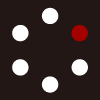
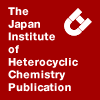
HETEROCYCLES
An International Journal for Reviews and Communications in Heterocyclic ChemistryWeb Edition ISSN: 1881-0942
Published online by The Japan Institute of Heterocyclic Chemistry
e-Journal
Full Text HTML
Received, 2nd June, 2012, Accepted, 13th July, 2012, Published online, 19th July, 2012.
DOI: 10.3987/COM-12-12518
■ An Improved Synthesis of 5-Acylamino-6-oxo-2-phenyl-1(6H)-pyrimidineacetic Acid from Glycine with Readily Removable Protecting Groups
Daisuke Takahashi,* Tatsumi Kashiwagi, Hiromi Onoye, Robert M. Williams, and Kunisuke Izawa*
Research Institute for Bioscience products and Fine chemicals, Ajinomoto Co., Inc., 1780 Hinaga, Yokkaichi, Mie, 510-0885, Japan
Abstract
Concise synthesis of N-acyl-5-amino-6-oxo-2-phenyl-1(6H)-yrimidineacetic acid was achieved by cyclization reaction of 2-alkyl-4-lkoxymethylene-5(4H)-oxazolone with N-(carboxymethyl)benzamidine, while a similar reaction with sodium salt of 2-alkyl-4-hydroxymethylene-5(4H)-xazolones gave a mixture of regioisomers of the pyrimidinone. N-Acyl groups (acetyl or phenylacetyl) of the pyrimidinone derivatives were readily cleaved under very mild conditions with weak base or enzyme. Thus, the process enabled us to synthesize the drug candidate without exchanging N-protecting group. Since the starting oxazolones were easily prepared from N-acylglycine, the synthetic route can be used for the large scale synthesis of the key intermediate for several enzyme inhibitors.INTRODUCTION
5-Amino-6-oxo-2-phenyl-1(6H)-pyrimidineacetic acid 1 was designed by mimicking the Ala-Pro moiety of a known serine protease inhibitor along with 3-amino-2-oxo-1(2H)-pyridineacetic acid, and was used as a core structure for the development of orally active non-peptidic inhibitors of human leukocyte elastase and chymase. Typical drug candidates (2-5) that contain 1 as a core molecule are shown in Figure 1.1-4
Several methods are available for the synthesis of pyrimidinone 1.5 To avoid the hazardous conditions and reagents used in the previous syntheses and to improve the economy and safety of the process, we have recently developed a new and efficient method (eq. 1) for synthesizing pyrimidinone 1 by using 2-phenyl-4-alkoxymethylene-5(4H)-oxazolone 7a, which is readily prepared from N-benzoylglycine 6a.6
This new process eliminated hazardous reactions. However, there were several drawbacks in the synthesis of the enzyme inhibitors shown in Figure 1. For example, 1 is easily dimerized under the conditions for peptide condensation if the amino group is not protected. In addition, we could selectively cleave the t-butyl ester of 9 to make the peptide bond, but it is difficult to remove the N-Bz (benzoyl) group selectively under mild conditions. To avoid these drawbacks, it was necessary to re-protect the 5-amino group with a Cbz (carbobenzyloxy) group for the following steps. Meanwhile, we found that an N-acetyl group was easily deprotected under remarkably mild reaction conditions. Based on these results, we decided to develop a new process to synthesize the pyrimidone intermediates efficiently.
RESULTS AND DISCUSSION
Synthetic approach to 1a via N-(benzyloxycarbonyl)-2-(hydromethylene)glycinate
To prepare the N-acyl (acetyl or phenacetyl) derivative of 5-amino-2-phenyl-4-pyrimidinone, it is necessary to obtain 2-alkyl(methyl or benzyl)-4-ethoxymethylene-5-oxazolone 7b or 7c in good yield. However, there are few reports on the synthesis of not only 7b but also 2-alkyl-4(H)-5-oxazolone itself.7 It has also been reported that the conventional Erlenmeyer method using N-acylglycine and acetic anhydride gave the desired products in very poor yield.8a To the best of our knowledge, there have been no reports on the direct synthesis of 7b and 7c from N-acylglycine, although there have been reports on the synthesis of 7a from hippuric acid (N-benzoylglycine).8
Thus, we re-examined the synthesis of 7b and 7c by the Erlenmeyer method and confirmed that the HPLC yields for 7b (25%, R=methyl) and 7c (33%, R=benzyl) were much less than that (>70%) for 7a. Inspired by suggestions in the literature,9 we tested the effect of additives on the reaction yield. As a result, we found that the HPLC yield was improved to 52% for 7b and 56% for 7c when we used a catalytic amount (0.1 equiv.) of ZnCl2/NaOAc as an additive. Though the reaction yield was slightly improved, there were still problems such as the formation of byproducts, difficult purification, and silica gel column chromatography was required.
