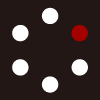
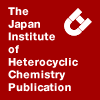
HETEROCYCLES
An International Journal for Reviews and Communications in Heterocyclic ChemistryWeb Edition ISSN: 1881-0942
Published online by The Japan Institute of Heterocyclic Chemistry
e-Journal
Full Text HTML
Received, 14th June, 2012, Accepted, 19th July, 2012, Published online, 30th July, 2012.
DOI: 10.3987/REV-12-745
■ Biological Evaluation of Pyrimidopyrimidines as Multi-Targeted Small Molecule Inhibitors and Resistance Modifying Agents
Reda Abdel-Rahman and Kamelia El-mahdy*
Department of Chemistry, Faculty of Education, Ain Shams University, El-Maqreezy St, Roxy, Heliopolis, Cairo 11711, Egypt
Abstract
This review provides detailed methods for the synthesis of pyrimidopyrimidine derivatives and their biological properties as uptake inhibitors. The main accent was put on the important findings in pyrimidopyrimidine chemistry and is useful for developing tumor growth inhibitors based on pyrimidopyrimidine.CONTENTS
1. Introduction
2. Distribution
2.1. Pyrimido[1,2-a]pyrimidines
2.2. Pyrimido[1,6-a]pyrimidines
2.3. Pyrimido[4,5-d]pyrimidines
2.4. Pyrimido[5,4-d]pyrimidines
3. Applications
4. Conclusion
5. References
1. INTRODUCTION
During the last few decades, a considerable attention has been devoted to the synthesis of pyrimidopyrimidine derivatives. Pyrimidopyrimidines possessing such diverse pharmacological and medicinal properties as inhibitors especially tumor-induced angiogenesis,1 dihydrofolate reductase agents,2 inhibition of the human epidermal growth factor receptors (EGFRs),3 antimycobacterium tuberculosis agents,4 resistance-modifying agents inhibit nucleoside transport,5 antiproliferative activity of bases thioanalogues,6 and as platelets aggregation inhibitor.7 As part of an on going program to develop new pyrimidine derivatives as biocidal agents,8-12 the present review reports a modern synthesis of pyrimidopyrimidines in view of their potential therapeutic applications especially uptake inhibitors for various diseases.
2. DISTRIBUTION
Pyrimidine compounds play an essential role in several biological processes and have very important chemical and pharmacological importance. Pyrimidopyrimidine analogues of folic acid (one of the B vitamins that is a key factor in the synthesis of nucleic acids RNA and DNA) have been screened for anti-tumor activity,13 as they exhibit observable inhibition of the human Epidermal Growth Factor Receptors (EGFRs) that occurs frequently in human cancers and is associated with aggressive tumor behavior and poor patient prognosis.3 Furthermore, pyrimidopyrimidine systems are useful as bronchodilators, vasodilators,14,15 anticancer,16 and anti-hypertensive agents.17
2.1. Pyrimido[1,2-a]pyrimidines
It is well known that the heterocyclic spiro-oxindole framework is an important structural modify in biologically relevant compounds as natural products and pharmaceuticals,18,19 e.g. surugatoxin, horsfiline, spiro-tryprostain, elacomine, gelsemine, alstonisine, and strychnofoline. Furthermore, annulation of heterocyclic ring often improves biological properties such as potency, selectivity, toxicity, and metabolic stability.20,21 Thus, an environmentally benign, efficient, and facile route was developed22 for the synthesis of novel spiro[indoline-3,2`-thiazolo[5,4-e]pyrimido[1,2-a]pyrimidin]-2(1H)-one derivatives 2 via Micheal addition of benzylidene 1 with an equimolar amount of 2-aminopyrimidine in a single step using montmorillonite KSF as inorganic solid support with few drops of DMF (Scheme 1).
Pyrimido[1,2-a]pyrimidines can be used as a co-enzymatic factor in acceleration of cellobiase activity.23 Thus, 2-amino-6-anisyl-5-cyano-4(3H)-pyrimidinone (3) was readily converted into 7-cyanopyrimido[1,2-a]pyrimidine-2,6-dione (4) when reacted with dimethyl acetylenedicarboxylate in the presence of catalytic amounts of pyridine. Treatment of compound 3 with ethyl acetoacetate in boiling DMF yielded 8-anisyl-7-cyano-4-methyl-1H-pyrimido[1,2-a]pyrimidine-2,6-dione (5). Refluxing compound 3 with ethyl 3-aryl-2-cyanopropenoate in pyridine provided the dicyanopyrimido[1,2-a]pyrimidinediones 6a,b, while the reaction of 3 with ethyl 2-acetyl-3-anisylpropenoate in DMF/NaOEt afforded 4,8-dianisyl-7-cyano-3-ethoxycarbonyl-2-methyl-1,4-dihydropyrimido[1,2-a]pyrimidin-6-one (7). When compound 3 was allowed to react with ethyl ethoxymethylenecyanoacetate in ethanol containing catalytic amounts of triethylamine, 4-amino-8-anisyl-3-ethoxycarbonyl-7-cyanopyrimido[1,2-a]pyrimidin-6-one (8) was produced.
