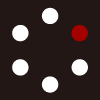
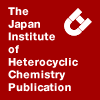
HETEROCYCLES
An International Journal for Reviews and Communications in Heterocyclic ChemistryWeb Edition ISSN: 1881-0942
Published online by The Japan Institute of Heterocyclic Chemistry
e-Journal
Full Text HTML
Received, 20th June, 2012, Accepted, 2nd August, 2012, Published online, 3rd August, 2012.
DOI: 10.3987/COM-12-S(N)45
■ SYNTHESIS OF PHENANTHRIDINES AND RELATED COMPOUNDS BY PALLADIUM-CATALYZED DIRECT COUPLING VIA C–H AND N–H BOND CLEAVAGES
Daisuke Takeda, Koji Hirano, Tetsuya Satoh,* and Masahiro Miura*
Department of Applied Chemistry, Graduate School of Engineering, Osaka University, 2-1 Yamadaoka, Suita, Osaka 565-0871, Japan
Abstract
The palladium-catalyzed direct annulation of benzophenone imines with o-dihalobenzenes proceeds through C–H and N–H bond cleavages to produce 6-arylphenanthridine derivatives. 2-Phenyl-imidazole, -benzimidazole, and -indole also undergo the annulation to form the corresponding tetra- and pentacyclic compounds.INTRODUCTION
Nitrogen-containing fused heteroaromatic compounds including phenanthridines can be seen in a wide range of π-conjugated functional materials such as organic semiconductors and luminescent materials as well as pharmaceuticals and natural products.1 Among them, 6-arylphenanthridine derivatives are of particular interest due to their applicability as ligands for phosphorescent iridium complexes, which are promising emitters in pure red region.2 Therefore, their effective construction has attracted much attention and a number of synthetic approaches through transition-metal-catalyzed cyclization reactions have been developed (Scheme 1). However, in reported procedures shown as routes a,3 b,4 and c,5 starting materials have been prepared through complicated multistep processes.
In contrast, the annulation between two aromatic substrates (routes a & c) appears to be attractive because of the substrate availability and applicability.6 In the course of our recent studies on transition-metal-catalyzed annulation reactions involving C–H bond cleavage,7 we succeeded in conducting the one step synthesis of 6-arylphenanthridines through the direct coupling of benzophenone imines with o-dihalobenzenes under palladium catalysis, accompanied by C–H and N–H bond cleavages. The catalyst was also found to be applicable to the related annulation of 2-phenyl-imidazoles and -indole. These new findings are described herein.
RESULTS AND DISCUSSION
In an initial attempt, benzophenone imine (1a, 0.5 mmol) was treated with o-dibromobenzene (2a, 0.4 mmol) in the presence of Pd(OAc)2 (0.02 mmol), PCy3 (0.04 mmol), and K2CO3 (0.9 mmol) as a catalyst, ligand, and base, respectively, in DMF at 160 oC for 9 h. As a result, 6-phenylphenanthridine (3a) was obtained in 53% yield (Table 1, Entry 1). The use of other bases such as Na2CO3, Cs2CO3, KOBut, and K3PO4 in place of K2CO3 decreased the product yield (Entries 2-5). The reaction proceeded more efficiently at 170 oC in DMAc to afford 3a in 65% yield (Entry 6). Even at 170 oC, the 3a yield decreased in NMP, diglyme, or mesitylene (Entries 7-9). Without PCy3, the reaction did not proceed catalytically (Entry 10). Increasing the amount of PCy3 to 0.08 mmol decreased the product yield (Entry 11). Other mono- (Entries 12 and 13) and diphosphine ligands (Entries 14-17) were less effective than PCy3. In the case using xantphos (Entry 17), a significant amount (45%) of monobromide 4 was formed together with 3a.