Next, we tried to find an alternative way to obtain the 2-alkyl 4-oxymethylene-5-oxazolone in good yield. Stanovnik and co-workers reported that 4-[(N,N-dimethylamino)methylene]oxazolone 11b was prepared from N-acetylglycine 6b in yields of 51% and 55% by treatment with POCl3/DMF and DCC/DMF-DMA, respectively.10 According to this method, we could obtain 11b and 11c as crystalline solids in isolated yields of 76% and 73%, respectively.
Singh and Singh reported the addition-elimination reaction of 11b to replace the N,N-dimethylamino group at the β-position of the exo-methylene bond with a monoalkylamine.11 With this in mind, we examined the reaction of 11b with N-tert-butoxycarbonylmethylbenzamidine 8a. However, the reaction did not take place in toluene at reflux temperature to give the pyrimidinone 12b, and instead 11b was recovered almost quantitatively. When we used N-carboxymethylbenzamidine (8b) in DMF as a solvent, the desired cyclized product 13b was obtained in 32% yield at 130 oC after 12 h. We also changed the β-substituent of the exo-olefin from a N,N-dimethylamino- to a morpholine- or piperidino-group, but the yield was not improved. When we used unsubstituted benzamidine 14, we obtained the cyclization product 15 in modest yield (38%).
Based on these results, it seemed that the major reason for the low yield of the desired reaction might be the lower reactivity of not only N-substituted benzamidines 8a and 8b but also the dimethylamino group of the exo-methylene bond of the oxazolone 11b.
In a previous paper,6 we reported that the reactivity of tert-butoxycarbonylmethylbenzamidine hydrochloride (8a) toward methyl 2-oxymethyleneglycinate was strongly influenced by the β-substituent. Thus, we became interested in the conversion of 11b to its hydroxyl- or methoxy-derivative. Benoiton et al. obtained 2-phenyl-4-hydroxymethylene-5-oxazolone (16) in 88% yield from the ethoxy compound 7a by treatment with 1 equivalent of NaOH in water without decomposition of the oxazolone ring.12 Furthermore, Ikemoto et al. synthesized (α-hydroxymethylene)chloroacetate from ethyl chloroacetate (17) and isolated 18 as a stable potassium salt.13
Encouraged by these results, we carried out the hydrolysis of 11c under basic conditions, and found that the 2-benzyl-4-hydroxymethylene-5-oxazolene was obtained as a sodium salt (19c) as a mixture of E/Z isomer (80:20 from 1H-NMR) in 92% yield after precipitation from ethyl acetate. We also obtained the 2-methyl derivative 19b in a similar manner (94%) after precipitation from acetonitrile. 19b was also a mixture of E/Z isomer (80:20 from 1H-NMR). The ring-opening reaction of the oxazolone was observed when ethanol was used as a solvent for hydrolysis.14
To clarify the molecular structure of 19c, we obtained single crystals from methanol as a methanol solvate, and performed X-ray crystallographic analysis. Interestingly, 19c was found to exist as a dicarbonyl form coordinated with sodium, as shown in Figure 2. It is well known that 11a exists as the Z-isomer.15 However, the stable form of the corresponding monoalkylamine analog of 11a was revealed to be the E-isomer based on an NMR study (inverse gated decoupling technique of exo-olefinic H and carbonyl C) by Norton Matos et al.8b It was assumed that an intermolecular hydrogen-bonding interaction may contribute to this stabilization. Coordination of oxygen atom to the sodium ion is reasonably presumed to stabilize the E-isomer (19c) in our case.
The ORTEP drawing of crystal structure of 19c. One methanol molecule is included in the crystal, and carbon and oxygen atoms of it are represented C12 and O4, respectively. The compound crystallized in the space group C2/c with unit cell dimensions of a = 25.67(3) Å, b = 6.276(8) Å, c = 15.52(3) Å, and b = 99.78(8)°. The diffraction data was collected to a maximum sinθ / λ value of 0.694, using the fully automatic data-collection system16 installed on the beam line 6C at the Photon Factory of the National Laboratory for High Energy Physics, Tsukuba, Japan. The crystal structure was determined by direct methods and refined by full-matrix least-squares to R1 = 6.4% and GOF = 1.06.
We further transformed sodium salts 19b and 19c into their corresponding β-methoxy, β-TMSO, β-acetoxy as well as β-benzyloxy derivatives in order to compare the relative reactivity in this series. First, we examined the cyclization reaction of 2-alkyl-4-hydroxymethylene-5-oxazolone with benzamidine derivatives. Thus, the sodium salts (19a-c) were reacted with tert-butoxycarbonylmethyl- benzamidine hydrochloride (8a) in acetonitrile at 80 °C for 18 h. Surprisingly, the desired reaction took place to give the cyclization products 12a-c along with the formation of a substantial amount of the isomers 20a-c in each case, though the conversions from 19a-c were relatively high (eq. 9). Attempts to improve the selectivity by protecting the OH group by acetylation or silylation were not very effective, though the cyclization yield including the isomer was increased to 95% in the case of TMS protection of 19c.