β-Dicarbonyl reagents such as diethyl malonate, acetylacetone, and benzoylacetanilide reacted with compound 3 to give the corresponding cyanopyrimido[1,2-a]pyrimidinediones 9-11. Active methylene compounds such as a ω-cyanoacetophenone derivative gave 2-amino-8-anisyl-4-(p-bromophenyl)-7-cyanopyrimido[1,2-a]pyrimidin-6-one (12) when reacted with heterocyclic amidine 3 in acetic acid containing fused sodium acetate. On the other hand, the 7-cyano-2,4,8-triarylpyrimido[1,2-a]pyrimidin-6-ones 13a-c were obtained upon refluxing compound 3 with the appropriate enones in acetic acid-fused sodium acetate mixture.
Similar reaction occurred on treatment of 3 with cinnamaldehyde which led to the formation of 7-cyano-2,8-diarylpyrimido[1,2-a]pyrimidin-6-one (14). Also, α,β-unsaturated ketoacid reacted with the cyclic amidine 3 to furnish 7-cyano-pyrimido[1,2-a]pyrimidin-6-one 15 (Scheme 2).23
Schistosomiasis is the second most imperative tropical parasitic disease of humans after malaria especially in the developing world with an estimation that at least 200 million people, in 74 countries, are currently infected and 600 million are at risk of infection. Annual mortality due to schistosomiasis was estimated at 15,000 deaths/year and the burden of disease at 1.702 million DALYs lost per year. About 20 million people suffer several consequences from the disease and 120 million are symptomatic.24,25 Although the current strategy for morbidity control is largely based on chemotherapy, control of the snails host using molluscicides to stop transmission cycle is still considered a vital role because the use of molluscicides in the prophylactic treatment leads to the rupture of evolutionary lifecycle of the worm via destructing its intermediate host.25
El-mahdy12 reported convenient methods for the synthetic new pyrimidopyrimidines as molluscicidal activities against Biomophalaria alexandrina snails. Thus, formylation of 2-aminopyrimidine (3) using formic acid afforded 2-formamidopyrimidine (16) which on treatment with malononitrile and/or ethyl cyanoacetate in basic medium gave 6-amino-2-(p-methoxyphenyl)-4-oxo-4H-pyrimido[1,2-a]pyrimidine 3,7-dicarbonitrile (17) and 4-amino-1-formyl-8-(p-methoxyphenyl)-2,6-dioxo-2,6-dihydro-1H-pyrimido-[1,2-a]pyrimidine-7-carbonitrile (18), respectively. While, interaction of 3 with (p-chlorobenzylidene)malononitrile and/or 3-(p-chlorophenyl)-1-(m-nitrophenyl)-2-propen-1-one yielded 8-amino-6-(p-chlorophenyl)-2-(p-methoxyphenyl)-4-oxo-4H-pyrimido[1,2-a]pyrimidine-3,7-dicarbonitrile (19) and 6-(p-chlorophenyl)-2-(p-methoxyphenyl)-8-(m-nitrophenyl)-4-oxo-4H-pyrimido[1,2-a]pyrimidine-3-carbo-nitrile (20), respectively.
On the other hand, when compound (3) was treated with acrylonitrile in a pyridine-water mixture gave 4,6-dioxo-8-(p-methoxyphenyl)-1,3,4,6-tetrahydro-2H-pyrimido[1,2-a]pyrimidine-7-carbonitrile (21) (Scheme 3).
2.2. Pyrimido[1,6-a]pyrimidies
An easy and efficient route for the synthesis of some new pyrimido[1,6-a]pyrimidine derivatives was described26 through the reaction of sodium salts of formyl ketones (23-25) with 6-aminothiouracil (22) to give the cyclocondensed pyrimido[1,6-a]pyrimidines (26), 4-methyl-6-thioxo-6,7-dihydro-8H-pyrimido[1,6-a]pyrimidin-8-one (27) and 4-phenyl-6-thioxo-6,7-dihydro-8H-pyrimido[1,6-a]pyrimidin-8-one (28) respectively (Scheme 4). The novel systems obtained exhibited a moderate activity against different types of microorganisms Grams positive bacteria as Staphylococus aureus, Bacillus subtilis and Grams negative bacteria as Escherichia coli and Pseudomonas aeruginosa and a fungus as Candida albicans.26
The pyrimidines and purine ring systems undoubtedly belong to the most ubiquitous heterocycles in nature, as they represent the main structure of many biologically significant compounds, including nucleosides and nucleotides as drugs.27 Thus, new developed a novel versatile and convenient approach to 2,9-disubstituted-5,6-dihydro-4H,8H-pyrimido[1,2,3-cd]purine-8,10(9H)-diones (32) which can be envisaged as xanthine derivatives to which a third ring is attached bridging the N3- and N9-positions.28
Thus, condensation of 29 with aldehyde in ethanol produced the imino-derivative (30) which on heterocyclization using DMF/K2CO3 followed by 1,3-dibromopropane afforded 9-(arylideneamino)-7-butyl-1,2,3,4-tetrahydropyrimido[1,6-a]pyrimidine-6,8-dione (31). Refluxing compound (31) with thionyl chloride as reagent and solvent29 to give the tricyclic product (32) in good yield. On the other hand, compounds (32) were obtained from treatment of 29 with RCO2H/MeOH in the presence of EDC [N`-(3-dimethylaminopropyl)-N-ethylcarbodiimide hydrochloride] to produce the amide (33). Warming of compound (33) with DMF/K2CO3 and 1,3-dibromopropane yielded 7,9-disubstituted 1,3,4,6,7,8-hexahydro-2H-pyrimido[1,6-a]pyrimidine-6,8-diones (34). Refluxing of 34 with HMDS [1,1,1,3,3,3-hexamethyldisilazane] afforded the target compounds (32) (Scheme 5).30
