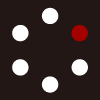
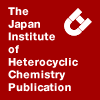
HETEROCYCLES
An International Journal for Reviews and Communications in Heterocyclic ChemistryWeb Edition ISSN: 1881-0942
Published online by The Japan Institute of Heterocyclic Chemistry
e-Journal
Full Text HTML
Received, 4th June, 2012, Accepted, 12th July, 2012, Published online, 20th July, 2012.
DOI: 10.3987/COM-12-12521
■ A New Series of Bisbenzimidazole Derivatives: Synthesis, Antitumor Activity and Low Toxicity on PBMC
Xiu-Jun Wang, Qing-He Wang,* Chao Liu, Wei Li, Jian Wang, Na Yang, and Mao-Sheng Cheng*
School of Pharmaceutical Engineering, Shenyang Pharmaceutical University, 103 Wenhua Road, Shenyang 110016, China
Abstract
Herein, a new series of bisbenzimidazole derivatives were designed and synthesized. Most of these new compounds showed significant in vitro anticancer activity when compared to Hoechst 33258 and 5-FU. Among them, the most potent compound 8 had the IC50 values of 0.78 µM for U937 tumor cell line, 5.62 µM for HL60 tumor cell line and 6.97 µM for Hela tumor cell line. Molecular modeling, fluorescence and viscosimetry study showed that compound 8 could bind into the minor groove of DNA. Subsequent toxicity study on human peripheral blood mononuclear cells (PBMC), shows that compound 8 exhibited less toxicity than paclitaxel and 5-FU.INTRODUCTION
Bis-benzimidazoles have been proven to be potent anti-tumor agents via DNA minor groove binding. Hoechst 33258,1 a fluorescent compound with a head-to-tail bis-benzimidazole structure, was initially found to be active against L1210 murine leukemia.
Phase I clinical trials of this compound showed positive responses towards pancreatic cancer. However, a subsequent phase II clinical trial failed to yield any objective results.2 Due to the synthetic accessibility and high binding affinity of Hoechst 33258 1, several groups have focused on a strategy to utilize the pharmacophore-like benzimidazole motif derived from Hoechst 33258 1.3-7 In 2009, our group have synthesized and evaluated the antitumor activities of a new series of bisbenzimidazole derivatives.8
To our knowledge, most derivatives of Hoechst 33258 1 keep whole molecules to planar. No literature related adding the linker between two benzimidazole has reported so far. Pentamidine is a symmetric aromatic diamidine with a linker between two benzene, which has a good activity against a variety of protozoa via DNA minor groove binding.9 Pentamidine’s linker makes it preference for AT-rich region of the DNA minor groove containing at least five consecutive AT base pairs.10,11 Based on these structural studies, molecular modelling has suggested to us that compound 8 adding a linker between two bis-benzimidazole could also effectively bind into the DNA minor groove (Figure 1). A series of derivatives were synthesized on the basis of modeling results. The interaction of compound 8 with CT (calf thymus)-DNA has been investigated using absorption spectroscopy and fluorescence spectroscopy. These results show that compound 8 interacted with the DNA by binding into the minor groove. All of these compounds were screened for anti-tumor activity in vitro. Among them, the most potent compound 8 had the good activity for three tumor cell lines. Subsequent toxicity study on human peripheral blood mononuclear cells (PBMC), shows that compound 8 exhibited less toxicity than paclitaxel and 5-FU.
RESULTS AND DISCUSSION
Molecular modeling
In order to determine the binding ability of compound 8 with the DNA minor groove, a molecular modeling study was carried out by the AutoDock 3.05 program.12 In our model, the X-ray crystallographic structure of the DNA dodecamer d(CGCAAATTTGCG) was selected from the Protein Data Bank (PDB code: 2DND) for the docking study. Figure 1 show that there are van der Waals contacts between compound 8 and the narrow minor groove. Compound 8 exactly fits into the convex minor groove in the model. In addition, four hydrogen bonds between compound 8 and DNA are formed between two benzimidazole NH group. Molecular modeling studies (shown in Figure 1) suggest that the novel symmetric bis-benzimidazole derivative (compound 8) could effectively bind into the DNA minor groove.