Next, we tried to prepare the alkoxymethyleneoxazolone derivatives 7d-f by methylation, and benzylation. Interestingly, the products mainly exist in the Z-form probably due to very facile isomerization. We then examined the cyclization reactions of 7d-f with 8a after the free form was created by treatment with a base. The reaction proceeded very smoothly in toluene at 80 °C to give 12b and 12c in very high yields with less than 3% of 20b-c. When we used 8b as an amidine derivative, the desired products were also obtained, though the yields were slightly lower than in the case of 8a and the reactions required a polar solvent such as isopropyl alcohol or DMF to dissolve 8b.
A possible reaction mechanism is shown in Scheme 1. It is well known that the alkoxy group in 7a can be easily replaced by an amine via addition-elimination at the β-position. In a similar fashion, an imino group of the amidine will add to the β-position of the exo-olefin bond of 7 to give an adduct, which is followed by a cyclization reaction to selectively give the pyrimidinone 12. In contrast, the N-1 isomer should be obtained if the imino nitrogen attacks the carbonyl group of oxazolone. Although the reason is not clear at this moment, the proportion of the undesired isomer with β-OH and β-OAc is greater than that with β-OMe.
This can likely be best understood in the context of why other compounds (R’ = OH, mesylate, acetate, TMS) give some of the undesired compound 20. The hydroxymethylene compound may provide the other product because of a possible intramolecular H-bonded enol structure as shown below. This should activate the lactone carbonyl group, which results in an initial competing attack at the oxazolone carbonyl residue to give the undesired product (20).
The other compounds (mesylate, acetate and silyl) that also give undesired isomers are likely to have an electron-withdrawing effect through the sigma bond framework of the enol ether system and also activate the carbonyl for attack. This electron-withdrawing effect is at odds with the resonance effect as shown for the alkoxy substrates shown below. The alkoxy substrates (R = Me, Et) likely exist primarily as the Z-isomer and do not activate the carbonyl for nucleophilic attack; rather, these electron-donating groups reduce the electrophilicity of the lactone carbonyl through the aromatic resonance form shown below. This is very likely a delicate balance of electron-donating effects as shown below and electron-withdrawing effects and H-bonded activation of the carbonyl group that bifurcates the two reaction pathways.
Deprotection
Deprotection of the N-benzoyl group of 9 required severe conditions such as NaOH in methanol at reflux-temperature for 24 h. Thus, we examined the effect of the substituent on the benzene ring on hydrolysis. However, no dramatic changes were observed with p-MeO-, p-Me, and p-Cl substitution, though the pyrimidine derivatives were readily prepared via their corresponding oxazolones.
Next, we tried to deprotect the N-acetyl group of 13b, which was found to be easily hydrolyzed to give 1 under extremely mild conditions, such as by exposure to 1M aqueous NaOH solution at room temperature for 1 h. We then further investigated hydrolysis under milder conditions using an enzyme. Although we examined several commercially available enzymes to hydrolyze the N-acyl group, only one, penicillin amidase, was effective for deprotecting the N-phenylacetyl group of the pyrimidinone 13c under very mild conditions.
In summary, t-butyl ester of the pyrimidinone 9 is easily deprotected under acidic conditions while deprotection of the N-benzoyl group required more severe conditions such as refluxing in MeOH with NaOH. However, it was found that the N-acetyl group of (13b) was readily cleaved under very mild conditions, such as at ambient temperature for 1 h in 1M aqueous NaOH solution. In the case of 13c, the N-phenylacetyl group was deprotected enzymatically (penicillin amidase) under neutral conditions at pH 7 in almost quantitative yield.
This methodology might be applicable to the late-stage deprotection of drug candidates, since they contain an amino acid moiety and labile acyl residues and therefore it should be possible to perform deprotection on other substrates under very mild conditions. In the present methodology, it is not necessary to exchange the initial protecting group, rendering the overall process highly efficient.
CONCLUSION
We have successfully synthesized 2-alkyl-4-hydroxymethylene-oxazolone sodium salts that were difficult to prepare by the conventional Erlenmeyer method. Alkylation of the hydroxyl group of the exo-olefin bond leads to the desired product by minimizing formation of the undesired regioisomers. The pyrimidine thus obtained was confirmed to be readily deprotected under very mild hydrolytic conditions and therefore this process could be further applied to the synthesis of several drug candidates and enzyme inhibitors.