2.3. Pyrimido[4,5-d]pyrimidines
The most promising methods for the synthesis of pyrimido[4,5-d]pyrimidine are multistep synthesis starting from 1,3-disubstituted 5-cyanouracils31 or from polymer bound 2-(alkylsulfanyl)-4-aminopyrimidine-5-carbonitrile.32 However, the synthetic strategy commences from Biginelli compound, which led to the construction of requisite pyrimido[4,5-d]pyrimidine nucleus. Thus, the synthesis of precursor ethyl 4-(2-chlorophenyl)-6-ethoxy-2-oxo-1,2,3,4-tetrahydropyrimidine-5-carboxylate (35) was achieved as per standard Biginelli condensation reaction. This key compound was stirred in an equimolar quantity with freshly prepared 4-chlorophenyldiazonium chloride in concentrated hydrochloric acid, under diazotization conditions to give compound (36). Subsequently, this diazotized derivative (36) was allowed to reflux with various arylthioureas (37a–k) in EtOH affording 4-(2-chlorophenyl)-2-oxo-3-(4-chlorophenylazo)-6-(N-arylthioureido)-5-ethylcarboxylato-1,2,3,4-tetrahydropyrimidine (38a–k) in 70–80% yield. The corresponding target compounds (39a–k) were obtained by intramolecular cyclization of 38a–k under stirring in a solution of sodium methoxide and MeOH in an excellent yields (Scheme 6).33
All the reported compounds exhibited remarkable in vitro activity against the tested bacterial and fungal strains compared to reference drugs within a MIC range of 2–57 µg/mL. A systematic perusal of the data reveals that in comparison to antifungal behaviour, manifested by these compounds, their antibacterial effect is observed to be highly significant and pronounced. Particularly, Gram-positive bacteria seem to be more sensitive towards the newly synthesized pyrimido[4,5-d]pyrimidine analogues. However, these compounds have been found to show the least to moderate activity on the growth of A. niger with MIC range of 14–57 µg/mL. In general antimicrobial activity of the tested compounds follows the pattern: S. aureus > B. subtilis > E. coli > P. diminuta > C. albicans > A. niger.
QSAR analysis studies of the newly obtained compounds were undertaken using the linear free energy relationship (LFER) model of Hansch and Fujita,34 and revealed that the antimicrobial activities of these synthesized derivatives against the test microorganisms are mainly governed by molar refractivity, a polarizeability parameter. The proper substitution of the group with high polarizability at N-6 position probably improves the potency of these derivatives as antibacterial and antifungal agents.33
In order to better understand if this conformation is a pharmacophore requirement, starting 40, basic congeners (41) were also synthesized and tested.6 Synthesis of intermediate 5-imino-1,3,6-triphenyl-5,8-dihydropyrimido[4,5-d]pyrimidine-2,4,7-(1H,3H,6H)-trithione (40) was started by reacting malononitrile and phenylisothiocyanate in anhydrous DMF in the presence of sodium hydride. Alkylation of 40 using 4-chloroalkyldialkyl- or cyclo-amines in the presence of diverse bases (NaHCO3, Triton, sodium hydroxide, K2CO3, triethylamine and or 2 equivalents of chloroamine) afforded the targets (41) (Scheme 7). To better understand pharmacophore requirements, a forth series of conformationally constrained analogues 41 were also prepared to be active in the low micromolar concentration range (IC50; 3.3-4.3 μM). In general bicyclic 41 up to 10-fold more potent than monocyclic counterparts against solid tumor-derived cell lines.
Antimetabolite drugs which act by inhibition of the novel purine and pyrimidine biosynthesis play an important role in the chemotherapy of cancer,35 and the classical thymidate synthesis (TS) inhibitor raltitrexed. Tumour resistance to antimetabolites may arise via a number of mechanisms one of which involves the salvage of extracellular nucleosides and nucleobaser via carrier-medicated plasma membrane transport, resulting in repletion of nucleotide pools.36
Electron deficient nature of pyrimidine ring facilitates the synthesis of large number of pyrimidine derivatives through nucleophilic aromatic substitution of suitable leaving groups. The halogen atoms have been especially useful in this regard.37,38 Thus, heteroannelation of ethyl 4-chloro-2-(4-chlorophenyl)-6-methylpyrimidine-5-carboxylate (42) via a nucleophilic attack of guanidinum carbonate and/or thiourea in refluxing EtOH/TEA afforded39 the corresponding pyrimido[4,5-d]pyrimidinone (43 and 44) respectively. Fusion of compound 42 with urea did not give compound 45 but yielded ethyl 4-amino-2-(4-chlorophenyl)-6-methylpyrimidine-5-carboxylate (46) (Scheme 8). Compound 44 had anti-fertility.
