analogue, tiazofurin (34),8m which plays an important role in the mechanism of several biological effects, antitumor activity and inhibition against inosine monophosphate dehydrogenase.6a,h,10c,d,f However, Makara and Keseru proposed another explanation based on the conformation of tiazofurin analogues.10e,f
In order to examine possible mechanisms of bioluminescence of firefly D-(-)-luciferin (35), Blank and his coworkers determined the structure of luciferin (35) by X-ray crystallographic analysis.25 However, they did not focus on the non-covalent bonded S···N interaction in the molecule of luciferin (35). Therefore, we synthesized dl-luciferin26 and then attempted its crystallization for the X-ray crystallographic analysis. Because a desired crystal of dl-luciferin (35) suitable for X-ray analysis was not obtained, we synthesized two kinds of luciferin-like compounds (36 and 37).26b Fortunately both intramolecular non-covalent bonded S···N interactions were recognized in their X-ray crystallographic structures, as shown in Figure 11. Hence the same predominant conformation of D-(-)-luciferin (35) as in the compounds (36 and 37) is expected even in the complex molecule with the enzyme, luciferase.27 The computational evaluation of the possible seven monomeric conformers of model compounds (38 and 39) of 35-37 based on the DFT calculations at the B3LYP/6-31G(d) level indicated that the conformer bearing both the intramolecular non-covalent bonded S···N interactions is most stable, as shown in Figure 12. Thus, the relative energy profile (△E kcal/mol) of the possible seven conformers may be affected by both non-covalent bonded S···N attractions and dipole-dipole repulsion between both C=N groups, etc.
Umezawa and his coworkers reported the X-ray crystallographic structure of bithiazole (40), which was isolated by the acid hydrolysis of a tumor-inhibitor bleomycin A2 in 1968 (Figure 13).28 In order to clarify the stereochemistry of myxothiazol (41), an antifungal antibiotic and an inhibitor of ubiquinol, Trowitzsch’s group reported the X-ray crystallographic structure of the bithiazole (42) obtained via sequential chemical reactions of myxothiazol (Figure 13).29
Because Trowitzsch and his co-workers did not describe the non-covalent bonded S···N interaction in the molecular of 42, we synthesized mono-thiazoles (43 and 44), bi-thiazoles (45 and 46), and tri-thiazole (47).2,18 From the viewpoint of the X-ray crystallographic structures (45’ and 46’), a biologically important conformation of the bi-thiazole moieties of bleomycins and myxothiazol seems to be like one (45’) involving the non-covalent bonded S···N interaction (Figure 14). Interestingly, a tri-thiazole (47) exhibited considerable antitumor activities against several tumor cells.18 The computational evaluation of the possible seven conformers of model compounds (48 and 49) of bi-thiazoles (45 and 46) based on DFT calculations at the B3LYP/6-31G(d) level showed that the conformer bearing the intramolecular non-covalent bonded S···N interaction is most stable, as shown in Figure 15. Thus, the relative energy profile (△E kcal/mol) of the possible seven conformers of the structures (48 and 49) may be principally affected by non-covalent bonded S···N attraction, dipole-dipole repulsion between both C=N groups, and other minor factors.
In 2004, Haginoya and his colleagues revealed that a 5-methyl-4,5,6,7-tetrahydrothiazolo [5,4-c]pyridine-type compound (50) exhibited orally potent anti-fXa activity and evident prolongation of prothrombin time in rats.30 In an X-ray study of 50 and Gla-less fXa, they discovered a novel intramolecular non-covalent bonded 1,4-type S···O interaction in the crystal complex.30
In 2006, Jung and his colleagues demonstrated that quinazolines with a substituted aminothiazole at C4 posses potent Aurora A and B inhibitory activity and excellent selectivity against a panel of various serine-threonine and tyrosine kinases, as exemplified by compound (51).31 Compound (51) is a potent suppressor of the expression of phospho-histone H3 in tumor cells in vitro as well as in vivo. The importance of the C5’ position for substitution in the model compound (52) has been rationalized by ab initio calculations with the restricted Hartree-Fock (RHF) model at the 6-31G* level. The calculation revealed that the planer conformation with the sulfur of the thiazole next to the quinazoline N3 is strongly favored over the other possible planar conformations. This result may be due to an intramolecular non-covalent bonded S···N interaction.
