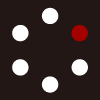
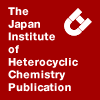
HETEROCYCLES
An International Journal for Reviews and Communications in Heterocyclic ChemistryWeb Edition ISSN: 1881-0942
Published online by The Japan Institute of Heterocyclic Chemistry
e-Journal
Full Text HTML
Received, 24th May, 2012, Accepted, 15th June, 2012, Published online, 21st June, 2012.
DOI: 10.3987/COM-12-S(N)21
■ A CONCISE SYNTHESIS OF THE AB-RING FRAGMENT OF (−)-GAMBIEROL
Haruhiko Fuwa,* Kazuaki Hirota, and Makoto Sasaki*
Graduate School of Life Sciences, Tohoku University, 2-1-1, Katahira, Aoba-ku, Sendai, 980-8577, Japan
Abstract
We describe herein a concise synthesis of the AB-ring fragment of gambierol, wherein silver(I) trifluoromethanesulfonate-catalyzed 6-endo cyclization of a hydroxy ynone was exploited for the formation of the A-ring.(−)-Gambierol (1, Figure 1) is a structurally complex marine polycyclic ether natural product that was isolated from the cultured cells of the ciguatera causative dinoflagellate Gambierdiscus toxicus by Satake and co-workers.1 The entire structure of 1 was elucidated by extensive 2D-NMR analysis and application of a chiral anisotropic reagent. Satake et al. have reported that gambierol exhibits potent lethal toxicity against mice with a minimal lethal dose value of 50 µg/kg (ip) and that the neurological symptoms caused in mice resemble those shown by ciguatoxins, suggesting the possible role of 1 in ciguatera seafood poisoning. Unfortunately, detailed biological studies on 1 had been precluded for almost a decade, due to the extreme natural scarcity of 1. Motivated by the structural complexity as well as the biological aspects, we have successfully completed the first total synthesis of 1 and enabled material supply for extensive biological investigations.2,3 Consequently, we have identified that 1 inhibits voltage-gated potassium channels (Kv channels) in mice taste cells in low nanomolar concentrations,4 while 1 acts as a weak partial agonist of voltage-gated sodium channels in human neuroblastoma cells.5 Snyders and co-workers have shown that 1 selectively inhibits the Kv1 and Kv3 subfamilies and that 1 binds to the previously undescribed binding site present between the S5 and S6 segments of Kv3.1 channels.6 In addition, we have recently found that the EFGH-ring domain of 1 plays a crucial role in inhibiting voltage-gated potassium currents and that, in an in vitro model of Alzheimer’s disease, 1 and its truncated analogues are able to lower the amyloid β (Aβ) and hyperphosphorylated tau levels with a possible implication of Kv channel inhibition.7 Undoubtedly, 1 represents an intriguing molecular probe for the functional analysis of Kv channels as well as for understanding downstream events of Kv channel inhibition.
Toward an even more practical synthesis of 1, we describe herein a concise synthesis of the AB-ring fragment of 1. We envisioned that the AB-ring fragment 2 would be readily accessible from dihydropyrone 3, which in turn would be obtainable from the known tetrahydropyran 48 via standard chemistry (Scheme 1).
Our initial synthetic approach toward 2 involved cyclodehydration of 1,3-diketone 5 (Scheme 2).9 Protection of 4 with triethylsilyl chloride (TESCl) and Et3N in the presence of a catalytic amount of 4-(dimethylamino)pyridine (DMAP) gave TES ether 6 in 95% yield. Ozonolysis of the double bond delivered aldehyde 7 in 95% yield. Methylation with MeMgBr, followed by oxidation of the resultant alcohol with 2-iodoxybenzoic acid (IBX), provided methyl ketone 8 in 75% yield (two steps). Enolization of 8 with lithium hexamethyldisilazide (LHMDS) in the presence of ZnCl2 followed by addition of 4-benzyloxybutanal (9) (THF, −78 to −20 °C) afforded β-hydroxy ketone 10 as a 1.8:1 mixture of diastereomers in 96% combined yield (stereochemistry not determined). Oxidation of 10 was most efficiently performed with IBX in refluxing EtOAc10 to give 1,3-diketone 5 in 86% yield. Unexpectedly, cyclodehydration of 5 was found to be rather problematic. Treatment of 5 with (+)-10-camphorsulfonic acid (CSA) in methanol under reflux resulted in decomposition of the material, possibly due to the instability of the diketone moiety. Accordingly, 5 was reacted with CSA initially at room temperature to cleave the silyl ether and then at 60 °C to effect cyclodehydration. This modified procedure gave dihydropyrone 11 with concomitant loss of the benzylidene acetal in 41% yield, along with a mixture of several byproducts. Careful examination of the mixture revealed that the major byproduct was bicycle 12. Running the reaction with pyridinium p-toluenesulfonate (PPTS) as an acid only provided a mixture of 12 and unidentified byproducts. The structure of 12 was assigned on the basis of extensive NMR studies on 12 and its acetate 13 as shown. Upon cleavage of the benzylidene acetal, the steric repulsion existing between the 1,3-diaxial methyl groups of the tetrahydropyran would facilitate ring flipping, hemiacetal formation, and dehydration to produce 12.
