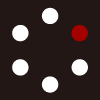
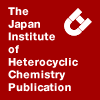
HETEROCYCLES
An International Journal for Reviews and Communications in Heterocyclic ChemistryWeb Edition ISSN: 1881-0942
Published online by The Japan Institute of Heterocyclic Chemistry
e-Journal
Full Text HTML
Received, 18th June, 2012, Accepted, 6th July, 2012, Published online, 11th July, 2012.
DOI: 10.3987/COM-12-12526
■ Synthesis of Procyanidins C2 and C1 Using Lewis Acid Mediated Equimolar Condensation
Yukiko Oizumi, Miyuki Katoh, Yasunao Hattori, Kazuya Toda, Koichiro Kawaguchi, Hiroshi Fujii, and Hidefumi Makabe*
Sciences of Functional Foods, Graduate School of Agriculture, Shinshu University, 8304 Minamiminowa-mura, Kamiina-gun, Nagano 399-4598, Japan
Abstract
Synthesis of procyanidins C2 and C1 was achieved via a stereoselective intermolecular condensation of equimolar amount of dimeric catechin or epicatechin nucleophile and monomeric catechin or epicatechin electrophile using Lewis acid. In the case of synthesis of procyanidin C2, AgBF4 and AgOTf afforded condensed product in excellent yield. As to the synthesis of procyanidin C1, Yb(OTf)3 was effective for equimolar condensation.INTRODUCTION
Proanthocyanidins are known as condensed or non-hydrolysable tannins.1 These tannins are widely found in the vegetables, fruits and the bark of trees.2 Proanthocyanidins have been reported to exhibit strong free-radical scavenging and antioxidative activities.3 Many biological activities such as antitumor,4 antiviral,5 anti-inflammatory,6 and inhibition of DNA polymerase were reported.7 Thus proanthocyanidins are increasingly recognized as possessing health beneficial effects for humans. Tannin extracts from the nature give various types of polyphenols. Because their identification as well as purification is extremely difficult, further investigation of biological activities, i. e. mechanism of action remains unsolved. In these days, in order to obtain procyanidin oligomers in pure state, synthetic efforts have been devoted.8 As to the synthesis of catechin and/or epicatechin trimers, several synthetic studies were reported. The typical synthetic methods are as follows. The first example is pioneering work of the synthesis of procyanidins reported by Saito et al.9 This method requires to use nucleophilic partner in large excess (3.0 to 4.5 eq.) for condensation to prevent further oligomerization. The disadvantage of this procedure is that excess nucleophilic partner needs to be removed after condensation. The next one is use of C-8 bromide derivative to prevent forming further oligomeration.10 Quite recent example is using C-8 bromide and C-8 metallated nucleophile for the selective synthesis of C-4 and C-8 bound catechin trimers.11 So far, little attention has been paid to the screening of Lewis acids for equimolar condensation to construct the skeleton of procyanidin trimers. We have already reported a stereoselective synthesis of catechin trimer derivative using equimolar condensation.12 However, synthesis of epicatechin trimer using equimolar condensation has not been reported yet. In this article, we wish to report total synthesis of procyanidin C2 (1) and the recent progress of the synthesis of procyanidin C1 (2) via equimolar condensation between dimeric catechin or epicatechin nucleophile and monomeric catechin or epicatechin electrophile (Figure 1).