We demonstrated the first example of intramolecular non-covalent bonded S···X (X= F, Cl, Br, and I) interactions in new 2-(2-halophenyl)-1,3-thiazole derivatives (53-56) (Figure 16) obtained by our synthetic methods.32 These particular compounds were characterized by X-ray crystallographic analyses (Figure 16) as well as computational evaluations of the model compounds as follows. The relative energy values (△E kcal/mol) of possible seven conformers [30° intervals (torsion angle, theta degree) of the S-C-C-CX (X = halogens) rotation ranging from 0° to 180°] of model compounds (57-60) were obtained by using quantum chemical calculations based on the DFT at BSLYP/6-31G(d) or B3LYP/LanL2DZ level, as shown in Figure 17. Among the seven conformers (57-60), each corresponding theta 0° planer conformer (0 kcal/mol) proved to be most stable in terms of its relative energy. The stability of intramolecular non-covalent bonded S···X (X= F, Cl, Br, and I) interactions may be explained in terms of an nX→σ* interaction between the halogen nX orbital and C-S σ* orbital. In each theta 90° perpendicular conformer, an insufficient nX→σ* interaction between the halogen nX orbital and C-S σ* orbital and an insufficient π-conjugation of the 2-halophenyl group with the 1,3-thiazole group may cause its relative instability. The instability of each theta 180° planer conformer seems to be due to extensive dipole-dipole repulsion between Fδ- (or Clδ-) and Nδ- atoms or steric repulsion between Br (or I) and N atoms competing with diminution of the π-conjugation stability.
5. SULFOXIDES AND SULFONES
Sodium rabeprazole (61) is one of the inhibitors of the gastric (H+·K+)-ATPase.33 Intramolecular non-covalent bonded S···N interactions in the X-ray crystallographic structures of some derivatives (62-64) of sodium rabeprazole (61) were demonstrated, as shown in Figure 18. An intermolecular non-covalent bonded S···N interaction between ethylmethylsulfoxide and pyridine in a MeOH solution was recognized (CSI-MS analysis).34 These results led us to estimate that the intramolecular non-covalent bonded S···N interaction exists in the drug (61) and its derivatives in a solution, and formed the 4-membered quasi-ring in 65 followed by the increase of the reactivity (1→2 shift of the pyridine N atom) of 65 to give the putative spiro sulfoxide (66), which is the key intermediate in the reaction cascade of 61 (Figure 19).34
Various intramolecular non-covalent bonded 1,4 and 1,5 types of S···O interactions in the sulfoxides (e.g., 67-69) and other sulfoxides have been observed in their X-ray crystallographic structures (Figure 20).8h,8w,8x In the amide moiety of the crystalline structure (68), an intramolecular non-covalent bonded 1,4-type S···O interaction (2.723 Å) that is stronger than that (2.884 Å) in the ester moiety can be recognized. In the X-ray crystallographic structures of chiral sulfoxides (RS,S-70 and RS,S-71), intramolecular non-covalent bonded S···O interactions were observed (Figure 20).8x Analyses of several sulfoxide complexes with N,N-dimethylacetamide (DMAC) or N-methyl-2-pyrrolidone (NMP) in a MeOH solution utilizing CSI-MS presented, for the first time, direct information on intermolecular non-covalent bonded S···O interactions between sulfoxides and an amide (or a lactam) in a solution (Figure 21).8x Thus, highly diastereo- and enantioselective asymmetric Pummerer reactions that incorporate intermolecular and intramolecular non-covalent bonded S···O interactions were developed by our group, as shown in Figure 22.8x
We found a very interesting X-ray crystallographic structure, i.e., that of the 1,4,7,10-tetrasulfonylcyclodecane・2DMF complex (72), as shown in Figure 23. In this structure, each amide carbonyl O atom of two DMF molecules is arranged for the S atom of two sulfonium groups even though the close contacts between both O and S atoms are insufficient.35
6. DISULFIDE AND RELATED COMPOUNDS
Five related antibiotic compounds, dithiolopyrrolone derivatives, named xenorhabdins were isolated from cultures of Xenorhabdus spp., bacteria symbiotically associated with insect-pathogenic nematodes.36 The X-ray crystallographic structure of a xanthydrol derivative (73) of xenorhabdin 1 (74) was reported by McInerney’s group in 1991.36 In that paper, however, they did not describe the intramolecular non-covalent bonded S···O close contact in the structure.