Synthesis
The synthesis of these bis-benzimidazoles13 is outlined in Scheme 2. 1,2-Bis(4-methyl- benzenesulfonyloxy)ethane14 was reacted with 4-amino-3-nitrophenol in dimethylformamide at 80 °C to synthesize 1,2-bis(4-amino-3-nitrophenyl-5-yl-oxy)ethane, followed by reduction of nitro group, gave 1,2-bis(4-amino-3-nitrophenyl-5-yl-oxy)ethane. Treatment of 1,2-bis(4-amino-3-nitrophenyl-5-yl-oxy)- ethane with various substituted benzaldehyde in methanol at reflux gave the desired compounds 8-15.
Inhibition of Cancer Cell Proliferation
All the newly synthesized compounds were investigated for their in vitro anti-tumor activities on three cancer cell lines using MTT assay.15 Table 1 reported the IC50 (µM) values (concentration required to achieve 50% inhibition of the tumor growth) of the tested compounds and the standards. With respect to the in vitro cytotoxic activities listed in Table 1, almost all of the synthesized compounds exhibited high antitumor activity when compared with known DNA minor groove agent Hoechst 33258. As shown in Table 1, compounds 8-11 with electron donating substituents (hydroxyl or methoxyl) on the benzene ring showed more potent antitumor activities than compounds 12-15, which only contains electron withdrawing halogen substituents (Cl, F or Br) on benzene ring. Compounds 8-11 showed low cytotoxicity at concentration of 10 µM while compounds 12-15 were less potent with IC50 values more than 10 µM. Among them, compound 8 was most potent with IC50 values of 0.78 µM for U937 tumor cell line, 5.62 µM for HL60 tumor cell line and 6.97 µM for Hela tumor cell line.
UV-Vis Absorption Spectral Studies
To understand the binding mode of the compound 8 with DNA, UV-Vis absorption spectral was carried out. The CT-DNA solution (10 mM) was titrated against compound 8 in 0.1 M Tris-HCl buffer pH 7.4 (Figure 2a). The absorption maxima at 254 nm is due to the CT-DNA. The absorbance of CT-DNA at 254 nm progressively increased when the concentration of compound 8 solution was increased from 0 to 20 mM. There was a distinct blue shift of DNA-compound 8 complex in the 254 nm region. Hoechst 33258 and Hoechst 33342 have the similar increase in absorbance of CT-DNA at 254 nm associated with blue shift.5 So, compound 8 may have the same binding ability with DNA minor groove.
Fluorescence Spectral Measurements
Fluorescence quenching measurements is effective to monitor the binding nature of the small molecules to DNA. The molecular fluorophore EB (ethidium bromide) has a conjugate planar structure and its fluorescence intensity is very weak, but it emits intense fluorescence at about 600 nm in the presence of DNA due to its strong intercalation between the adjacent DNA base pairs. Many DNA minor groove agents could quench the intense fluorescence.16 Similar quenching was observed in the compound 8 (Figure 2b). Therefore, it is concluded that compound 8 could bind into the minor groove of DNA.
Comparison of the toxicity of compound 8, paclitaxel and 5-FU on PBMC17
Paclitaxel and 5-FU are widely used as clinical antitumor drugs. We compared the toxicity on PBMC of compound 8 with paclitaxel and 5-FU (Figure 3). When human PBMC were cultured with 160 μM compound 8 for 12 h, 24 h, 48 h and 72 h, the viabilities of PBMC were about 94, 90, 85 and 60%. In the case of paclitaxel (160 μM) were about 90, 89, 77 and 47% respectively. 5-FU’s viabilities (160 μM) were about 92, 88, 81 and 54% respectively. In other concentration, the viabilities of compound 8 were also higher than paclitaxel and 5-FU. Thus, compound 8 has lower toxicity to normal PBMC than paclitaxel and 5-FU.