The disappointing outcome of the cyclodehydration of 1,3-diketone 5 led us to investigate 6-endo cyclization of hydroxy ynone 14 as the revised approach toward dihydropyrone 3 (Scheme 3). We have previously shown that a variety of substituted dihydropyrones could be accessed based on silver(I) trifluoromethanesulfonate (AgOTf)-catalyzed cyclization of the corresponding hydroxy ynones.11,12 Addition of a lithium acetylide generated from 5-benzyloxypentyne (15)13 to aldehyde 7 gave propargylic alcohol 16 as a 1.6:1 mixture of diastereomers in 96% combined yield. Without separation, this material was oxidized with IBX to deliver ynone 17 in 98% yield. Removal of the TES group was effected by exposure to tetra-n-butylammonium fluoride (TBAF) buffered with acetic acid (93%). The resultant hydroxy ynone 14 was cyclized smoothly upon treatment with AgOTf in the presence of 2,6-di-tert-butylpyridine (DTBP),14 giving rise to dihydropyrone 3 in 88% yield.
Having established the reliable route to 3, our focus was shifted toward elaboration of the AB-ring fragment 2 (Scheme 3). Hydrogenation of 3 proceeded in a stereoselective manner to give ketone 18 in 89% yield as a single stereoisomer. The newly generated stereogenic center was established through an NOE experiment as shown. Stereoselective reduction of 18 to establish the C6 stereogenic center turned out to be a challenging task. Even bulky reducing agents such as diisobutylaluminum hydride (DIBALH) or L-selectride® provided the desired alcohol 19a with moderate diastereoselectivity (19a:19b = 1.4–1.8:1), while reduction with NaBH4 or LiAlH4 resulted in preferential formation of the undesired alcohol 19b (19a:19b = ca. 1:6). However, we were fortunate to find that these diastereomeric alcohols were readily separable by flash chromatography on silica gel, and the undesired 19b could be recycled by oxidation with IBX (80%).15 Finally, benzylation of 19a afforded bis(benzyl) ether 2 in 85% yield, successfully intercepting our previous synthesis of 1.
In conclusion, we have devised a concise synthesis of the AB-ring fragment 2 of (−)-gambierol, by exploiting AgOTf-catalyzed 6-endo cyclization of hydroxy ynone 14. The present synthesis allows an efficient access to 2 from the B-ring tetrahydropyran 4 in nine steps, which compares favorably with our previous synthesis (17 steps). Further studies on the chemistry and biology of (−)-gambierol and its synthetic analogues are currently underway and will be reported shortly.
ACKNOWLEDGEMENTS
This work was financially supported in part by Grants-in-Aid for Scientific Research on Innovative Areas “Chemical Biology Using Bioactive Natural Products as Specific Ligands” (Nos. 23102016 and 24102517) from the Ministry of Education, Culture, Sports, Science and Technology (MEXT).