Recently, pyrimido[4,5-d]pyrimidine derivatives used as common drugs as RO4383596,40 RO4396686,1 and negligible enzyme activity towards dihydrofolate reductase (DHFR),2 as well as antiproliferative agents of basic thioanalogues of merbarone.6
Most of the antitumor agents currently used in the treatment of human malignancies are targeted to topoisomerase II (topo II). The function of this enzyme is that of relaxing a supercoiled DNA by allowing double standed DNA (ds DNA) chains to pass one through another following a cleavage generating blunted DNA ends.41,42 Compounds interfering with the catalytic activity of the enzyme (Merbarone)43 is expected to be active against both fast and slow growing cancer.44,45 Merbarone is particularly interesting for the following reasons: i) it acts primarily by blocking topo II-mediated DNA cleavage without intercalating into DNA or binding to the minor groove ii) it inhibits in vitro topo II decatenation and relaxation activities and causes G2/M blockade, iii) it induces apoptosis by a mechanism distinct from that reported for the topo II poisons etoposide and doxorubicin.46
Upon these facts, the present review reports the synthesis and chemistry of pyrimido[4,5-d]pyrimidine as RO4383596 in view of their pharmacological and medicinal activities. Thus, (±)-1-(anti-3-hydroxy-cyclopentyl-3-(4-methoxyphenyl)-7-phenylamino-3,4-dihydro-1H-pyrimido[4,5-d]pyrimidin-2-one(RO4384596) (52) is a potent and selective inhibitor of the pro-angiogenic receptor tyrosine Kinases KDR, FGFR and PDGFR. Compound (52) was synthesized40 via the interaction between ethyl 4-chloro-2-(methylthio)pyrimidine-5-carboxylate (47) with the known racemic TBS-protected aminocyclopentanol (48) followed by full heterocyclization reactions (Scheme 9). The target 52 has an excellent pharmacokinetic profile and is highly efficacious in rodent models of angiogenesis upon oral administration. The kinase selectively profile of RO4383596 data were reported in Table 1, which showed that this drug had a high plasma exposure and bioavailability upon oral administration. In vivo it was efficacious at doses as low as 25 mg/kg.
In program to design and develop mechanism-based compounds active as substrates and inhibitors of dihydrofolate reductase (DHFR), Gebauer et al.,2 reported the synthesis and physical properties of 6-methyl- (57), 8-methyl- (58a) and 8-ethyl- (58b) derivatives of the parent 2-amino-4,8-dihydropyrimido[4,5-d]pyrimidin-4(3H)-one (54). Thus, treatment of 2,6-diaminopyrimidin-4(3H)-one (53) with formamide yielded 54 while formylation of 53 using POCl3/DMF produce the 5-formylpyrimidine (55). Condensation of 55 with methylamine followed by refluxing with acetic acid afforded 2-amino-6-methylpyrimido[4,5-d]pyrimidin-4(6H)-one (57).
Alkylation of 57 using various alkylhalides in warming methanol led to the formation of 8-alkyl-2-amino-pyrimido[4,5-d]pyrimidin-4(8H)-one (58a-c) (Scheme 10).
2-Amino-4,8-dihydropyrimido[4,5-d]pyrimidin-4(3H)-one (54) has a basic pKa of 2.8 and is slightly more basic than pterin (A). Thus, protonation of 57, 58a, 58b and 54 gave a charge delocalization occurs in the cations 59, 60, and 61. Also, covalent hydration of 60 has occurred across the 7-8 bond.47
Enzymic and chemical tests of the new series of N6- and N8- alkylated derivatives of compound 54 have supported that design principle that such compounds should have enhanced basicity allowing them to be substrates of DHFR at neutral pH compounds 58a and 58b, have optimized yield (1-8%) were still low due to product decomposition from ring opening.
The basicities of 57, 58a (pKa ca 5.5) are similar to those of 8-alkylpterins (B) this is an essential elemental of the design to promote binding to DHFR in their protonated form. Enzyme kinetics of 57, 58a and 58b with chicken DHFR confirmed that predictions that they are substrates with apparent Km values of 3.8, 0.08 and 0.65 mM, and apparent νmax values of 0.47, 2.27 and 0.30 n mol L-1 min-1 (Chart 1).