EXPERIMENTAL
Chemistry
All commercially available reagents and solvents were used without further purification unless otherwise specified. Solvents were dried and re-distilled prior to use according to standard methods. Melting points were determined on a Büchi Melting Point B-540 apparatus (Büchi Labortechnik, Flawil, Switzerland) and are uncorrected. 1H-NMR and 13C-NMR spectra were measured in DMSO-d6 on a Bruker ARX 300 spectrometer (Bruker, Rheinstetten, Germany). Chemical shifts are reported in parts per million (ppm) using tetramethylsilane (TMS) as internal standard if not specifically mentioned (J in Hz). Mass spectra were obtained on Waters Micromass Quattro Micro API mass spectrometer (Waters Corporation, Milford, United States).
Synthesis of compounds 8-15
1,2-Bis(4-amino-3-nitrophenyl-5-yl-oxy)ethane (5). Compound 3 (18.6 g, 0.050 mol) was mixed with 4-amino-3-nitrophenol and a solution of potassium carbonate (14.9 g, 0.108 mol) in dimethylformamide (10 mL) heated to 80 °C for 6 h. Then, the mixture was poured into water (100 mL) and filterated to obtained the crude product which was recrystallized from ethanol to afford compound 5 14.9 g. Colorless solid; Yield: 89.2%, mp 240.8-243.2 °C; 1H NMR (600 MHz, DMSO) δ: 4.26 (s, 4H, -CH2), 7.02 (d, J = 9.2 Hz, 2H, Bz-2), 7.22 (dd, J = 9.2, 2.8 Hz, 2H, Bz-6), 7.28 (s, 4H, -NH2), 7.45 (d, J = 2.8 Hz, 2H, Bz-5). 13C-NMR (600 MHz, DMSO-d6) δ: 148.7, 135.9, 132.6, 122.2, 118.6, 110.3, 67.5. MS (EI, m/z) 334 [M]+. Anal. Calcd for C14H14N4O6: C, 50.30; H, 4.22; N, 16.76. Found: C, 50.26; H, 4.18; N, 16.73.
1,2-Bis(3,4-diaminophenyl-5-yl-oxy)ethane (6). Hydrogen gas was passed through a stirred mixture of compound 5 (14.9 g, 0.044 mol), Pd-C (0.3 g, 10%) and methanol (200 mL) at a flow rate of 10 mL/min refluxing for 6 h. After filtration, the filtrate was evaporated under reduced pressure to give 6.78 g of compound 6 which was recrystallized from methanol. red solid; Yield: 56.2%, mp 298.6-301.5 °C; 1H NMR (600 MHz, DMSO) δ: 4.02 (s, 4H, -CH2), 6.01 (dd, J = 8.3, 2.4 Hz, 2H, Bz-6), 6.20 (d, J = 2.4 Hz, 2H, Bz-2), 6.42 (d, J = 8.3 Hz, 2H, Bz-5), 7.26 (s, 8H, -NH2). 13C-NMR (600 MHz, DMSO-d6) δ: 148.2, 136.1, 127.9, 116.3, 105.6, 101.4, 67.7. MS (EI, m/z) 274 [M]+. Anal. Calcd for C14H18N4O2: C, 61.30; H, 6.61; N, 20.42. Found: C, 61.26; H, 6.67; N, 20.39.
Compound 6 (0.5 g, 1.8 mmol), substituted benzaldehyde, sodium hydrogensulfite (0.37 g, 3.6 mmol), methanol (40 mL) were heated to reflux for 8 h. The mixture was evaporated under reduced pressure and obtained the compounds 8-15. Compounds 8-15 were purified by gel column chromatography (CHCl3:MeOH = 50:1).
General method for the synthesis of compounds 8-15.