EXPERIMENTAL
All reagents were purchased and used without further purification. Thin-layer chromatography (TLC) was conducted on precoated TLC plates (Merck 60F250). High-performance liquid chromatography (HPLC) was performed with a Hitachi L-6000 pump and L-4000 UV detector system using an Inertsil ODS-2 column. Melting points were measured with a Büchi B-545 or a Yanaco melting point apparatus MP model and are uncorrected. NMR spectra were obtained on a Varian XL-300 spectrometer. All proton NMR spectra were measured in CDCl3 or DMSO-d6 solvent, and chemical shifts are reported as δ values in parts per million relative to tetramethylsilane (δ 0.00) or CDCl3 (δ 7.26) as an internal standard. Data are reported as follows: chemical shift (integrated intensity or assignment, multiplicity, coupling constants in hertz, assignment). All carbon NMR spectra were measured in CDCl3 or DMSO-d6 solvent, and chemical shifts are reported as δ values in parts per million relative CDCl3 (δ 77.0) or DMSO-d6 (δ 39.5) as an internal standard. Infrared (IR) spectra were recorded on a SHIMAZU IR Prestige-21 Fourier transform infrared spectrophotometer Smith Dura Sample IR II and are reported in wave number (cm-1). Mass spectra (MS) were obtained with a ThermoQuest TSQ700 a JEOL JMS-HX110 instrument with ESI (electrospray) or FAB (fast atom bombardment) ionization. High-resolution mass spectra (HRMS) were obtained with a JEOL MS700V by JEOL datum Ltd.
2-Alkyl(methyl or benzyl)-4-ethoxymethylene-5-oxazole-1-one (7b, 7c)
Compounds 7b and 7c were prepared according to the method reported by Singh11 except for the use of additives. Analytical data of 7b and 7c were fully consistent with those reported.
4-Dimethylaminomethylene-2-alkyl-5-oxazole-1-one (11b, 11c)
Compounds 11b and 11c were prepared according to the method reported by Stanovnik10 after some modifications. Analytical data of 11b and 11c were fully consistent with those reported.
5-Acetylamino-6-oxo-2-phenyl-1(6H)-pyrimidineacetic acid (13b)
To a solution of N-(carboxymethyl)benzamidine 8b (1.26 g, 7.09 mmol) and 21% of NaOEt/EtOH (2.23 g, 7.09 mmol) in DMF (15 mL) was added oxazolone 11b (1.09 g, 7.09 mmol) and the mixture was stirred at 130 °C for 12 h. The reaction mixture was concentrated and EtOAc (15 mL) and water (10 mL) were added. The aqueous layer was acidified with hydrochloric acid and extracted with EtOAc. To the organic layer was added hexane and the precipitate was collected and dried under reduced pressure to give 13b (651 mg, 31%) as pale yellow solid.
Mp 216-218 °C, IR (neat): 1732, 1680, 1523, 1365, 1246, 1102, 772, 714, 601 cm-1. 1H-NMR (DMSO-d6) δ 2.15 (3H, s), 4.52 (2H, s), 7.48-7.56 (5H, m), 8.83 (1H, s), 9.58 (1H, s). 13C-NMR (DMSO-d6) δ 24.0, 48.4, 125.4, 128.4, 129.0, 130.5, 134.4, 137.6, 153.9, 157.5, 169.1, 170.0. MS (ESI) m/z [M+H]+ 288, [M-H]+ 286. Anal. Calcd for C14H13N3O4: C, 58.53; H, 4.56; N, 14.63. Found: C, 58.26; H, 4.48; N, 14.50.
5-Acetylamino-2-phenyl-4(3H)-pyrimidinone (15)
To a solution of oxazolone 11b (500 mg, 3.24 mmol) in EtOH (7 mL) were added benzamidine hydrochloride 14 (560 mg, 3.58 mmol) and Et3N (0.5 mL) and stirred at 70 °C for 16 h. The reaction mixture was concentrated and purified by silica gel chromatography (hexane/EtOAc) to give 15 in 38% yield (282 mg, 1.23 mmol).
Mp 281-281.5 °C. IR (neat): 1636, 1603, 1533, 1505, 1372, 1310, 1258, 1071, 957, 882, 764, 696, 671, 658, 617, 596, 576, 530 cm-1. 1H-NMR (DMSO-d6) δ 2.15 (3H, s), 7.50-7.58 (3H, m), 8.06-8.09 (2H, m), 8.85 (1H, s), 9.50 (1H, s), 13.0 (1H, brs). 13C-NMR (DMSO-d6) δ 23.6, 125.2, 127.2, 128.6, 131.1, 132.0, 169.5, 150.5, 150.6, 169.5. MS (ESI) m/z [M+H]- 228. HRMS: Calcd for C12H11N3O2; m/z [M+H]+ 230.0929. Found: 230.0918.
Sodium salt of 4-hydroxymethylene-2-methyl-5-oxazol-1-one (19b)
To a solution of 11b (4.0 g, 26.0 mmol) in MeCN (50 mL) was added 2M NaOH (15 mL) in an ice bath and the mixture was stirred at room temperature for 14 h. The mixture was evaporated to dryness. To the residue was added acetonitrile (5 mL), and the mixture was stirred at 60 °C for 1 h. The precipitates were collected by filtration, washed with acetonitrile, and dried under reduced pressure at 80 °C to give 19b (3.65 g, 94%) as a crystalline solid.