RESULTS AND DISCUSSION
We have investigated various Lewis acids including Ag, Cu, and Yb for equimolar condensation between dimeric catechin nucleophile 3 and monomeric catechin electrophile 4. Among these Lewis acids, we found that silver Lewis acids such as AgBF4 and AgOTf gave condensed product in excellent yield Scheme 1).12
The condensed product 5 was obtained in high yield,9 we next pursued to establish total synthesis of procyanidin C2 (1). Hydrolysis of the acetate of 5 using NaOMe under reflux did not furnish 6 although this reaction worked well in the case of procyanidin B1-B4.13 Thus diol 5 was once acetylated using Ac2O to give triacetate and subsequent reduction with DIBALH afforded 6 in 45% yield through 2 steps.9 However, the yield of transformation from 5 to 6 was not high enough, we tried the Kozikowski’s method using n-Bu4NOH to afford 6 in 66% yield.14 The 1H and 13C NMR spectral data of 6 were identical with those of the reported values.9 The 3,4-cis trimer could not be detected. This result indicated that electrophile 4 reacted with nucleophile 3 at C-4 position in a stereoselective manner to afford 3,4-trans trimer 5. The benzyl ether 6 was deprotected to yield procyanidin C2 (1) by hydrogenolysis over Pearlman’s catalyst. As to the purification of 1, when the crude products were directly evaporated, partially insoluble materials remained.14 We met with some difficulty to purify 1 after debenzylation. We tried separation by ODS cartridge column chromatography and preparative HPLC, however, we could not obtain 1 in a satisfying yield. Kozikowski and colleagues reported that lyophilization is useful for purification of these type compounds.14 Thus we used this method to afford procyanidin C2 (1) in good yield. We confirmed that lyophilized procyanidin C2 (1) was pure by HPLC analysis.15 The optical rotation value and mass spectrum data of synthetic 1 were in good accordance with those of the reported values.9 With regard to the 1H and 13C NMR spectral data of 1, we could not find them in any reports to the best of our knowledge. In this article, we wish to supply these data in experimental section and supporting information for future reference. The 1H NMR spectral datum of peracetate 7 was in good agreement with that of the reported values (Scheme 2).16
As to the synthesis of procyanidin C1 (2), equimolar condensation of 8 with 9 was examined using transition metal Lewis acids and Yb(OTf)3 in CH2Cl2. As shown in Table 1, AgBF4 and AgOTf gave condensed product 109,14 in poor yield although these silver Lewis acids gave good results in the condensation of 3 with 4. The stereochemistry at C-3 position of 9 seemed to affect the reactivity, however, we could not explain the reason at this stage and further investigation is necessary. Next we used Yb(OTf)3 and found that the yield of condensation was slightly improved (Table 1).
This result encouraged us to examine equimolar condensation of 8 with various 4-alkoxy epicatechin derivatives 11-13 using Yb(OTf)3. As shown in Table 2, 4-(2”-ethoxyethoxy) derivative 13 afforded condensed product 10 in 57% yield. Other 4-alkoxy derivatives gave 10 in low yield and both of the starting materials remained. We found that 4-(2”-ethoxyethoxy) group was suitable for Yb(OTf)3 mediated activation at C-4 position of electrophile (Table 2).
The condensed product 10 was transformed into triol 14 using n-Bu4NOH.14 The 1H and 13C NMR spectral data of 14 were in good agreement with those of the reported values. The 3,4-cis diastereomer could not be detected.9,14 Finally deprotection of the benzyl group of 14 subsequent lyophilization afforded procyanidin C1 (2) in good yield. We confirmed that lyophilized procyanidin C1 (2) was pure by HPLC analysis (Scheme 3).17
The optical rotation value and spectral data of synthetic 2 were in good accordance with those of the reported values.9,14 The 1H and 13C NMR spectral data of peracetate 15 were in good agreement with those of the reported values.14
CONCLUSION
Synthesis of procyanidin C2 (1) was achieved via AgBF4 or AgOTf mediated intermolecular condensation using equimolar amount of dimeric catechin nucleophile 3 and monomeric catechin electrophile 4. As to the synthesis of procyanidin C1 (2), dimeric epicatechin derivative 8 and 4-(2”-ethoxyethoxy) epicatechin electrophile 13 in the presence of Yb(OTf)3 afforded condensed product 10 in moderate yield. For purifying procyanidin C2 (1) and C1 (2) after debenzylation, we recognized that lyophilization was useful.
EXPERIMENTAL
General
1H and 13C NMR spectra were measured with a Bruker Avance DRX 500 FT-NMR spectrometer in CDCl3 or CD3OD at 500 and 125 MHz, respectively. Chemical shifts were relative to tetramethylsilane as an internal standard. The coupling constants were given in Hz. Mass spectra were obtained on Shimadzu LCMS-2010EV and Waters Xevo QTOF mass spectrometers. Optical rotations were measured with a JASCO DIP 1000 polarimeter. Merck silica gel 60 (70-230 mesh) was used for column chromatography unless otherwise noted. Solvents for reactions were distilled prior to use: THF from Na and benzophenone, CH2Cl2 from CaH2.