Hirayama and Matsuzawa reported the X-ray crystallographic structure of antitumor antibiotic leinamycin (75) in 1993.37 Gates and his coworkers revealed oxidative DNA cleavage by leinamycin (75) and simple 1,2-dithiolan-3-one 1-oxides (76-78).38 In 2000, Wu and Greer reported a study on the intramolecular non-covalent bonded S···O interaction in the DNA-cleaving antitumor antibiotic leinamycin (75).39 The experimental outcome from DFT (B3LYP/6-31G* level) and semiempirical (PM3) calculations on leinamycin (75) and its model molecule (79) provided evidence for the intramolecular non-covalent bonded 1,5-type S···O interaction and the detailed role of the adjacent amide group in the leinamycin molecule.
We found a rare intramolecular non-covalent bonded 1,6-type S···O interaction in the X-ray crystallographic structure of a marine natural antibiotic, thiomarinol B (80),6g,40 which was isolated from a marine bacterium, Alteromanas rava sp. Nov. SANK 73390, by the Sankyo research group.41 In this molecule, the adjacent amide group may play a role (e.g., stabilization of the unstable disulfide moiety) similar to that of leinamycin39 as reported by Wu and Greer.
7. VARIOUS CONSIDERATIONS ON THE NON-COVALENT BONDED INTERACTIONS
Kucsman and Kapovits introduced various mechanistic considerations of non-covalent bonded interactions in their excellent review paper.8h In the qualitative interpretation, the valence bond description of conjugated molecules bearing 1,5-type S···O close contacts is shown in typical structures (81-83). The ionic structure (82) exhibits the “electrostatic interaction” between S and O atoms. On the other hand, the structures (81 and 83) demonstrate the “covalent interaction” or “no-bond single-bond resonance” between S and O atoms. A delocalized σ-system (83) in the X-S···O sequence must refer to as a 3-center 4-electron bond (hypervalent bond).8h In addition, a delocalized π-system composed of the 6 electrons in 5 p-orbitals is possible, as shown in 84.
A typical 1,5-type S···O close contact can be considered in terms of frontier orbital theory, as shown in Figure 24. The close contact of S and O atoms in a linear X-S···O sequence may depend on an oxygen-HOMO (nO) sulfur-LUMO (X-S σ*) overlap. An interaction such as the HOMO-LUMO is effective in the case of the 1,5- and 1,6- type S···O close contact bearing an O atom with electron-donating groups (the nO HOMO-energy is rather high) and an S atom with electron-withdrawing groups (the X-S σ* LUMO-energy is rather low). However, in the case of intramolecular non-covalent bonded 1,3- and 1,4-types S···X (X = O, N, S, halogens, other heteroatoms, etc.) interactions, an effective orbital-orbital overlap (HOMO-LUMO interaction) may be difficult because of the wrong direction of both orbitals. Instead, an electrostatic charge (positive) – charge (negative) interaction must be plausible. In the molecule of tiazofurin (34), 1,4-type S···O interaction is interpreted as an electrostatic interaction between a negatively charged furanose oxygen and a positively charged sulfur based on the MNDO computations (Figure 25).8m Franchetti, Goldstein, and their colleagues10f reported that heteroatom charges (Figure 25) for tiazofurin and its related compounds were calculated by using at the DFT/6-31G**//RHF/3-21G* level of theory and partitioned using the natural bond orbital (NBO) method incorporated in Gaussian 98. Thus, the 1,4-type S···O close contact in the molecule of tiazofurin (34) seems to be explained by electrostatic interaction between the S (+0.50) and O (-0.51) atoms as polarized oppositely.10f
Although no quantitative data has yet emerged from our studies, the stability of an intramolecular non-covalent bonded 1,5-S (with electron-withdrawing groups: Ew )···X (with electron-donating groups: Ed) interaction may be due to nx → σ*(C-S) orbital overlap based on frontier orbital theory, as shown in Figure 26.6p
8. CONCLUSION
We realized that various non-covalent bonded S···X (X = O, N, S) interactions were recognized in the molecules of many organosulfur drugs and biologically active organosulfur compounds, as described above. Surprisingly, both intra- and intermolecular non-covalent bonded S···O interactions were confirmed to be efficient for the asymmetric Pummerer reactions.
Thus, the positive incorporation of the mimic cyclic heterocycle system consisting of the inter- and/or intramolecular non-covalent bonded S···X (X = O, N, S, halogens, other heteroatoms, etc.) interactions into the designed bioactive compounds, drugs, and reactants is encouraging for the development of new drugs and chemical reactions.