2.4. Pyrimido[5,4-d]pyrimidines
Infection with Mycobacterium tuberculosis affects much of the world population, despite the fact that drugs for treating tuberculosis (TB) were available for over half a century. Each year, it is estimated that 9.2 millions new cases appear, of which many lead to death.48-51 Thus, a novel class of antimycobacterium tuberculosis agents as substituted pyrimido[5,4-d]pyrimidines (65 and 66) have been synthesized from the interaction of 6-cyanopurines (64) with ammonia, primary amines, and acid hydrazides.52,53 The activity of compounds 65 and 66 against M. tuberculosis strain H37Rv depends on the substituents R, R` (Scheme 11). Furthermore, structural modifications of the identified hits are in progress in order to enhance that efficacy of this new structural class of compounds active against M. tuberculosis.4
Coplanar π-conjugated polymers generally have a strong tendency to self-assembled, π-conjugated polymers often show unique electrical and optical properties.54-57 Thus, Fukumoto et al.,58 prepared new π-conjugated pyrimidopyrimidine (polymers with two different alkylamino groups at C4 and C8 positions, PPympym (4-NH-n-C8H17-8-NHR) (R = n-C4H9, n-C6H13, n-C12H25 & n-C16H33) by dehalogenation of 67 and polycondensation using nickel zerovalent complex in high yield (Scheme 12).
Uv-vis spectra of these polymers in CHCl3 showed a π-π* absorption peak at about 450 nm, and the peak position shifted by 30 nm to a longer wavelength in film. Also, the polymers showed photoluminescence both in solution and in film. Cast films of the polymers were electrochemically reduced at about -2.3 V vs. Ag+/Ag''. Powder XRD data indicate that a pair of two different alkyl side-chains aggregates to take an end-to-end packing mode. Compound 70 was used as resistance-modifying agent.
Dipyridamole binds avidly to α1-acid glycoprotein (AGP1, orosomueoid) an acute phase protein the plasma levels of which may be elevated in cancer patients, and as a consequence, AGP reduces the effective concentration of the drug below those required to inhibit salvage pathways.59,60
Thus, a part of an ongoing program to develop novel pyrimidopyrimidines as resistance modifiers in cancer chemotherapy, Barlow et al.,5 synthesized some targets and studied designs to elucidate structure-activity relationships for binding to AGP.
The required pyrimidopyrimidines (73) were initially prepared analogously to dipyridamole starting from tetrachloropyrimidopyrimidine (67).61
Treatment of 67 with 4 equivalents of the required benzylamine at room temperature to give 71, followed by 4 equivalents of the appropriate amine or alkoxide at elevated temperature (reactivity order: 4 ≈ 8> 2 ≈ 6) afforded the target compounds. However, while suitable for the preparation of analogues bearing N-methylbenzylamino substituents at the 4/8 positions (72, R1 = Me), analogous reactions with benzylamines afforded low product yields and necessitated extensive product purification. This was attributed to side reactions arising from deprotonation of the relatively acidic 4,8-dibenzylamino functionality (71, R1 = H) under the basic conditions and elevated temperatures employed in the second displacement step. Protection of the vulnerable benzylamino NH substituent was achieved with the 3,4-dimethoxybenzyl (dmb) protecting group. This was introduced by reacting the appropriate benzylamine with 3,4-dimethoxybenzaldehyde in dry EtOH, and reducing the resultant imine with sodium borohydride in MeOH. Treatment of 67 with the requisite N-dmb-protected benzylamine at room temperature, gave the 4,8-disubstituted derivatives (71, R1 = dmb) in excellent yield. Substitution at the 2,6-positions by the required amine or mono-triisopropylsilyl protected diol was then achieved without incident to furnish 72. Where necessary, removal of the triisopropylsilyl groups at the 2,6-positions was effected with TBAF, and the 4,8-dmb protecting groups were finally removed on treatment with TFA or DDQ at room temperature, affording the target pyrimidopyrimidines (73) in good overall yield.
Biological evaluation of potent 4,8-dibenzylaminopyrimidopyrimidines (72 and 73) nucleoside transport inhibitors, with reduced binding to α1-acid glycoprotein was reported in the Table 2.