3-O-Acetyl-tris(5,7,3’,4’-tetra-O-benzyl)catechin (4α/8)2-trimer (5). To a solution of nucleophile 3 (68 mg, 0.052 mmol) and electrophile 4 (38 mg, 0.052 mmol) in CH2Cl2 (4 mL) under an argon atmosphere was added AgBF4 (10 mg, 0.052 mmol). After the resulting mixture had been stirred for 3 h at room temperature, the reaction was quenched with water. The mixture was extracted with Et2O, and the combined organic layer was washed with brine, dried over MgSO4, filtered, and concentrated. The crude product was purified with silica gel column chromatography (hexane:AcOEt:CH2Cl2 = 4:1:2) to give 5 (89 mg, 85%) as a colorless oil. [α]D16 −100 (c 3.20, CHCl3). ESIMS: m/z 2011.9 [M+Na]+.
Tris(5,7,3’,4’-tetra-O-benzyl)catechin (4α/8)2-trimer (6). To a solution of 5 (108 mg, 0.054 mmol) in THF (3 mL) was added 40% aqueous n-Bu4NOH (0.58 mL, 0.89 mmol). The reaction mixture was allowed to be stirred for 96 h at room temperature, then partially evaporated to remove THF. The residue was diluted with H2O (5 mL), and the product was extracted with EtOAc (2×5 mL). The combined organic layer was washed with brine and concentrated. The residue was purified with preparative TLC (hexane:AcOEt:CH2Cl2 = 4:1:2) to afford 6 (70 mg, 66%) as a colorless foam. [α]D22 −143 (c 0.850, CHCl3), {lit., [α]D26 −82.2 (c 0.12, CHCl3)}.9 1H NMR (CDCl3, 0.82:0.18 mixture of rotational isomer, major isomer) δ = 7.44-6.40 (69H, m), 6.17 (1H, d, J = 2.3 Hz), 6.16 (1H, d, J = 2.3 Hz), 6.09 (1H, d, J = 2.3 Hz), 6.08 (1H, s), 5.22-4.40 (26H, m), 4.25 (1H, d, J = 8.8 Hz), 4.05-3.95 (2H, m), 3.81-3.75 (1H, m), 3.60 (1H, d, J = 8.9 Hz), 3.10 (1H, dd, J = 16.0, 6.0 Hz), 2.96 (1H, d, J = 9.5 Hz), 2.37 (1H, dd, J = 16.5, 9.8 Hz), 1.36 (1H, d, J = 4.0 Hz, -OH), 1.33 (1H, d, J = 3.5 Hz, -OH), 1.14 (1H, d, J = 3.5 Hz), 13C NMR (CDCl3, major isomer) δ = 157.9, 157.8, 156.7, 155.5, 155.4, 155.2, 155.1, 155.0, 153.8, 149.2, 149.1, 148.8, 148.6, 137.7, 137.5, 137.4, 137.3, 137.2, 137.1, 136.0, 132.4, 131.7, 128.9-126.9, 121.1, 120.7, 120.6, 115.1, 114.9, 114.8, 114.6, 114.2, 113.8, 112.4, 109.4, 108.8, 102.4, 94.7, 93.8, 92.0, 91.8, 81.8, 81.0, 80.4, 73.1, 73.0, 71.9-69.8, 68.4, 37.6, 37.5, 28.5; ESIMS: m/z 1969.8 [M+Na]+.