ACKNOWLEDGEMENT
I am very grateful to my exceptional coworkers, Prof. S. Sano, Dr. T. Hirata, Dr. H. Iimori, Dr. T. Honjo, Dr. S. Miyamoto, Dr. M. Miyamoto, Prof. K. Hayashi, Prof. S. Goto, Dr. M. Nakao, Dr. T. Kumagai, Dr. S. Tamai, and Dr. T. Abe for their devoted efforts and hard work. I would also like to express my gratitude to Dr. M. Shiro of the Rigaku Corporation for help with the X-ray crystallographic analyses and Prof. K. Yamaguchi and Dr. Y. Sei of Tokushima Bunri University (Kagawa) for help with the CSI-MS analyses and their fruitful discussions.
References
1. Although Kucksman and Kapovits summarized the variations of the sulfur-oxygen linkage (i.e., covalent S=O double and S-O single bonds, hypervalent S---O bond, nonbonded S…O interaction, and van der Waals S/O distance) in their review paper,8h I adopted a tentative term, “non-covalent bonded S…O interaction” instead of “nonbonded S…O interaction” in this paper. It is sometimes difficult to distinguish the “nonbonded S…O interaction” from the “hypervalent S---O bond” (V. I. Minkin and R. M. Minyaev, Chem. Rev., 2001, 101, 1274). CrossRef
2. Y. Nagao, Yakugaku Zasshi, 2002, 122, 1. CrossRef
3. Y. Nagao, MEDCHEM NEWS, 2007, FEB. No. 1, 112.
4. Y. Nagao, Farumashia, 2010, 46, 207.
5. (a) Y. Kishi, S. Matsuura, S. Inoue, O. Shimomura, and T. Goto, Tetrahedron Lett., 1968, 2847; CrossRef (b) C. Ireland and P. J. Scheuer, J. Am. Chem. Soc., 1980, 102, 5688; CrossRef (c) S. Carmeli, R. E. Moore, G. M. L. Patterson, T. H. Corbett, and F. A. Veleriote, J. Am. Chem. Soc., 1990, 112, 8195; CrossRef (d) W. H. Gerwick, P. J. Proteau, D. G. Nagle, E. Hamel, A. Blokin, and D. L. Slate, J. Org. Chem., 1994, 59, 1243; CrossRef (e) P. Wipf and S. Venkatraman, Synlett., 1997, 1 and references cited therein; CrossRef (f) J. D. White, T.-S. Kim, and M. Nambu, J. Am. Chem. Soc., 1997, 119, 103 and references cited therein. CrossRef
6. (a) B. M. Goldstein, F. Takusagawa, H. M. Berman, P. C. Srivastava, and R. K. Robins, J. Am. Chem. Soc., 1983, 105, 7416; CrossRef (b) J. V. Metzger, Comprehensive Heterocyclic Chemistry; Thiazoles and Their Benzo Derivatives, ed. by A. R. Katritzky and C. W. Rees, Pergamon Press, New York, 1984, Vol. 6, Part 4B (ed. by K. T. Potts) pp. 235-331 and references cited therein; (c) M. Hara, I. Takahashi, M. Yoshida, K. Asano, I. Kawamoto, M. Morimoto, and H. Nakano, J. Antibiot., 1985, 42, 333; CrossRef (d) S. M. Hecht, Acc. Chem. Res., 1986, 19, 383; CrossRef (e) P. Crews, Y. Kakou, and E. Quiñoà, J. Am. Chem. Soc., 1988, 110, 4365; CrossRef (f) M. Debono, R. M. Molloy, J. L. Occolowitz, J. W. Paschal, A. M. Hunt, K. H. Michel, and J. W. Martin, J. Org. Chem., 1992, 57, 5200; CrossRef (g) H. Shiozawa, T. Kagasaki, A. Torikata, N. Tanaka, K. Fujimoto, T. Hata, Y. Furukawa, and S. Takahashi, J. Antibiot., 1995, 48, 