1,2-Bis[2-(4'-methoxyphenyl)-1H-benzimidazole-5-yl-oxy]ethane (8). Colorless solid; Yield: 60.7%, mp 317.6-319.1 °C; 1H-NMR (600 MHz, DMSO-d6) δ: 3.91 (s, 6H, -OCH3), 4.52 (s, 4H, -CH2), 7.22 (dd, 2H, J = 2.4, 9.0 Hz, Bz-6), 7.29 (d, 4H, J = 9.0 Hz, Bz-3',5'), 7.32 (d, 2H, J = 2.4 Hz, Bz-4), 7.72 (d, 2H, J = 9.0 Hz, Bz-7), 8.27 (d, 4H, J = 9.0 Hz, Bz-2',6'), 15.09 (s, 2H, Bz-NH). 13C-NMR (600 MHz, DMSO-d6) δ: 161.2, 153.9, 149.3, 140.9, 133.4, 130.8, 115.8, 115.4, 114.6, 111.2, 104.2, 67.5, 56.5. MS (EI, m/z) 506 [M]+. Anal. Calcd for C30H26N4O4: C, 71.13; H, 5.17; N, 11.06. Found: C, 71.16; H, 5.11; N, 11.09.
1,2-Bis[2-(3',5'-methoxyphenyl)-1H-benzimidazole-5-yl-oxy]ethane (9). Colorless solid; Yield: 65.2%, mp 151.8-153.1 °C; 1H-NMR (600 MHz, DMSO-d6) δ: 3.85 (s, 12H, -OCH3), 4.42 (s, 4H, -CH2), 7.31 (s, 2H, Bz-4), 6.93 (dd, 2H, J = 2.4, 8.4Hz, Bz-6), 7.34 (d, 4H, J = 2.4 Hz, Bz-2', 6'), 7.58 (d, 2H, J = 8.4Hz, Bz-7), 6.60 (s, 2H, Bz-4'), 12.74 (d, 2H, Bz-NH). 13C-NMR (600 MHz, DMSO-d6) δ: 161.2, 153.4, 149.8, 140.2, 133.1, 132.5, 115.5, 111.0, 104.5, 104.2, 101.3, 67.3, 56.2. MS (EI, m/z) 566 [M]+. Anal. Calcd for C32H30N4O6: C, 67.83; H, 5.34; N, 9.89. Found: C, 67.78; H, 5.31; N, 9.86.
1,2-Bis[2-(3',4',5'-methoxyphenyl)-1H-benzimidazole-5-yl-oxy]ethane (10). Colorless solid; Yield: 68.7%, mp 199.6-202.5 °C; 1H-NMR (600 MHz, DMSO-d6) δ: 3.74 (s, 6H, -OCH3), 3.91 (s, 12H, -OCH3), 4.42 (s, 4H, -CH2), 6.92 (dd, 2H, J = 8.4 Hz, Bz-6), 7.30 (s, 2H, Bz-4), 7.49 (s, 4H, Bz-2', 6'), 7.57 (d, 2H, J = 8.4 Hz, Bz-7), 12.74 (s, 2H, -NH). 13C-NMR (600 MHz, DMSO-d6) δ: 161.2, 154.2, 149.1, 145.7, 141.5, 133.2, 125.7, 115.7, 111.5, 105.0, 104.2, 67.3, 60.6, 56.5. MS (EI, m/z) 626 [M]+. Anal. Calcd for C34H34N4O8: C, 65.17; H, 5.47; N, 8.94. Found: C, 65.15; H, 5.43; N, 8.92.