Procyanidin C2 (1). A solution of 6 (74 mg, 0.038 mmol) in THF/MeOH/H2O (20/20/1) (15 mL) was hydrogenated over 20% Pd(OH)2/C (65 mg) for 1 h at room temperature. The mixture was filtered and filtration residue was washed with MeOH (20 mL). The combined filtrates were evaporated, and the residue was taken up in distilled water (10 mL). The solution was filtered and lyophilized to give 1 (30 mg, 81%) as a fluffy amorphous solid. [α]D20 −274 (c 0.110, EtOH), {lit., [α]D20 −233.7 (c 0.38, EtOH)}.9 1H NMR (CD3OD) δ = 7.05-6.50 (8H, m), 6.40-5.65 (5H, m), 4.80-4.05 (6H, m), 4.00-3.50 (1H, m), 2.90-2.10 (3H, m); 13C NMR (CD3OD) δ = 158.5, 158.0, 157.7, 157.3, 156.9, 156.1, 155.7, 154.5, 146.3, 146.2, 146.0, 145.9, 145.3, 145.2, 132.6, 132.4, 132.3, 132.1, 132.0, 131.6, 121.1, 120.5, 120.3, 120.2, 120.0, 117.3, 116.7, 116.6, 116.4, 116.1, 115.7, 115.3, 114.1, 113.1, 109.2, 108.8, 108.6, 107.4, 98.7, 97.5, 97.3, 96.8, 96.4, 96.0, 95.5, 85.6, 84.0, 83.7, 83.4, 83.0, 82.9, 80.0, 78.1, 74.9, 74.0, 73.7, 73.0, 72.8, 39.1, 38.8, 38.7, 28.5; HRESIMS calcd for C45H37O18 [M−H]−; 865.1979; found 865.1955.
Peracetate of 1 (7). Acetylation of 1 (6 mg, 6.9 µmol) using general procedure gave peracetate 7 (5 mg, 35%). [α]D19 −205 (c 0.302, CHCl3); 1H NMR (CDCl3) δ = 7.27 (2H, d, J = 8.5 Hz), 7.10-7.05 (5H, m), 6.86 (1H, d, J = 1.5 Hz), 6.80 (1H, d, J = 2.0 Hz), 6.67 (1H, s), 6.62 (1H, s), 6.54 (1H, d, J = 2.5 Hz), 6.55-6.50 (2H, m), 6.21 (1H, d, J = 2.5 Hz), 5.58 (1H, dd, J = 10.0, 9.0 Hz), 5.48 (1H, dd, J = 10.0, 8.5 Hz), 5.25 (1H, brs), 5.23 (2H, m), 4.74 (1H, d, J = 10.0 Hz), 4.62 (1H, d, J = 10.0 Hz), 4.57 (1H, d, J = 8.5 Hz), 4.12 (1H, d, J = 9.0 Hz), 2.60-2.55 (1H, m), 2.35 (3H, s), 2.34 (3H, s), 2.29 (3H, s), 2.272 (9H, s), 2.269 (3H, s), 2.25 (3H, s), 2.22 (3H, s), 2.14 (3H, s), 2.07 (3H, s), 1.96 (3H, s), 1.65 (3H, s), 1.64 (3H, s), 1.62 (3H, s); 13C NMR (CDCl3) δ = 171.4, 169.7, 169.4, 168.7, 168.6, 168.4, 168.1, 167.9, 167.6, 156.3, 154.8, 151.1, 150.0, 149.2, 148.0, 147.4, 147.2, 147.0, 142.5, 141.9, 141.6, 141.5, 141.1, 136.6, 135.1, 134.9, 125.6, 124.6, 123.7, 123.2, 122.9, 122.5, 122.4, 119.5, 118.7, 117.2, 116.7, 116.6, 110.4, 109.8, 109.0, 108.4, 108.3, 79.7, 78.5, 76.3, 72.1, 70.9, 66.6, 53.4, 36.7, 29.7, 21.2, 20.9, 20.7, 20.6, 20.5, 20.4, 20.3, 20.2.