3. APPLICATIONS
Investigation of the compounds BIBU 1361, BIBU 1476, and BIBX 1382 gave a good correlation for tolerability/lethality between C. elegans and rodents. Also, application of this model allowed the determination of a rank order potency for toxicity among compounds.63
Dipyridamole, pyrimidopyrimidine derivative is used clinically as coronary vasolidlator and a platelet aggregation inhibitor.7 Also, dipyridamole inhibits both es and ei nucleoside transporters.64,65 It has been reported that dipyridamole inhibits [3H] NBMPR binding to membranes or cells with K1 values of 10-9-10-7 M.66-68 A high dosage of dipyridamole is required for effective inhibition of nucleoside transport in vivo.69 Thus, one of its principal pharmacological mechanisms is dipyridamole uptake inhibition and also has other pharmacological actions including phosphdiesterase inhibition.70,71
On the other hand, dipyridamole and its analogues are well known inhibitor of both hENT1 and hENT2 nucleoside transporter.72,73
Similarly, RO4396686; a racemic pyrimidopyrimidine that selectively inhibits three key pro-angiogenic RTKs KDR, FGFR, and PDGFR.74-76
In a mouse corneal neovascularization assay, this compound inhibited77 VEGF—induced angiogenesis. Tested in a H460a xenograft tumor model this agent effected significant tumor growth inhibition at doses as low as 50mg/kg.1,77
Finally, poly(pyrimido[5,4-d]pyrimidine-2,6-diyl)s were synthesized and used as new polymers similar to ppympym (4,8-NHR) as resistance-modifying agents.58,78
4. CONCLUSION
Various kinds of pyrimidopyrimidines uptake inhibitors are currently available and have been used to uptake sites in tissues or organs. Also, pyrimidopyrimidines uptake inhibitors are able to enhance the protective effects of endogenous released at sites of tissue or organ damage. Finally, pyrimidopyrimidines, uptake inhibitors are expected to exhibit protective and ameliorating effects against various clinical diseases. The therapeutic importance of pyrimidines and annulated pyrimidine derivatives are antibacterial,79 antitumor,80 anti-inflammatory,81 antifungal,82 and cardiovascular agents.83
References
1. L. A. Mc Dermott, B. Higgins, M. Simcox, K. C. Luk, T. Nevins, K. Kolensky, M. Smith, H. Yang, J. K. Li, Y. Chen, J. Ke, N. Mallalieu, T. Egan, S. Kolis, A. Railkar, L. Gerber, J. Liu, F. Konzelmann, Z. Zhang, T. Flynn, O. Morales, and Y. Chen, Bioorg. Med. Chem. Lett., 2006, 16, 1950. CrossRef
2. M. G. Gebauer, C. McKinaly, and J. E. Gready, Eur. J. Med. Chem., 2003, 38, 719. CrossRef
3. F. F. Solca, A. Baum, k. Lang, D. Elke, H. Georg, Z. Karl-Hein, F. Himmelsbach, and C. A. Jacques, J. Pharmacol. Exp. Therap., 2004, 311, 502. CrossRef
4. A. H. Bacelar, M. C. Carvalho, and M. F. Proenca, Eur. J. Med. Chem., 2010, 45, 3234. CrossRef
5. H. C. Barlow, K. J. Bowman, N. J. Curtin, A. H. Calvert, B. T. Golding, B. Huang, P. J. Loughlin, D. R. Newell, P. G. Smith, and R. J. Griffin, Bioorg. Med. Chem. Lett., 2000, 10, 58. CrossRef
6. A. Ranise, A. Spallarossa, S. Schenone, O. Bruno, F. Bondavalli, A. Pani, M. E. Marongiu, V. Mascia, P. Colla, C. La, and R. Loddo, Bioorg. Med. Chem. Lett., 2003, 11, 2575. CrossRef
7. E. L. De Schryver, A. Algra, and J. Van Gijin, Stroke, 2003, 34, 2072. CrossRef
8. M. Seada, R. M. Abdel-Rahman, and F. Hanafy, J. Indian Chem. Soc., 1992, 69, 882.
9. M. Seada, R. M. Abdel-Rahman, and M. Abdel-Magid, Indian J. Heterocycl. Chem., 1993, 3, 9.
10. M. Seada, R. M. Abdel-Rahman, M. El-Behairy, and F. Hanafy, Asian J. Chem., 1992, 4, 604.
11. S. A. Abdel-Aziz, H. A. Allimony, H. M. El-Shaaer, U. F. Ali, and R. M. Abdel-Rahman, Phosphorous, Sulfur and Silicon and Related Elements, 1996, 113, 67. CrossRef
12. M. Abdel-Megid, M. Abdel-Hamid, K. M. El-Mahdy, M. Seada, and M. M. Elsayed, J. Chem. and Chemical Engineering, 2010, 4, 41.
13. T. J. Delia, M. Baumann, and A. Bunker, Heterocycles, 1993, 35, 1397. CrossRef
14. E. C. Taylor, R. J. Knopf, R. F. Meyer, A. Holmes, and M. L. Hoefle, J. Am. Chem. Soc., 1960, 82, 5711. CrossRef
15. J. D. Figueroa Villar, C. L. Carneiro, and E. R. Cruz, Heterocycles, 1992, 34, 891. CrossRef
16. W. J. Coates, Eur. Patent 1990, 351058 (Chem. Abstr., 1990, 113, 40711).
17. N. Kitamura and A. Onishi, Eur. Patent 1984, 163599 (Chem. Abstr., 1984, 104, 186439).
18. M. Y. Chang, C. L. Pai, and Y. H. Kung, Tetrahedron Lett., 2005, 46, 8463. CrossRef
19. S. P. Baran and R. M. Richter, J. Am. Chem. Soc., 2005, 127, 15394. CrossRef
20. H. J. Knolker and K. R. Raddy, Chem. Rev., 2002, 102, 4303. CrossRef
21. D. A. Williams and T. L. Lemke, Foye′s Principles of Medicinal Chem. 5th Edn., Lippincott Williams & Wikins, pp. 924-951, 2002.
22. A. Dandia, G. Sharma, R. Singh, and A. Laxkar, ARKIVOC, 2009, Xiv, 100.
23. M. Abdel-Megid, Pharmazie, 2000, 55, 263.
24. WHO, World Health Report, 2004.
25. WHO, TDR/Schistosomiasis, 2002.
26. A. M. Hussein, J. Saudi Chem. Soc., 2010, 14, 61. CrossRef
27. H. J. Roth and H. Fenner, Struktur Bioreaktivitiat-Wirkungs bezogen Eigenschaften, Deutscher Apotheker: Stuttgart, 2000.