Mp 251 °C, IR (neat): 1714, 1641, 1067, 904, 763, 630, 505, 470 cm-1. 1H-NMR (DMSO-d6) δ 2.00 (3H, s), 8.67 (0.8H, s), 8.90 (0.2H, s). 13C-NMR (D2O) δ 13.8, 111.6, 154.3, 173.8, 174.5. MS (APCI) m/z [M+H]+ 126. Anal. Calcd for C5H4NO3Na: C, 40.28; H, 2.70; N, 9.40. Found: C, 40.31; H, 2.42; N; 9.15.
Sodium salt of 4-hydroxymethylene-2-benzyl-5-oxazol-1-one (19c)
92% (crystallized from EtOAc)
Mp 235 °C, IR (neat): 1710, 1620, 1085, 916, 781, 696, 597, 491 cm-1. 1H-NMR (DMSO-d6) δ 3.66 (2H, s), 7.22-7.32 (5H, m), 8.71 (0.8H, s), 8.96 (0.2H, s). 13C-NMR (D2O) δ : 34.8, 111.5, 127.7, 129.2, 129.3, 135.4, 155.1, 173.6, 175.2. MS (ESI) m/z [M-H]+ 202. Anal. Calcd for C11H8NO3Na: C, 58.67; H, 3.58; N, 6.22. Found: C, 58.55; H. 3.34; N, 6.09.
General procedure for the reaction of oxazolone sodium salt 19 with amidine 8a.
To a suspension of N-(tert-butoxycarbonylmethyl)benzamidine hydrochloride 8a (120 mg, 0.44 mmol) in MeCN (3 mL) was added oxazolone sodium salt 19 (1.0 eq.) and vigorously stirred at 80 °C for 17 h. After the reaction, the mixture was filtered and concentrated. The residue was purified by silica gel chromatography to give 12 and 20.
5-Benzoylamino-6-oxo-2-phenyl-1(6H)-pyrimidineacetic acid tert-butyl ester (12a)
Mp 189-190 °C, IR: 1734, 1649, 1512, 1485, 1365, 1238, 1149, 771, 698, 597 cm-1. 1H-NMR (DMSO-d6) δ 1.31 (9H, s), 4.57 (2H, s), 7.51-7.65 (8H, m), 797-8.00 (2H, m), 8.79 (1H, s), 9.58 (1H, s). 13C-NMR (CDCl3) δ 27.9, 49.0, 83.3, 125.2, 125.3, 127.3, 128.2, 128.9, 128.9, 129.0, 130.5, 132.3, 133.7, 153.4, 158.4, 165.6, 166.2. MS (ESI) m/z [M+H]+ 406, Anal. Calcd for C23H23N3O4: C, 68.13; H, 5.72; N, 10.36. Found: C, 68.20; H, 5.65; N, 10.30.
5-Benzoylamino-4-oxo-2-phenyl-1(4H)-pyrimidineacetic acid tert-butyl ester (20a)
IR: 1731, 1633, 1516, 1610, 1458, 1240, 1149, 700, 686 cm-1. 1H-NMR (CDCl3) δ 1.45 (9H, s), 4.44 (2H, s), 7.46-7.55 (5H, m), 7.82-7.90 (5H, m), 8.83 (1H, s), 9.07 (1H, s). 13C-NMR (CDCl3) δ 28.3, 56.2, 84.6, 123.4, 126.5, 128.5, 129.0, 129.3, 129.4, 129.7, 130.8, 133.6, 133.8, 159.0, 164.6, 165.0, 171.1. HRMS: Calcd for C23H23N3O4 ; m/z [M+H]+ 406.1767. Found: 406.1762.
5-Acetylamino–6-oxo-2-phenyl-1(6H)-pyrimidineacetic acid tert-butyl ester (12b)
Mp 150-152 °C, IR: 3329, 1735, 1645, 1508, 1354, 1226, 1147, 771, 667, 590 cm-1. 1H-NMR (CDCl3) δ: 1.45 (9H, s), 2.23 (3H, s), 4.54 (2H, s), 7.48 (5H, m), 8.03 (1H, s), 9.09 (1H, s). 13C-NMR (CDCl3) δ 24.4, 27.9, 49.0, 94.1, 125.1, 128.1, 128.9 130.0, 134.0, 136.6, 153.3, 158.0, 166.1, 168.8. MS(ESI) m/z [M+H]+ 344. HRMS: Calcd for C18H21N3O4; [M+H]+ 344.1610. Found: 344.1584.