References
1. M. Satake, M. Murata, and T. Yasumoto, J. Am. Chem. Soc., 1993, 115, 361; CrossRef A. Morohashi, M. Satake, and T. Yasumoto, Tetrahedron Lett., 1999, 50, 97. CrossRef
2. H. Fuwa, M. Sasaki, M. Satake, and K. Tachibana, Org. Lett., 2002, 4, 2981; CrossRef H. Fuwa, N. Kainuma, K. Tachibana, and M. Sasaki, J. Am. Chem. Soc., 2002, 124, 14983. CrossRef
3. I. Kadota, H. Takamura, K. Sato, A. Ohno, K. Matsuda, and Y. Yamamoto, J. Am. Chem. Soc., 2003, 125, 46; CrossRef I. Kadota, H. Takamura, K. Sato, A. Ohno, K. Matsuda, M. Satake, and Y. Yamamoto, J. Am. Chem. Soc., 2003, 125, 11893; CrossRef H. W. B. Johnson, U. Majumder, and J. D. Rainier, J. Am. Chem. Soc., 2005, 127, 848; CrossRef U. Majumder, J. M. Cox, H. W. B. Johnson, and J. D. Rainier, Chem. Eur. J., 2006, 12, 1736; CrossRef H. W. B. Johnson, U. Majumder, and J. D. Rainier, Chem. Eur. J., 2006, 12, 1747; CrossRef H. Furuta, Y. Hasegawa, and Y. Mori, Org. Lett., 2009, 11, 4382; CrossRef H. Furuta, Y. Hasegawa, and Y. Mori, Chem. Eur. J., 2010, 16, 7586. CrossRef
4. V. Ghiaroni, M. Sasaki, H. Fuwa, G. P. Rossini, G. Scalera, T. Yasumoto, P. Pietra, and A. Bigiani, Toxicol. Sci., 2005, 85, 657; CrossRef V. Ghiaroni, H. Fuwa, M. Inoue, M. Sasaki, K. Miyazaki, M. Hirama, T. Yasumoto, G. P. Rossini, G. Scalera, and A. Bigiani, Chem. Senses, 2006, 31, 673. CrossRef
5. M. C. Louzao, E. Cagide, M. R. Vieytes, M. Sasaki, H. Fuwa, T. Yasumoto, and L. M. Botana, Cell. Physiol. Biochem., 2006, 17, 257. CrossRef
6. E. Cuypers, Y. Abdel-Mottaleb, I. Kopljar, J. D. Rainier, A. L. Raes, D. J. Snyders, and J. J. Tytgat, Toxicon, 2008, 51, 974; CrossRef I. Kopljar, A. J. Labro, E. Cuypers, H. W. B. Johnson, J. D. Rainier, J. Tytgat, and D. J. Snyders, Proc. Natl. Acad. Sci. U. S. A., 2009, 106, 9896. CrossRef
7. E. Alonso, H. Fuwa, C. Vale, Y. Suga, T. Goto, Y. Konno, M. Sasaki, F. M. LaFerla, M. R. Vieytes, L. Giménez-Llort, and L. M. Botana, J. Am. Chem. Soc., 2012, 134, 7467. CrossRef
8. K. C. Nicolaou, D. A. Nugiel, E. Couladouros, and C.-K. Hwang, Tetrahedron, 1990, 46, 4517. CrossRef
9. K. Tsubone, K. Hashizume, H. Fuwa, and M. Sasaki, Tetrahedron, 2011, 67, 6600. CrossRef
10. J. D. More and N. S. Finney, Org. Lett., 2002, 4, 3001. CrossRef
11. H. Fuwa, S. Matsukida, and M. Sasaki, Synlett, 2010, 1239; CrossRef H. Fuwa, K. Mizunuma, S. Matsukida, and M. Sasaki, Tetrahedron, 2011, 67, 4995. CrossRef
12. For other reports on the AgOTf-mediated 6-endo cyclization of hydroxy ynones, see: S.-L. Shi, M. Kanai, and M. Shibasaki, Angew. Chem. Int. Ed., 2012, 51, 3932; CrossRef C. R. Reddy and B. Srikanth, Synlett, 2010, 1536; CrossRef K. C. Nicolaou, M. O. Frederick, A. C. B. Burtoloso, R. M. Denton, F. Rivas, K. P. Cole, R. J. Aversa, R. Gibe, T. Umezawa, and T. Suzuki, J. Am. Chem. Soc., 2008, 130, 7466; CrossRef C. Wang and C. J. Forsyth, Org. Lett., 2006, 8, 2997. CrossRef
13. Alkyne 15 was prepared from aldehyde 9 in two steps ((i) CBr4, Ph3P, CH2Cl2, 0 °C; (ii) n-BuLi, THF, −78 °C, 96% yield for the two steps).
14. Addition of DTBP was found to be essential for obtaining 3 in excellent yield.
15. All attempts at inverting the C6 stereogenic center by means of Mitsunobu reaction were unfruitful (p-NO2C6H4CO2H, Ph3P, diethyl azodicarboxylate, toluene or THF, reflux or p-MeOC6H4CO2H, n-Bu3P, N,N,N’,N’-tetramethyl azodicarboxamide,16 benzene, reflux).
16. T. Tsunoda, J. Otsuka, Y. Yamamiya, and S. Itô, Chem. Lett., 1994, 539. CrossRef