3-O-Acetyl-tris(5,7,3’,4’-tetra-O-benzyl)epicatechin (4β/8)2-trimer (10). To a solution of nucleophile 8 (58 mg, 0.045 mmol) and electrophile 13 (35 mg, 0.045 mmol) in CH2Cl2 (4 mL) under an argon atmosphere was added Yb(OTf)3 (28 mg, 0.045 mmol). After the resulting mixture had been stirred for 1 h at room temperature, the reaction was quenched with water. The mixture was extracted with Et2O, and the combined organic layer was washed with brine, dried over MgSO4, filtered, and concentrated. The crude product was purified with silica gel column chromatography (hexane:AcOEt:CH2Cl2 = 4:1:2) to give 10 (51 mg, 57%) as a colorless oil. [α]D19 +129 (c 0.901, CHCl3). ESIMS: m/z 2011.9 [M+Na]+.
Tris(5,7,3’,4’-tetra-O-benzyl)epicatechin (4β/8)2-trimer (14). In the same manner as described the synthesis of 6, compound 10 (42 mg, 0.021 mmol) afforded 14 (27 mg, 66%) as a colorless foam. [α]D18 +93.9 (c 1.32, CHCl3), {lit., [α]D25 +75.9 (c 0.32, CHCl3)}.9 1H NMR (CDCl3, 0.5:0.5 mixture of rotational isomer) δ = 7.51-6.73 (67.5H, m), 6.45 (0.5H, d, J = 8.0 Hz), 6.39 (0.5H, d, J = 8.0 Hz), 6.35 (0.5H, s), 6.27 (0.5H, d, J = 2.5 Hz), 6.18 (0.5H, s), 6.13 (0.5H, dd, J = 8.5, 1.5 Hz), 6.06 (0.5H, d, J = 2.0 Hz), 5.95 (0.5H, d, J = 2.0 Hz), 5.90 (0.5H, s), 5.85 (0.5H, s), 5.73 (0.5H, s), 5.70 (0.5H, d, J = 2.0 Hz), 5.49 (0.5H, s), 5.18-4.05 (29.5H, m), 3.95 (0.5H, d, J = 5.5 Hz), 3.64 (0.5H, s), 2.97-2.80 (2H, m), 1.81 (0.5H, d, J = 5.5 Hz, -OH), 1.70 (0.5H, d, J = 6.5 Hz, -OH), 1.55 (1H, m), 1.45 (0.5H, brs), 1.20 (1H, brs); 13C NMR (CDCl3) δ = 158.3, 158.1, 158.0, 157.4, 156.8, 156.5, 156.4, 156.2, 156.1, 155.7, 155.5, 155.3, 154.7, 137.3, 137.2, 137.0, 132.9, 132.6, 132.4, 132.3, 131.3, 128.6-126.2, 120.0, 119.7, 118.9, 118.8, 115.1, 115.0, 114.8, 114.2, 113.6, 113.5, 113.2, 113.0, 111.3, 111.1, 110.1, 106.0, 105.4, 104.7, 104.6, 101.4, 100.8, 94.4, 94.0, 93.8, 93.4, 92.6, 92.5, 92.3, 90.3, 72.7, 72.5, 71.4-69.3, 65.3, 64.9, 36.6, 36.2, 35.5, 28.6; ESIMS: m/z 1969.8 [M+Na]+.
Procyanidin C1 (2). In the same manner as described the synthesis of 1, compound 14 (54 mg, 0.028 mmol) afforded 2 (25 mg, 92%) as a fluffy amorphous solid. [α]D19 +60.7 (c 0.45, MeOH), {lit., [α]D27 +59 (c 0.30, MeOH), 9 [α]D +70.4 (c 2.2, MeOH)14}. 1H NMR (CD3OD) δ = 7.13 (1H, s), 7.02 (1H, s), 6.92 (2H, s), 6.77-6.70 (5H, m), 6.02 (2H, d, J = 16.5 Hz), 5.93 (2H, s), 5.22 (1H, s), 5.07 (1H, s), 4.99 (1H, s), 4.71 (2H, brs), 4.33 (1H, s), 4.01 (2H, m), 2.95 (1H, d, J = 15.5 Hz), 2.81 (1H, d, J = 16.5 Hz); 13C NMR (CD3OD) δ = 158.6, 157.3, 156.9, 156.6, 154.6, 146.0, 145.8, 145.7, 132.7, 132.1, 119.3, 119.1, 118.9, 116.0, 115.3, 115.1, 107.2, 101.4, 100.6, 97.6, 96.6, 96.2, 79.7, 77.1, 73.5, 72.9, 66.8, 59.5, 39.1, 37.4, 33.1, 30.7, 30.6, 29.8, 24.8; HRESIMS calcd for C45H37O18 [M−H]−; 865.1979; found 865.1984.