5-Acetylamino-4-oxo-2-phenyl-1(4H)-pyrimidineacetic acid tert-butyl ester (20b)
Mp 197-199 °C. IR (neat): 1748, 1505, 1516, 1465, 1433, 1234, 1201, 1166, 754, 705, 540 cm-1. 1H-NMR (CDCl3) δ 1.43 (9H, s), 2.22 (3H, s), 4.41 (2H, s), 7.45-7.51 (5H, m), 8.32 (1H, s), 8.62 (1H, s). 13C-NMR (CDCl3) δ 24.3, 27.9, 55.8, 84.3, 123.5, 128.1, 128.8, 130.7, 133.2, 158.6, 164.4, 165.8, 169.8. HRMS: Calcd for C15H15N3O4 [M+H]+ 344.1610. Found: 344.1587.
5-Phenylacetylamino-6-oxo-2-phenyl-1(6H)-pyrimidineacetic acid tert-butyl ester (12c)
Mp 111.0-111.5 °C. IR (neat): 1692, 1635, 1510, 1482, 1363, 1151, 760, 704, 524 cm-1. 1H-NMR (CDCl3) δ 1.40 (9H, s), 3.81 (2H, s), 4.58 (2H, s), 7.33-7.44 (5H, m), 7.53-7.67 (5H, s), 8.02 (1H, s), 9.10 (1H, s). 13C-NMR (CDCl3) δ 27.9, 44.2, 50.0, 84.2, 125.2, 125.8, 127.9, 128.5, 129.5, 130.0, 133.5, 133.5, 155.5, 156.4, 165.5, 170.4. HRMS: Calcd for C24H25N3O4 [M+H]+ 420.1923. Found: 420.1898.
5-Phenylacetylamino-4-oxo-2-phenyl-1(4H)-pyrimidineacetic acid tert-butyl ester (20c)
Mp 162-163 °C. IR (neat): 1741, 1610, 1593, 1510, 1470, 1230, 1215, 1160, 746, 696, 540 cm-1.
1H-NMR (CDCl3) δ 1.44 (9H, s), 3.75 (3H, s), 4.35 (2H, s), 7.30-7.37 (5H, m), 7.42-7.50 (5H, m), 8.38 (1H, s), 8.63 (1H, s). 13C-NMR (CDCl3) δ 28.3, 44.7, 56.2, 84.7, 123.8, 127.9, 128.5, 129.2, 129.3, 129.4, 129.7, 131.1, 133.6, 134.2, 159.1, 164.7, 166.1, 171.1. HRMS: Calcd for C24H25N3O4 [M+H]+ 420.1923. Found: 420.1903.
2-Methyl-4-methoxymethylene-5-oxazol-1-one (7d)
To a suspension of 19b (3.0 g, 20.1 mmol) in DMF (30 mL) was added Me2SO4 (2.20 mL, 23.2 mmol), and the mixture was stirred at 60 oC for 5 h. The solvent was removed by evaporation, EtOAc was added, and mineral salt was filtered off. The filtrate was washed with aq. NaHCO3 solution and brine. The organic layer was evaporated and purified by silica gel column chromatography (eluted with hexane/EtOAc) to give 7d as crystalline solid (1.73 g, 61%).
Mp 109-111 °C. IR (neat): 1689, 1636, 1531, 1433, 1248, 1142, 961, 916, 598, 552, 448 cm-1. 1H-NMR (CDCl3) δ 2.28 (3H, s), 4.11 (3H, s), 7.13 (1H, s). 13C NMR (CDCl3) δ 15.5, 63.5, 117.5, 152.9, 161.8, 168.9. MS (APCI) m/z [M+H]+ 142.1. HRMS: Calcd for C6H7NO3 [M+H]+ 142.0504. Found: 142.0488.
2-Methyl-4-benzyloxymethylene-5-oxazol-1-one (7e)
To a suspension of 19b (420 mg, 2.81 mmol) in DMF (5 mL) was added benzyl bromide (530 mg, 3.10 mmol) , and the mixture was stirred at 80 oC for 5 h. The solvent was removed by evaporation, toluene was added, and mineral salt was filtered off. The filtrate was washed with aq. NaHCO3 solution and brine. The organic layer was evaporated and purified by silica gel column chromatography (eluted with hexane/EtOAc) to give 7e as crystalline solid (370 mg, 60%).
Yield: 60%
Mp 76-77 °C. IR (neat): 1700, 1651, 1628, 1522, 1265, 1179, 1142, 976, 743, 698, 597, 476 cm-1. 1H-NMR (CDCl3) δ 2.27 (3H, s), 5.35 (2H, s), 7.27 (1H, s), 7.35-7.40 (5H, m). 13C NMR (CDCl3) δ 15.2, 78.0, 117.5, 128.2, 128.4, 128.8, 129.0, 151.0, 161.6, 168.5. MS (ESI) m/z [MH]+ 218.3. HRMS: Calcd for C12H11NO3 [M+H]+ 218.0817. Found: 218.0802.