907; CrossRef (h) P. Franchetti, L. Cappellacci, M. Grifantini, A. Barzi, G. Nocentini, H. Yang, A. O’Cornor, H. N. Jayaram, C. Carrell, and B. M. Goldstein, J. Med. Chem., 1995, 38, 3829; CrossRef (i) Abstracts of the 5th Annual Meeting of Division of Medicinal Chemistry in Japan (Toyama), 1966; (j) T. Fujita, Biosc. Biotechnol. Biochem., 1996, 60, 557; CrossRef (k) T. Hirata, S. Goto, K. Tamura, M. Okuhira, and Y. Nagao, Bioorg. Med. Chem. Lett., 1997, 7, 385; CrossRef (l) A. Martínez, A. Castro, I. Cardelús, J. Llenas, and J. M. Palacios, Bioorg. Med. Chem., 1997, 5, 1275; CrossRef (m) G. M. Mokara and G. M. Keserû, J. Med. Chem., 1997, 40, 4154; CrossRef (n) R. Tanaka, Y. Oyama, S. Imajo, S. Matsuki, and M. Ishiguro, Bioorg. Med. Chem., 1997, 5, 1389; CrossRef (o) R. M. Rzasa, H. A. Shea, and D. Romo, J. Am. Chem. Soc., 1998, 120, 591; CrossRef (p) Y. Nagao, T. Hirata, S. Goto, S. Sano, A. Kakehi, K. Iizuka, and M. Shiro, J. Am. Chem. Soc., 1998, 120, 3104; CrossRef (q) T. Kumagai, S. Tamai, T. Abe, H. Matsunaga, K. Hayashi, I. Kishi, M. Shiro, and Y. Nagao, J. Org. Chem., 1998, 63, 8145; CrossRef (r) Y. Kanda, T. Ashizawa, S. Kakita, Y. Takahashi, M. Kono, M. Yoshida, Y. Saitoh, and M. Okabe, J. Med. Chem., 1999, 42, 1330; CrossRef (s) G. L. Patrick, An Introduction to Medicinal Chemistry, 2nd ed., Oxford University Press: New York, 2001; (t) Y. Nagao, H. Iimori, K. H. Nam, S. Sano, and M. Shiro, Chem. Pharm. Bull., 2001, 49, 1660; CrossRef (u) Y. Nagao, H. Iimori, S. Goto, T. Hirata, S. Sano, H. Chuman, and M. Shiro, Tetrahedron Lett., 2002, 43, 1709; CrossRef (v) Y. Nagao, T. Honjo, H. Iimori, S. Goto, S. Sano, M. Shiro, K. Yamaguchi, and Y. Sei, Tetrahedron Lett., 2004, 45, 8757. CrossRef
7. Tsuji, Book of Current Drug Information (in Japanese), Hirokawa Publishing Co., Tokyo, 2003.
8. (a) S. M. Johnson, C. A. Maier, I. C. Paul, J. Chem. Soc. (B), 1970, 1603; CrossRef (b) J. C. Martin and R. J. Arhart, J. Am. Chem. Soc., 1971, 93, 2341; CrossRef (c) I. Kapovits and A. Kálmán, J. Chem. Soc. Chem. Commun., 1971, 649; (d) I. C. Paul, J. C. Martin, and E. F. Perozzi, J. Am. Chem. Soc., 1971, 93, 6674; CrossRef (e) C. W. Perkins, J. C. Martin, A. J. Arduengo, W. Lau, A. Alegria, and J. K. Kochi, J. Am. Chem. Soc., 1980, 102, 7753; CrossRef (f) M. M. Olmstead, K. A. Williams, and W. K. Musker, J. Am. Chem. Soc., 1982, 104, 5567; CrossRef (g) J. T. Doi, R. M. Kessler, D. L. deLeeuw, M. M. Olmstead, and W. K. Musker, J. Org. Chem., 1983, 48, 3707; CrossRef (h) A. Kucksman and I. Kapovits, Organic Sulfur Chemistry: Theoretical and Experimental Advances, ed. by F. Bernardi, I. G. Csizmadia, and A. Mangini, Elsevier Scientific Publishing Co., Amsterdam, 1985, pp. 191-245 and references cited therein; (i) F. Iwasaki and K. Akiba, Acta Crystallogr., 1985, B41, 445; (j) F. Iwasaki, Acta Crystallogr., 