1,2-Bis[2-(3'-methoxy-4'-hydroxyphenyl)-1H-benzimidazole-5-yl-oxy]ethane (11). Colorless solid; Yield: 76.9%, mp 184-186 °C; 1H-NMR (600 MHz, DMSO-d6) δ: 3.91 (s, 6H, -OCH3), 4.52 (s, 4H, -CH2), 5.38 (s, 2H, -OH), 7.22 (d, 2H, J = 2.4 Hz, Bz-6 ), 7.27 (d, 2 H, J = 9.0 Hz, Bz-2'), 7.29 (d, 2H, J = 9.0 Hz, Bz-5'), 7.46 (dd, 2H, J = 2.4, 9.0 Hz, Bz-4), 7.72 (d, 2H, J = 9.0 Hz, Bz-7), 8.27 (dd, 2 H, J = 9.0 Hz, Bz-6'), 15.09 (s, 2H, -NH). 13C-NMR (600 MHz, DMSO-d6) δ: 153.6, 149.8, 147.9, 146.5, 140.7, 133.2, 126.7, 124.5, 116.2, 115.5, 113.6, 111.7, 104.0, 67.5, 56.3. MS (EI, m/z) 538 [M]+. Anal. Calcd for C30H26N4O6: C, 66.91; H, 4.87; N, 10.40. Found: C, 66.90; H, 4.85; N, 10.45.
1,2-Bis(2-phenyl-1H-benzimidazole-5-yl-oxy)ethane (12). Colorless solid; Yield: 72.4%, mp 263.9-266 °C; 1H-NMR (600 MHz, DMSO-d6) δ: 4.42 (s, 4H, CH2), 6.93 (dd, 2H, J = 2.4, 9.0 Hz, Bz-6), 7.19 (s, 2H, Bz-4), 7.50 (m, 6H, Bz-3', 4', 5'), 7.56 (d, 2H, J = 9.0 Hz, Bz-7), 8.15 (d, 4H, J = 7.2 Hz, Bz-2', 6'), 12.84 (s, 2H, Bz-NH). 13C-NMR (600 MHz, DMSO-d6) δ: 154.3, 149.5, 140.7, 132.6, 133.1, 130.5, 130.3, 129.5, 115.6, 111.8, 104.0, 67.3. MS (EI, m/z) 446 [M]+. Anal. Calcd for C28H22N4O2: C, 75.32; H, 4.97; N, 12.55. Found: C, 75.35; H, 4.96; N, 12.50.
1,2-Bis[2-(4'-bromophenyl)-1H-benzimidazole-5-yl-oxy]ethane (13). Colorless solid; Yield: 58.7%, mp 156-159 °C; 1H-NMR (600 MHz, DMSO-d6) δ: 4.52 (s, 4H, -CH2), 7.14 (d, 4H, J = 9.0 Hz, Bz-3',5'), 7.22 (dd, 2H, J = 2.4, 9.0 Hz, Bz-6), 7.32 (d, 2H, J = 2.4 Hz, Bz-4), 7.56 (d, 4H, J = 9.0 Hz, Bz-2',6'), 7.72 (d, 2H, J = 9.0 Hz, Bz-7), 15.09 (s, 2H, Bz-NH). 13C-NMR (600 MHz, DMSO-d6) δ: 154.1, 149.5, 141.3, 135.4, 133.6, 133.4, 132.9, 130.4, 128.2, 115.9, 111.8, 104.7, 67.8. MS (EI, m/z) 602 [M]+. Anal. Calcd for C28H20N4O2Br2: C, 55.65; H, 3.34; N, 9.27. Found: C, 55.62; H, 3.30; N, 9.25.
1,2-Bis[2-(2',4'-chlorophenyl)-1H-benzimidazole-5-yl-oxy]ethane (14). Colorless solid; Yield: 53.6%, mp 384.4-386.2 °C; 1H-NMR (600 MHz, DMSO-d6) δ: 4.52 (s, 4H, -CH2), 7.18 (dd, 2H, J = 1.8,9.0 Hz, Bz-5'), 7.22 (dd, 2H, J = 2.4,9.0 Hz, Bz-6), 7.32 (d, 2H, J = 2.4 Hz, Bz-4), 7.34 (d, 2 H, J = 1.8 Hz, Bz-3'), 7.36 (d, 2 H, J = 9.0 Hz, Bz-6'), 7.72 (d, 2H, J = 9.0 Hz, Bz-7), 15.09 (s, 2H, Bz-NH). 13C-NMR (600 MHz, DMSO-d6) δ: 153.1, 149.5, 140.2, 133.6, 133.1, 131.0, 134.5, 125.6, 115.0, 111.8, 104.9, 67.6. MS (EI, m/z) 582 [M]+. Anal. Calcd for C28H18N4O2Cl4: C, 57.56; H, 3.11; N, 9.59. Found: C, 57.54; H, 3.14; N, 9.55.