2-Benzyl-4-methoxymethylene-5-oxazol-1-one (7f)
To a suspension of oxazolone 19c (3.0 g, 13.3 mmol) in MeCN (30 mL) was added Me2SO4 (1.64 mL, 17.3 mmol), and the mixture was stirred at 60 °C for 4 h. The reaction mixture was concentrated and EtOAc was added. The insoluble salt was filtered off. The filtrate was washed with aq. NaHCO3 solution and brine. The organic layer was evaporated and purified by silica gel column chromatography (eluted with hexane/EtOAc) to give 7f (1.99 g, 69%).
Mp 92-94 °C.17 IR (neat): 1771, 1755, 1668, 1254, 1148, 1036, 895, 878, 756, 698, 638 cm-1. 1H-NMR (CDCl3) δ 3.86 (2H, s), 4.10 (3H, s), 7.15 (1H, s), 7.25-7.37 (5H, m). 13C NMR (CDCl3) δ 35.9, 63.3, 117.2, 127.5, 128.8, 129.2, 133.3, 153.2, 162.5, 168.4. MS (ESI) m/z [M+H]+ 218.2. HRMS: Calcd for C12H11NO3 [M+H]+ 218.0817. Found: 218.0789.
General procedure for the preparation of 5-acylamino-6-oxo-2-phenyl-1(6H)-pyrimidineacetic acid tert-butyl ester 12 from oxazolone derivatives 7d-f and amidine 8a.
To a suspension of N-(tert-butoxycarbonylmethyl)benzamidine hydrochloride 8a (2.00 g, 7.39 mmol) in toluene (25 mL) was added Na2CO3 (2.3 g) and water (10 mL) with vigorous stirring until the substrate dissolved. After separation, the aqueous layer was extracted with toluene. The combined organic layer was washed with brine and dried over Na2SO4. The organic layer was added dropwise to a solution of the oxazolone 7d-f (5.97 mmol) in MeCN (5 mL) and stirred at 80 °C for 17 h. The mixture was washed with 1M aqueous HCl, saturated aqueous NaHCO3 solution and brine. After concentration, the resulting slurry was added dropwise to hexane and stirred. The crystals were filtered and dried under reduced pressure to give 12 as crystals. Analytical data of 12a-c were fully consistent with those obtained from 19a-c (vide infra).
General procedure for the preparation of 5-acylamino-6-oxo-2-phenyl-1(6H)-pyrimidineacetic acid 13 from oxazolone derivatives 7d-f and amidine 8b.
To a solution of N-(carboxymethyl)benzamidine 8b (1.26 g, 7.09 mmol) in iPrOH (15 mL) was added 21% of NaOEt/EtOH (2.23 g, 7.09 mmol). The mixture was stirred for 0.5 h. To the mixture was added oxazolone derivative 7d-f (7.09 mmol) in 4 portions for 1 h at 50 °C, and the mixture was stirred at 80 °C for 18 h. The reaction mixture was concentrated and EtOAc (15mL) and water (10mL) were added. The aqueous layer was acidified with aq. HCl and extracted with EtOAc. To the organic layer was added hexane and the precipitate was collected and dried under reduced pressure to give 13 as pale yellow solid.
5-Acetylamino-6-oxo-2-phenyl-1(6H)-pyrimidineacetic acid (13b) from 7d
Yield: 78%
Analytical data of 13b were fully consistent with those obtained from 11b (vide infra).
5-Phenylacetylamino-6-oxo-2-phenyl-1(6H)-pyrimidineacetic acid (13c)
Yield: 48%
Mp 154-155 °C. IR (neat): 1722, 1654, 1508, 1488, 1217, 1199, 774, 721, 705 cm-1. 1H-NMR (DMSO-d6) δ 3.84 (2H, s), 4.52 (2H, s), 7.25-7.27 (1H, m), 7.31-7.34 (4H, m), 7.47-7.55 (5H, m), 8.83 (1H, s), 9.72 (1H, s). 13C-NMR (DMSO-d6) δ 42.9, 48.5, 125.3, 127.0, 128.4, 128.8, 129.4, 129.6, 130.5, 134.4, 136.1, 137.6, 154.0, 157.5, 169.1, 170.8. MS (ESI) m/z [M+H]+ 364. [M-H] + 362. HRMS: Calcd for C20H17N3O4 [M+H]+ 364.1297. Found: 364.1267.
Deprotection of N-acetyl group of 4-aminopyrimidinones under weak basic conditions
5-Acetylamino-6-oxo-2-phenyl-1(6H)-pyrimidineacetic acid 13b (200 mg, 0.697 mmol) was stirred in 1 M aqueous NaOH (2 mL) for 1 h at room temperature. The mixture was neutralized to pH 3 with 6M hydrochloric acid. Resulting precipitates were collected by filtration and washed with water and vacuum dried to give 1 as crystals in 97% yield (165 mg, 0.676 mmol).