Peracetate of 2 (15). Acetylation of 2 (14 mg, 16 µmol) using general procedure gave peracetate 15 (11 mg, 34%). [α]D17 +56 (c 0.35, acetone), {lit., [α]D +55.3 (c 10.4, acetone)},14 1H NMR (CDCl3, 2:1 mixture of rotational isomers) δ = 7.37 (0.33H, d, J = 2.0 Hz), 7.29 (0.67H, d, J = 1.5 Hz), 7.25 (0.33H, d, J = 1.5 Hz), 7.29-7.05 (1.65H, m), 7.20-7.05 (5.67H, m), 6.99 (0.33H, d, J = 2.0 Hz), 6.94 (0.33H, d, J = 8.5 Hz), 6.89 (0.33H, s), 6.79 (0.33H, dd, J = 8.5, 2.0 Hz), 6.76 (0.67H, d, J = 2.0 Hz), 6.72 (0.67H, s), 6.65 (0.67H, s), 6.60 (0.33H, s), 6.25 (0.33H, d, J = 2.0 Hz), 5.94 (0.33H, d, J = 2.0 Hz), 5.70 (0.33H, brs), 5.47 (0.67H, d, J = 4.5 Hz), 5.40 (1.67H, m), 5.36 (1.33H, m), 5.21 (0.67H, brs), 5.12 (0.33H, brs), 5.11 (0.33H, brs), 4.94 (0.33H, m), 4.78 (0.67H, brs), 4.70 (0.67H, brs), 4.67 (0.33H, brs), 4.66 (0.33H, brs), 4.49 (0.33H, d, J = 2.5 Hz), 3.07 (0.67H, dd, J = 18.0, 5.0 Hz), 3.02 (0.33H, dd, J = 18.0, 5.0 Hz), 2.97 (0.67H, m), 2.89 (0.33H, m), 2.38-1.38 (45H, m); 13C NMR (CDCl3) δ = 170.1, 169.9, 169.0, 168.8, 168.7, 168.5, 168.4, 168.3, 168.1, 168.0, 167.9, 167.8, 167.7, 167.6, 167.4, 154.9, 154.0, 151.8, 151.7, 149.9, 148.6, 148.5, 148.3, 147.9, 147.6, 147.2, 146.9, 142.1, 142.0, 141.9, 141.8, 141.6, 141.5, 136.5, 135.7, 135.4, 135.1, 133.1, 124.8, 124.2, 124.1, 123.9, 123.2, 123.1, 123.0, 122.7, 122.0, 121.6, 121.5, 121.3, 117.8, 117.7, 117.6, 117.2, 112.2, 111.6, 110.9, 110.6, 110.5, 110.0, 109.9, 109.3, 108.1, 107.5, 107.4, 76.1, 74.9, 74.7, 73.8, 71.4, 71.2, 70.9, 70.4, 66.6, 35.6, 35.0, 34.3, 32.9, 31.9, 29.7, 29.4, 22.7, 21.2, 21.0, 20.8, 20.7, 20.6, 20.5, 20.4, 20.2, 20.1, 20.0, 19.8, 19.7, 19.5.
SUPPORTING INFORMATION
1H and 13C NMR spectra of 1, 2, 7, and 15.
ACKNOWLEDGEMENTS
We thank financial support from Japan Science and Technology Agency, Adaptable and Seamless Technology transfer Program through target-driven R&D (to HM (AS232Z00626E)).