1,2-Bis[2-(4'-fluorophenyl)-1H-benzimidazole-5-yl-oxy]ethane (15). Colorless solid; Yield: 51.5%, mp 290-293 °C; 1H-NMR (600 MHz, DMSO-d6) δ: 4.52 (s, 4H, -CH2), 7.22 (dd, 2H, J = 1.8,9.0 Hz, Bz-6), 7.32 (d, 2H, J = 1.8 Hz, Bz-4), 7.36 (d, 4H, J = 9.0 Hz, Bz-2',6'), 7.50 (d, 4H, J = 9.0 Hz, Bz-3',5'), 7.72 (d, 2H, J = 9.0 Hz, Bz-7), 15.09 (s, 2H, Bz-NH). 13C-NMR (600 MHz, DMSO-d6) δ: 165.2, 153.6, 149.1, 140.7, 134.3, 133.3, 129.3, 116.5, 115.5, 111.7, 104.0, 67.7. MS (EI, m/z) 482 [M]+. Anal. Calcd for C28H20F2N4O2: C, 69.70; H, 4.18; N, 11.61; F, 7.88; O, 6.63. Found: C, 69.75; H, 4.13; N, 11.65.
Molecular modeling
The AutoDock 3.05 was used to perform docking calculations. The ligand structures were built in three-dimensionalcoordinates using Sybyl 6.91 software package and minimized using the Tripos force field and assigned charges using the Gasteiger–Hückel method. The molecular structure of the A-tract DNA dodecamer d(CGCAAATTTGCG) (PDB code: 2DND) was as receptor. The graphical front-end, AutoDockTools, was used to add polar hydrogens and partial charges for protein using the Kollman United Atom charges. Atomic solvation parameters were assigned using the addsol utility in the AutoDock package. Affinity grid fields were generated using the auxiliary program AutoGrid3.0. The Lamarckian genetic algorithm (LGA) was used to find the appropriate binding positions, orientations, and conformations of the ligands. The optimized AutoDocking parameters are as follows: the maximum number of energy evaluations was increased to 25,000,000 per run; the iterations of Solis & Wets local search was 3000; the number of individuals in population was 300. All other parameters were maintained as default. Cluster analysis with AutoDock results was performed to determine if different binding sites have been produced from multiple runs. Sybyl 6.91 software package was used for visualization.
Biological assays
The anti-proliferational effects of tumour cells were tested by the same methods. Tumor cells in RPMI1640 medium with 10% fetal bovine serum were plated in 96-well microtiter plates (4.0×104 cells per well), and allowed to adhere at 37 °C with 5% CO2 for 4 h. The test compound was then added, and the cells were incubated at 37 °C with 5% CO2 for 72 h. The cell viability was assessed using a standard MTT assay.
Spectral Measurements
The absorption spectral measurements were recorded on Cary Varian double beam spectrophotometer (Cary BIO 100, Australia). The sample cuvette used was a pair quartz cells of 1.00 cm path length. All scanning parameters were optimized to obtain the best spectra and in general the parameters were scan range 230-300 nm, wavelength step 0.5 and all measurements were carried out at room temperature.
Fluorescence Measurements
Fluorescence measurements were performed using Spectrofluorimeter model FS920 of Edinburgh Instruments, U.K. equipped with xenon arc lamp. The temperature of the sample holder was regulated with a peltier cooled thermostat. Quartz cuvettes of 3ml capacity, path length 1 cm were used for all measurements.