28. S. Weyler, A. M. Hayallah, and C. E. Muller, Tetrahedron, 2003, 59, 47. CrossRef
29. K. Senga, K. Shimizu, and S. Nishigaki, Chem. Pharm. Bull., 1977, 33, 227.
30. M. Eckhardt, E. Langkopf, M. Mark, M. Tadayyon, L. Thomas, H. Nar, W. Pfrengle, B. Guth, R. Lotz, P. Sieger, H. Fuchs, and F. Hummelsbach, J. Med. Chem., 2007, 50, 6450. CrossRef
31. (a) K. Hirota, Y. Kitade, H. Sajiki, and Y. Maki, Synthesis, 1984, 589; CrossRef (b) K. Hirota, Y. Kitade, H. Sajiki, and Y. Maki, J. Chem. Soc., Perkin Trans 1, 1990, 123. CrossRef
32. S. W. Srivastava, W. Haq, and P. M. S. Chauhan, Bioorg. Med. Chem. Lett., 1999, 9, 965. CrossRef
33. P. Sharma, N. Rane, and V. K. Gurram, Bioorg. Med. Chem. Lett., 2004, 14, 4185. CrossRef
34. C. Hansch and T. Fujita, J. Am. Chem. Soc., 1964, 86, 1616. CrossRef
35. A. L. Jackman and A. H. Calvert, Annals of Oncology, 1995, 6, 871.
36. J. A. Belt, N. M. Marina, D. A. Phelps, and C. R. Crawford, Advan. Enzyme Regul., 1993, 33, 235. CrossRef
37. S. El-Bahaie, A. El-Deeb, and M. G. Assy, Pharmazie, 1991, 46, 26.
38. S. El-Bahaie, M. G. Assy, and A. F. Heikal, J. Indian Chem. Soc., 1990, 67, 327.
39. H. A. Saad, H. Y. Moustafa, M. G. Assy, and M. A. Sayed, Bull. Korean Chem., 2001, 22, 311.
40. L. A. Mc Dermott, M. Simcox, B. Higgins, T. Nevins, K. Kolinsky, M. Smith, H. Yang, J. K. Li, Y. Chen, J. Ke, N. Mallalieu, T. Egan, S. Kolis, A. Raifker, L. Gerber, and K. C. Luk, Bioorg. Med. Chem. Lett., 2005, 13, 4835. CrossRef
41. A. H. Andersen, J. Q. Svejstrup, and O. Westergaard, Adv. Pharmacol., 1994, 29A, 83. CrossRef
42. P. M. Watt and I. D. Hickson, Biochem. J., 1994, 303, 681.
43. F. H. Drake, G. A. Hofmann, S. M. Mong, J. O. Bartus, R. P. Hertzberg R. K. Johnson, M. R. Mattern, and C. K. Mirabell, Cancer Res., 1989, 49, 2578.
44. J. L. Nitiss, Y. X. Liu, and Y. Hsiung, Cancer Res., 1993, 53, 89.
45. C. L. Fattman, W. P. Allan, B. B. Hasinoff, and J. C. Yalowich, Biochem. Pharmacol., 1996, 52, 635. CrossRef
46. Y. Y. Mo and W. T. Beck, Mol. Pharamacol., 1999, 55, 216.
47. P. Wormell and J. E. Gready, Chem. Phys., 1994, 179, 55. CrossRef
48. C. Dye, Nat. Rev. Microbiol., 2009, 7, 81. CrossRef
49. A. M. Cinsberg, Semin Respir. Crit. Care Med., 2008, 29, 552. CrossRef
50. H. D. Showalter and W. Denny, Tuberculosis, 2008, 88, S3. CrossRef
51. H. Tomioke, Y. Tatano, K. Yasumoto, and T. Shimizu, Expert Rev. Respir. Med., 2008, 2, 455. CrossRef
52. M. A. Carvalho, S. Esperance, T. Esteves, and M. F. Proenca, Eur. J. Org. Chem., 2007, 1324. CrossRef
53. A. Ribeiro, M. A. Carvalho, and M. F. Proenca, Eur. J. Org. Chem., 2009, 4867. CrossRef
54. I. McCulloch, M. Heeney, C. Bailey, K. Genevicicus, J. Mac. Donald, M. Shkunov, D. Sparrowe, S. Tierney, R. Wagner, W. Zhang, M. L.Chabinyc, R. J. Kline, M. D. McGehee, and M. F. Toney, Nat. Mater., 2006, 5, 328. CrossRef
55. M. L. Chabinyc, M. F. Toney, R. J. Kline, I. McCulloch, and M. Heeney, J. Am. Chem. Soc., 2007, 129, 3226. CrossRef
56. H. Sirringhaus, P. J. Brown, R. H. Friend, M. M. Nielsen, K. Bechhgaard, B. M. W. Langeveld-Voss, A. J. H. Spiering, R. A. J. Janssen, E. W. Meijer, P. Herwig, and D. M. D. Leeeuw, Nature (Lond), 1999, 401, 685. CrossRef
57. T. Morikita, I. Yamaguchi, and T. Yamamoto, Adv. Mater., 2001, 13, 1862. CrossRef
58. H. Fukumoto, S. Takasuki, B. Lin Lee, and T. Yamamoto, Synthetic Metals, 2009, 159, 900. CrossRef
59. N. J. Curtin, D. R. Newell, and A. L. Harris, Biochem. Pharmacol., 1989, 38, 3281. CrossRef
60. G. T. Budd, A. Jayaraj, D. Grabowski, D. Adelstien, L. Bauer, J. Boyett, R. Bukowski, S. Murthy, and J. Weick, Cancer Res., 1990, 50, 7206.