References
1. D. Ferreira and X.-C. Li, Nat. Prod. Rep., 2000, 17, 193. CrossRef
2. D. Ferreira and X.-C. Li, Nat. Prod. Rep., 2002, 19, 517. CrossRef
3. N. Seeram, M. Aviram, Y. Zhang, S. M. Hennings, L. Feng, M. Dreher, and D. Heber, J. Agric. Food Chem., 2008, 56, 1415. CrossRef
4. S. Mitsuhashi, A. Saito, N. Nakajima, H. Shima, and M. Ubukata, Molecules, 2008, 13, 2998. CrossRef
5. H. Y. Cheng, T. C. Lin, C. M. Yang, D. E. Shieh, and C. C. Lin, J. Sci. Food Agric., 2005, 85, 10. CrossRef
6. X. Terra, J. Valls, X. Vitrac, J. M. Merrillon, L. Arola, A. Ardevol, C. Blade, J. Fernandez-Larrea, G. Pujadas, J. Salvado, and M. Blay, J. Agric. Food Chem., 2007, 55, 4357. CrossRef
7. A. Saito, Y. Mizushina, H. Ikawa, H. Yoshida, Y. Doi, A. Tanaka, and N. Nakajima, Bioorg. Med. Chem., 2005, 13, 2759. CrossRef
8. D. Ferreira and C. M. Coleman, Planta Med., 2011, 77, 1071; CrossRef K-i. Oyama, K. Yoshida, and T. Kondo, Curr. Org. Chem., 2011, 15, 2567; CrossRef K. Ohmori and K. Suzuki, Curr. Org. Chem., 2012, 16, 566. CrossRef
9. A. Saito, A. Tanaka, M. Ubukata, and N. Nakajima, Synlett, 2004, 1069; CrossRef A. Saito, Y. Doi, A. Tanaka, N. Matsuura, M. Ubukata, and N. Nakajima, Bioorg. Med. Chem., 2004, 12, 4783; CrossRef A. Saito, Y. Mizushina, A. Tanaka, and N. Nakajima, Tetrahedron, 2009, 65, 7422. CrossRef
10. K. Ohmori, N. Ushimaru, and K. Suzuki, Proc. Natl. Acad. Sci. USA, 2004, 101, 12002; CrossRef K. Ohmori, T. Shono, Y. Hatakoshi, T. Yano, and K. Suzuki, Angew. Chem. Int. Ed., 2011, 50, 4862. CrossRef
11. E. G. Dennis, D. W. Jeffery, M. R. Johnston, M. V. Perkins, and P. A. Smith, Tetrahedron, 2012, 68, 340. CrossRef
12. Y. Oizumi, Y. Mohri, Y. Hattori, and H. Makabe, Heterocycles, 2011, 83, 739. CrossRef
13. Y. Mohri, M. Sagehashi, T. Yamada, Y. Hattori, K. Morimura, T. Kamo, M. Hirota, and H. Makabe, Tetrahedron Lett., 2007, 48, 5891; CrossRef Y. Mohri, M. Sagehashi, T. Yamada, Y. Hattori, K. Morimura, Y. Hamauzu, T. Kamo, M. Hirota, and H. Makabe, Heterocycles, 2009, 79, 549; CrossRef Y. Oizumi, Y. Mohri, M. Hirota, and H. Makabe, J. Org. Chem., 2010, 75, 4884; CrossRef M. Katoh, Y. Oizumi, Y. Mohri, M. Hirota, and H. Makabe, Lett. Org. Chem., 2012, 9, 233. CrossRef
14. A. P. Kozikowski, W. Tückmantel, G. Böttcher, and L. J. Romanczyk, Jr., J. Org. Chem., 2003, 68, 1641. CrossRef
15. HPLC measurement condition: column; Inertsil ODS-3 250×4.6 mm GL-Sciences, eluent MeOH-0.2%AcOH (5:95~25:75), flow rate: 0.8 mL/min, detection: UV 280 nm, retention time: 15.35 min.
16. J. A. Delcour and S. A. R. Vercruysse, J. Inst. Brew., 1986, 92, 244.
17. HPLC measurement condition: column; Inertsil ODS-3 250×4.6 mm GL-Sciences, eluent MeOH-0.2%AcOH (5:95~25:75), flow rate: 0.8 mL/min, detection: UV 280 nm, retention time: 23.60 min.