PBMC test
Blood was obtained from three healthy adult volunteers. PBMC were separated by density centrifugation in cell separation solution (Dalian Biological Reagent Factory) and suspended in RPMI-1640 medium containing 2% human AB type serum. PBMC 1×103 cells/well were added to the 96-well culture plates. Cell viability was measured by means of the trypan blue dry exclusion test.18
ACKNOWLEDGEMENTS
We thank Mr. Zi-Shuang Liu of Benxi Institute of Pharmaceutical Research for determining mass spectra and NMR analyses. This work was supported by the National Science and Technology Key Specific Project for Significant Creation of New Drugs of China (2009ZX09301-012).
References
1. B. S. Reddy, S. M. Sondhi, and J. W. Lown, Pharmac. Ther., 1999, 84, 1. CrossRef
2. E. H. Kraut, T. Fleming, M. Segal, J. A. Neidhart, B. C. Behrens, and J. MacDonald, Invest. New Drugs, 1991, 9, 95. CrossRef
3. S. Alper, O. Temiz Arpaci, E. Sener Aki, and I. Yalcin, Farmaco, 2003, 58, 497. CrossRef
4. J. Mann, A. Baron, Y. Opoku-Boahen, E. Johansson, G. Parkinson, L. R. Kelland, and S. Neidle, J. Med. Chem., 2001, 44, 138. CrossRef
5. M. Singh and V. Tandon, Eur. J. Med. Chem., 2004, 46, 659. CrossRef
6. T. Fonseca, B. Gigante, M. M. Marques, T. L. Gilchrist, and E. De Clercq, Bioorg. Med. Chem., 2004, 12, 103. CrossRef
7. J. Valdez, R. Cedillo, A. Hernandez-Campos, L. Yepez, F. Hernandez-Luis, G. Navarrete-Vazquez, A. Tapia, R. Cortes, M. Hernandez, and R. Castillo, Bioorg. Med. Chem. Lett., 2002, 12, 2221. CrossRef
8. Y. H. Yang, M. S. Cheng, Q. H. Wang, H. Nie, N. Liao, J. Wang, and H. Chen, Eur. J. Med. Chem., 2009, 44, 1808. CrossRef
9. S. F. Queener, J. Med. Chem., 1995, 38, 4739. CrossRef
10. B. Nguyen, M. P. Lee, D. Hamelberg, A. Joubert, C. Bailly, R. Brun, S. Neidle, and W. D. Wilson, J. Am. Chem. Soc., 2002, 124, 13680. CrossRef
11. C. M. Nunn and S. Neidle, J. Med. Chem., 1995, 38, 2317. CrossRef
12. G. M. Morris, D. S. Goodsell, R. S. Halliday, R. Huey, W. E. Hart, R. K. Belew, and A. J. Olson, J. Comput. Chem., 1998, 19, 1639. CrossRef
13. H. F. Ridley, R. G. Spickett, and G. M. Timmis, J. Heterocycl. Chem., 1965, 2, 453. CrossRef
14. N. S. Zefirov, V. V. Zhdankin, Y. V. Dankov, V. D. Sorokin, V. N. Semerikov, and A. S. Sozmin, Tetrahedron Lett., 1986, 27, 3971. CrossRef
15. L. M. Green and J. L. Reade, J. Immunol., 1984, 70, 257.
16. F. Barcelo, M. Ortiz-Lombardia, M. Martorell, M. Oliver, C. Mendez, J. A. Salas, and J. Portugal, Biochem., 2010, 49, 10543. CrossRef
17. X. F. Fei, B. X. Wang, T. J. Li, S. Tashiro, M. Minami, D. J. Xing, and T. Ikejima, Cancer Sci., 2003, 94, 92. CrossRef
18. H. Zhang, X. Feng, W. Liu, X. Jiang, W. Shan, C. Huang, H. Yi, B. Zhu, W. Zhou, L. Wang, C. Liu, L. Zhang, W. Jia, W. Huang, G. Li, J. Shi, S. Wanggou, K. Yao, and C. Ren, J. Cell. Biochem., 2011, 112, 1832. CrossRef