1986, C42, 121; (k) F. Iwasaki and N. Furukawa, Acta Crystallogr., 1987, C43, 80; (l) R. S. Glass, L. Adamowicz, and J. L. Broeker, J. Am. Chem. Soc., 1991, 113, 1065; CrossRef (m) F. T. Burling and B. M. Goldstein, J. Am. Chem. Soc., 1992, 114, 2313; CrossRef (n) G. L’abbe, L. V. Meervelt, S. Emmers, W. Dehaen, and S. Toppet, J. Heterocycl. Chem., 1992, 29, 1765; CrossRef (o) N. Pandya, A. J. Basile, A. K. Gupta, P. Hand, C. L. MacLaurin, T. Mohammad, E. S. Ratemi, M. S. Gibson, and M. F. Richardson, Can. J. Chem., 1993, 71, 561; CrossRef (p) M. Yasui, S. Murata, F. Iwasaki, and N. Furukawa, Bull. Chem. Soc. Jpn., 1995, 68, 744; CrossRef (q) K. Ohtaka, M. Ohsugi, K. Yamamoto, M. Ohsawa, and K.-y. Akiba, J. Am. Chem. Soc., 1996, 118, 6355 and references cited therein; CrossRef (r) R. M. Minyaev and V. I. Minkin, Can. J. Chem., 1998, 76, 776; CrossRef (s) V. G. Nenajdenko, N. E. Shevchenko, and E. S. Balenkova, Tetrahedron, 1998, 54, 5353; CrossRef (t) K. Kobayashi, E. Koyama, K. Namatame, T. Kitaura, C. Kono, M. Goto, T. Obinata, and N. Furukawa, J. Org. Chem., 1999, 64, 3190; CrossRef (u) Y. Nagao, H. Nishijima, H. Iimori, H. Ushirogochi, H. Sano, and M. Shiro, J. Organomet. Chem., 2000, 611, 172; CrossRef (v) S. Yamada and T. Misono, Tetrahedron Lett., 2001, 42, 5459; CrossRef (w) Y. Nagao, S. Miyamoto, K. Hayashi, A. Mihira, and S. Sano, Chem. Pharm. Bull., 2002, 50, 558; CrossRef (x) Y. Nagao, S. Miyamoto, M. Miyamoto, H. Takeshige, K. Hayashi, S. Sano, M. Shiro, K. Yamaguchi, and Y. Sei, J. Am. Chem. Soc., 2006, 128, 9722. CrossRef
9. (a) A. Bondi, J. Phys. Chem., 1964, 68, 441; CrossRef (b) A. Kálmán and L. Párkányi, Acta Crystallogr., 1980, B36, 2372; (c) C. Cohen-Addad, J. -M. Savariault, and M. S. Lehman, Acta Crystallogr., 1981, B37, 1703; (d) C. Cohen-Addad, M. S. Lehmann, P. Becker, L. Párkányi, and A. Kálmán, J. Chem. Soc., Perkin Trans. 2, 1984, 191; CrossRef (e) G. L’abbe, L. V. Meervelt, S. Emmers, W. Dehaen, and S. Toppet, J. Heterocycl. Chem., 1992, 29, 1765. CrossRef
10. (a) B. M. Goldstein, D. T. Mao, and V. E. Marquez, J. Med. Chem., 1988, 31, 1026; CrossRef (b) F. T. Burling, B. Gabrielsen, and B. M. Goldstein, Acta Crystallogr., 1991, C47, 1272; (c) H. Li, W. H. Hallows, J. S. Punzi, V. E. Marquez, H. L. Carrell, K. W. Pnkiewicz, K. A. Watanabe, and B. M. Goldstein, Biochemistry, 1994, 33, 23; CrossRef (d) B. M. Goldstein, H. Li, W. H. Hallows, D. A. Langes, P. Franchetti, L. Cappellacci, and M. Grifantini, J. Med. Chem., 1994, 37, 1684; CrossRef (e) G. M. Makara and G. M. Keseru, J. Med. Chem., 1997, 40, 4154; CrossRef (f) P. Franchetti, S. Marchetti, L. Capepellacci, H. N. Jayaram, J. A. Yalowitz, B. M. Goldstein, J.-L. Barascut, D. Dukhan, J.-L. Imbach, and M. Grifantini, J. Med. Chem., 2000, 43, 1264. CrossRef