Analytical data of 1 were fully consistent with those reported previously by us.6
Deprotection of N-phenylacetyl group with enzyme
5-Phenylacetylamino-6-oxo-2-phenyl-1(6H)-pyrimidineacetic acid 13c (150 mg, 0.413 mmol) was dissolved in 0.01 M potassium phosphate buffer (pH 7.4, 3 mL) and aqueous penicillin amidase solution (Sigma) were added. The mixture was stirred overnight at 37 °C. The reaction mixture was neutralized to pH 3 with 6M hydrochloric acid and added MTBE. The precipitates were collected by filtration, washed with water and vacuum dried to give 1 as crystals in 95% yield (96 mg, 0.392 mmol).
ACKNOWLEDGEMENT
We thank Dr. N. Sakabe and Dr. K. Sakabe for helpful assistance for the data collection at the beam line 6C of the Photon Factory and thank Ms. Naoko Hirose for kind assistance and advice with NMR, IR and MP measurements.
References
1. C. A. Veale, P. R. Bernstein, C. Bryant, C. Ceccarelli, J. R. Damewood, Jr., R. Earley, S. W. Feeney, B. Gomes, B. J. Kosmider, G. B. Steelman, R. M. Thomas, E. P. Vacek, J. C. Williams, D. J. Wolanin, and S. Woolson, J. Med. Chem., 1995, 38, 98. CrossRef
2. Y. Odagaki, K. Ohmoto, S. Matsuoka, N. Hamanaka, H. Nakai, M. Toda, and Y. Katsuya, Bioorg. Med. Chem., 2001, 9, 647. CrossRef
3. F. Akahoshi, A. Ashimori, H. Sakashita, T. Yoshimura, M. Eda, T. Imada, M. Nakajima, N. Mitsutomi, S. Kuwahara, T. Ohtsuka, C. Fukaya, M. Miyazaki, and N. Nakamura, J. Med. Chem., 2001, 44, 1297. CrossRef
4. S. Takai, D. Jin, M. Nishimoto, A. Yuda, M. Sakaguchi, K. Kamoshita, K. Ishida, Y. Sukenaga, S. Sasaki, and M. Miyazaki, Life Sciences, 2001, 69, 1725. CrossRef
5. (a) K. Ohmoto, T. Yamamoto, T. Horiuchi, T. Kojima, K. Hachiya, S. Hashimoto, M. Kawamura, H. Nakai, and M. Toda, Synlett, 2001, 299; CrossRef (b) T. Kojima, T. Okada, K. Hachiya, T. Motoi, and S. Hashimoto, PCT Int. Appl., 2001, WO 2001023361; (c) T. Sugiura, T. Horiuchi, T. Miyazaki, T. Kojima, and S. Hashimoto, PCT Int. Appl., 2002, WO 2002051815.
6. D. Takahashi, Y. Honda, and K. Izawa, Heterocycles, 2012, 85, 1089. CrossRef
7. (a) P. Kumar, H. D. Mishra, and A. K. Mukerjee, Synthesis, 1980, 836; CrossRef (b) P. K. Tripathi and A. K. Mukerjee, Synthesis, 1985, 285; CrossRef (c) R. Cotton, P. M. Hardy, and A. E. Langran-Goldsmith, Int. J. Peptide Protein Res., 1986, 28, 230. CrossRef
8. (a) I. Ojima, K. Kato, and K. Nakahashi, J. Org. Chem., 1989, 54, 4511; CrossRef (b) M. R. P. Norton Matos, P. M. P. Gois, M. L. E. N. Mata, E. J. Cabrita, and C. A. M. Afonso, Synth. Commun., 2003, 33, 1285; CrossRef (c) D. Takahashi, Y. Honda, and K. Izawa, PCT Int. Appl., 2003 WO 2003106434.
9. (a) J. Kashyap, A. B. Chetry, and J. Das Pranab, Synth. Comm., 1998, 28, 4187; CrossRef (b) R. V. Vnkataratnam and P. S. Rao, Indian J. Chem., 1994, 33B, 984.
10. L. Kralj, A. Hvala, J. Svete, L. Golic, and B. Stanovnik, J. Heterocycl. Chem., 1997, 34, 247. CrossRef
11. R. S. Singh and R. M. Singh, Indian J. Chem., 2000, 39B, 688.
12. N. L. Benoiton, F. Hudecz, and M. F. Chen, Int. J. Peptide Protein Res., 1995, 45, 266. CrossRef
13. T. Ikemoto, T. Kawamoto, K. Tomimatsu, M. Takatani, and M. Wakimasu, Tetrahedron, 2000, 56, 7915. CrossRef
14. K. K. Singh, R. S. Radhey, and R. M. Singh, Indian J. Chem., 1998, 37B, 120.
15. M. K. Singh, K. K. Singh, R. S. Singh, and R. M. Singh, Indian J. Chem., 1997, 36B, 137.
16. K. Sakabe, K. Sasaki, N. Watanabe, M. Suzuki, Z. G. Wang, J. Miyahara, and N. Sakabe, J. Synchrotron Rad., 1997, 4, 136. CrossRef
17. S. T. Rolfson, J. H. Hunter, and J. W. Hinman, US 2555006 (1951).