61. K. G. Thomae, Ger. Pat. 1963, 1151806 (Chem. Abstr., 1964, 60, 2974a).
62. N. J. Curtin, K. J. Bowman, R. N. Tuner, B. Huang, P. J. Loughlin, A. H. Calvert, B. T. Golding, R. J. Griffin, and D. R. Newell, Br. J. Cancer, 1999, 80, 1738. CrossRef
63. M. Dengg and J. C. A. van Meel, J. Pharm. Toxiocological. Metheods, 2004, 50, 209. CrossRef
64. T. V. Dunwiddie, Neuroscience, 2000, 95, 81. CrossRef
65. J. L. Ward, A. Sherali, Z. P. Mo, and C. M. Tse, J. Biol. Chem., 2000, 275, 8375. CrossRef
66. P. J. Marangos, J. Patel, R. Clark-Rosemberg, and A. M. Martino, J. Neurochem., 1987, 39, 184. CrossRef
67. A. Verma and P. J. Marangos, Life Sci., 1985, 36, 283. CrossRef
68. J. P. Heaton and A. S. Clanachan, Biochem. Pharamacol., 1987, 36, 1275. CrossRef
69. H. Van Belle and P. A. Janssen, Nucleoside Nucleotides, 1991, 10, 975. CrossRef
70. L. A. Harker and R. A. Kadatz, Thromb. Res., 1983, 4, 39. CrossRef
71. J. Dogne, X. de Leval, P. Benoit, J. Delarge, B. Mascrecl, and J. David, Cur. Med. Chem., 2002, 9, 577. CrossRef
72. W. Lin and J. K. Buolamwini, J. Med. Chem., 2007, 50, 3906. CrossRef
73. A. Gupta and J. K. Buolamwini, Bioorg. Med. Chem. Lett., 2009, 19, 917. CrossRef
74. For reviews about receptors and signaling pathways involved in tumor angiogenesis, see: (a) R. Bicknell and A. L. Harris, Annu. Rev. Pharmacol. Toxicol., 2004, 44, 219; CrossRef (b) S. V. Lutsenko, S. M. Kiselev, and S. L. Severin, Biochem. (Moscow), 2003, 68, 286; CrossRef (c) G. D. Yancopoulos, S. Davis, N. W. Gale, J. S. Rudge, S. Wiengand, and J. Holash, Nature, 2000, 407, 242. CrossRef
75. For reviews on angiogenessts inhibitors and the clinical aspects of anti-angiogenic therapy, see: (a) J. Rhee and P. M. Hoff, Expert Opin Pharmacother, 2005, 6, 1701; CrossRef (b) H. M. W. Verheul and H. M. Pinedo, Expert Opin Emerg. Drugs, 2005, 10, 403; CrossRef (c) F. A. Scoppaticci, Expert Opin. Investing Drugs, 2003, 12, 923. CrossRef
76. For some reviews, see: (a) R. Mazitscheck and A. Giannis, Opin. Ther. Targets, 2003, 7, 215; CrossRef (b) F. Magntti and M. Botta, Curr Pharm. Des., 2003, 9, 567; CrossRef (c) S. J. Boyer, Curr. Top. Med. Chem., 2002, 2, 973. CrossRef
77. W. L. Wu, D. A. Burnett, M. Domalski, W. J. GreenLee, and C. Li, J. Med. Chem., 2007, 50, 5550. CrossRef
78. E. G. T. Yamamoto, S. I. Otsuka, K. Namekawa, H. Fukumoto, I. Yamaguchi, T. Fukuda, N. Asakawa, T. Yamanobe, T. Shiono, and Z. Cai, Polymer, 2006, 47, 6038. CrossRef
79. J. Metzner and D. H. Hauses, Pharmazie, 1979, 34, 578.
80. M. Suiko and M. Mackawa, Agric. Biol. Chem., 1977, 41, 2047. CrossRef
81. D. Doria, C. Passarotti, R. Sala, R. Magrini, P. Sberze, M. Tibolla, A. Buttinnoi, M. Ferrara, and L. Tremolada, Farmaco, Ed. Sci. 1986, 41, 737.
82. Y. Tokunaga, K. Kahu, Y. Kojima, J. Maeno, N. Sawai, and Y. Saso, Eur. Pat. Ep 1987, 24268 (Chem. Abstr., 1988, 108, 75424).
83. T. Mishina, N. Tsuda, A. Inui, and Y. Miura, Jap. Pat. JP 1987, 62169793 (Chem. Abstr., 1988, 108, 5610).