11. (a) Y. Nagao, T. Ikeda, M. Yagi, and E. Fujita, J. Am. Chem. Soc., 1982, 104, 2079; CrossRef (b) Y. Nagao, T. Inoue, E. Fujita, S. Terada, and M. Shiro, J. Org. Chem., 1983, 48, 132; CrossRef (c) Y. Nagao, T. Ikeda, T. Inoue, M. Yagai, M. Shiro, and E. Fujita, J. Org. Chem., 1985, 50, 4072; CrossRef (d) Y. Nagao, S. Sano, S. Nakagawa, M. Tanaka, M. Shiro, and T. Taga, Chem. Lett., 1992, 21, 915; CrossRef (e) Y. Nagao, S. Sano, S. Nakagawa, M. Suzuki, M. Shiro, M. Kido, and T. Nishi, Heterocycles, 1993, 36, 1715; CrossRef (f) T. Honjo, S. Sano, M. Shiro, and Y. Nagao, Angew. Chem. Int. Ed., 2005, 44, 5838; CrossRef (g) T. Honjo, M. Nakao, S. Sano, M. Shiro, K. Yamaguchi, Y. Sei, and Y. Nagao, Org. Lett., 2007, 9, 509; CrossRef (h) Y. Nagao and S. Sano, J. Synth. Org. Chem., Jpn., 2003, 61, 1088. CrossRef
12. (a) T. Hirata, J. Nomiyama, N. Sakae, K. Nishimura, M. Yokomoto, S. Inoue, K. Tamura, M. Okuhira, H. Amano, and Y. Nagao, Bioorg. Med. Chem. Lett., 1996, 6, 1469; CrossRef (b) K. Tamura, M. Okuhira, H. Amano, K. Inokuma, T. Hirata, I. Mikoshiba, and K. Hashimoto, J. Cardiovasc. Pharmacol., 1997, 7, 607. CrossRef
13. Unpublished results by Y. Nagao, H. Iimori, S. Goto, S. Sano, M. Shiro, K. Yamaguchi, and Y. Sei.
14. I. Morishima, T. Inubushi, T. Yonezawa, and Y. Kyogoku, J. Am. Chem. Soc., 1977, 99, 4299. CrossRef
15. (a) M. Iwaoka, H. Komatsu, and S. Tomoda, Chem. Lett., 1998, 969; CrossRef (b) M. Iwaoka, H. Komatsu, T. Katsuda, and S. Tomoda, J. Am. Chem. Soc., 2002, 124, 1902. CrossRef
16. (a) W. Nakanishi, S. Hayashi, A. Sakaue, G. Ono, and Y. Kawada, J. Am. Chem. Soc., 1998, 120, 3635; CrossRef (b) W. Nakanishi and S. Hayashi, J. Org. Chem., 2002, 67, 38. CrossRef
17. (a) G. A. Landrum and R. Hoffmann, Angew. Chem. Int. Ed., 1998, 37, 1887; CrossRef (b) M. Iwaoka, H. Komatsu, and S. Tomoda, J. Organomet. Chem., 2000, 611, 164; CrossRef (c) M. Jeong and Y. Kwon, Chem. Phys. Lett., 2000, 328, 509. CrossRef
18. Unpublished results by Y. Nagao, H. Iimori, T. Honjo, S. Goto, S. Sano, and M. Shiro.
19. (a) Y. Nagao, T. Kumagai, S. Tamai, T. Abe, Y. Kuramoto, T. Taga, S. Aoyagi, M. Ochiai, Y. Inoue, and E. Fujita, J. Am. Chem. Soc., 1986, 108, 4673; CrossRef (b) Y. Nagao, T. Kumagai, Y. Nagase, S. Tamai, Y. Inoue, and M. Shiro, J. Org. Chem., 1992, 57, 4232; CrossRef (c) Y. Nagao, Y. Nagase, T. Kumagai, Y. Kuramoto, S. Kobayashi, Y. Inoue, T. Taga, and H. Ikeda, J. Org. Chem., 1992, 57, 4238; CrossRef (d) Y. Nagao, Y. Nagase, T. Kumagai, H. Matsunaga, T. Abe, O. Shimada, T. Hayashi, S. Kobayashi, and Y. Inoue, J. Org. Chem., 1992, 57, 4243. CrossRef
20. (a) W. J. Lenza, K. J. Wildonger, T. W. Miller, and B. G. Christensen, J. Med. Chem., 1979, 22, 1435; CrossRef (b) S. R. Norrby, K. Alestig, F. Fereer, M. Kahan, J. S. Kahan, H. Kropp, and M. A. Meisinger, Current Chemother. Immunother. (Proceeding of the 12th ICC), 1982, Vol. I, 743.
21. M. Sunagawa, H. Matsumura, Y. Inoue, M. Fukasawa, and M. Kato, J. Antibiot., 1990, 43, 519. CrossRef
22. N. Ohtake, O. Okamoto, R. Mitomo, Y. Kato, K. Yamamoto, Y. Haga, Y. Fukatsu, and S. Nakagawa, J. Antibiot., 1997, 50, 598. CrossRef
23. Y. Sendo, M. Kii, Y. Nishitani, T. Irie, and Y. Nishino, Eur. Pat. Appl., 1993, EP 557122 A1.
24. M. J. Betts, G. M. Davies, and M. L. Swain, PCT Int. Appl., 1993, WO 9315078 A1.
25. G. E. Blank, J. Pletcher, and M. Sax, Biochem. Biophys. Res. Commun., 1971, 42, 583. CrossRef
26. (a) Y. Toya, M. Takagi, H. Nakata, N. Suzuki, M. Isobe, and T. Goto, Bull. Chem. Soc. Jpn., 1992, 65, 392; CrossRef (b) Unpublished results by Y. Nagao, T. Honjo, S. Sano, and M. Shiro.
27. T. Hirano, Y. Hasumi, K. Ohtsuka, S. Maki, H. Niwa, M. Yamaji, and D. Hashizume, J. Am. Chem. Soc., 2009, 131, 2385. CrossRef
28. (a) G. Koyama, H. Nakamura, Y. Muraoka, T. Takita, K. Maeda, H. Umezawa, and Y. Iitaka, Tetrahedron Lett., 1968, 4635; CrossRef (b) H. Umezawa, Med. Chem., 1980, 16, 147; (c) C. A. Claussen and E. C. Long, Chem. Rev., 1999, 99, 2797; CrossRef (d) M. L. Sznaidman and S. M. Hecht, Org. Lett., 2001, 3, 2811. CrossRef
29. W. Trowitzsch, G. Höfle, and W. S. Sheldrick, Tetrahedron Lett., 1981, 22, 3829. CrossRef
30. N. Haginoya, S. Kobayashi, S. Komoriya, T. Yoshino, M. Suzuki, T. Shimada, K. Watanabe, Y. Hirokawa, T. Furugori, and T. Nagahara, J. Med. Chem., 2004, 47, 5167. CrossRef
31. F. H. Jung, G. Pasquet, C. L.-van der Brempt, J.-J. M. Lohmann, N. Warin, F. Renaud, H. Germain, C. D. Savi, N. Roberts, T. Johnson, C. Dousson, G. B. Hill, A. A. Mortlock, N. Heron, R. W. Wilkinson, S. R. Wedge, S. P. Heaton, R. Odedra, N. J. Keen, S. Green, E. Brown, K. Thompson, and S. Brightwell, J. Med. Chem., 2006, 49, 955. CrossRef
32. Unpublished results by Y. Nagao, T. Honjo, H. Iimori, S. Goto, S. Sano, and M. Shiro.
33. (a) M. Morii, H. Takata, H. Fujisaki, and N. Takeguchi, Biochem. Pharmacol., 1990, 39, 661; CrossRef (b) H. Fujisaki, K. Oketani, H. Shibata, M. Murakami, M. Fujimoto, T. Wakabayashi, I. Yamatsu, and N. Takeguchi, Nippon Yakurigaku Zasshi, 1993, 102, 389. CrossRef
34. K. Hayashi, S. Ogawa, S. Sano, M. Shiro, K. Yamauchi, Y. Sei, and Y. Nagao, Chem. Pharm. Bull., 2008, 56, 802. CrossRef
35. Unpublished results by Y. Nagao, Y. Ikee, S. Sano, and M. Shiro.
36. B. V. McInerney, R. P. Gregson, M. J. Lacey, R. J. Akhurst, G. R. Lyons, S. H. Rhodes, D. R. J. Smith, L. M. Engelhardt, and A. H. White, J. Nat. Prod., 1991, 54, 774. CrossRef
37. N. Hirayama and E. S. Matsuzawa, Chem. Lett., 1993, 1957.
38. K. Mitra, W. Kim, J. S. Daniels, and K. S. Gates, J. Am. Chem. Soc., 1997, 119, 11691. CrossRef
39. S. Wu and A. Greer, J. Org. Chem., 2000, 65, 4883. CrossRef
40. Various intramolecular non-covalent bonded S···O interactions of organosulfur bioactive compounds involving thiomarinol B were described by Y. Nagao at the Seminars (Naruto in 2002 and Tokyo in 2003) of the Society of Synthetic Organic Chemistry, Japan. See the abstract papers, pp. 35-40 and pp. 65-73, respectively.
41. H. Shiozawa, A. Shimada, and S. Takahashi, J. Antibiot., 1997, 50, 449. CrossRef