Under the optimized reaction conditions (Table 1, Entry 6), the reactions of a series of p-substituted benzophenone imines 1b-d with 2a were next examined (Scheme 2). 4,4’-Dimethyl- and 4,4’-di-tert-butylbenzophenone imines (1b and 1c) coupled with 2a effectively to form the corresponding phenanthridines 3b and 3c in 51 and 60% yields, respectively. In the reaction of 4,4’-dimethoxybenzophenone imine (1d), only a small amount of coupling product was detected by GC and GC-MS. The poor result seems to be due, at least in part, to the poorer solubility of 1d and/or the product.8
Under similar conditions, the reactions of 1a with o-bromochlorobenzene (2b) and o-dichlorobenzene (2c) gave 3a in low yields (Scheme 3). 1,2-Dibromo-4,5-dimethylbenzene (2d) coupled with 1a effectively to produce 3e in 52% yield. In contrast, the reaction of an electron-deficient dibromide, 1,2-dibromo-4,5-difluorobenzene (2e), was sluggish.
A plausible mechanism for the coupling of 1a with 2a is illustrated in Scheme 4, in which neutral ligands are omitted. Taking account of a fact that the N-arylation of 1a is known to occur under mild conditions at 65 oC,9 the arylation seems to first occur on the imino nitrogen of 1a to produce a monobromide 4. Actually, 4 was obtained in the case using xantphos ligand (Table 1, Entry 17), as described above. Then, the next intramolecular C–H arylation may occur as follows: Oxidative addition of the remaining C–Br bond in 4 to Pd0 species gives arylpalladium species A. Subsequent cyclopalladation and reductive elimination take place to form 3a and regenerate Pd0 species.
Under similar conditions, 2-phenylimidazole (5) also underwent the annulation with 2a, accompanied by C–H and N–H bond cleavages to afford a tetracyclic product 6 in 20% yield (Scheme 5). Increase of the amount of PCy3 ligand to 0.08 mmol improved the product yield up to 57%. Although the exact effect of increased ligand is not clear at the present stage, the deposition of palladium black was observed to be slightly retarded. Similarly, 2-phenylbenzimidazole (7) and 2-phenylindole (9) also coupled with 2a under modified conditions using Cs2CO3 as a base in place of K2CO3 to produce 8 and 10, respectively. In the latter case, the use of 0.12 mmol of PCy3 gave the best result.
In summary, we have demonstrated that the synthesis of phenanthridine derivatives from the readily available building blocks, benzophenone imines and o-dihalobenzenes, can be achieved through C–H and N–H bond cleavages under palladium catalysis. The procedure is also applicable to the construction of related fused tetra- and pentacyclic heteroarenes.
EXPERIMENTAL
General. 1H and 13C NMR spectra were recorded at 400 and 100 MHz for CDCl3 solutions. MS data were obtained by EI. GC analysis was carried out using a silicon OV-17 column (i. d. 2.6 mm x 1.5 m). GC-MS analysis was carried out using a CBP-1 capillary column (i. d. 0.25 mm x 25 m). The structures of all products listed below were unambiguously determined by 1H and 13C NMR with the aid of NOE, COSY, HMQC, and HMBC experiments.
Starting Materials. Imines 1b-d were prepared according to published procedures.10 Other starting materials were commercially available.
Typical Procedure for the Reactions of Benzophenone Imines 1, 2-Phenylimidazoles 5 and 7, and 2-Phenylindole 9 with o-Dihalobenzene 2. A mixture of nitrogen-containing substrate 1, 5, 7, or 9 (0.5 mmol), o-dihalobenzene 2 (0.4 mmol), Pd(OAc)2 (0.02 mmol, 4.5 mg), PCy3 (0.04-0.12 mmol), base (0.9 mmol), and dibenzyl (ca. 40 mg) as internal standard was stirred in DMAc (2.5 mL) under nitrogen at 170 oC for 6-9 h. GC and GC-MS analyzes of the mixtures confirmed formation of product 3, 6, 8, or 10. After cooling, the reaction mixture was extracted with ethyl acetate (100 mL). The organic layer was washed with water (100 mL, three times), and dried over Na2SO4. After evaporation of the solvent under vacuum, the product 3, 6, 8, or 10 was isolated by column chromatography on silica gel using hexane-ethyl acetate as eluant.
Characterization Data of Products.
6-Phenylphenanthridine (3a):6d mp 99-101 oC; 1H NMR (400 MHz, CDCl3) δ 7.51-7.63 (m, 4H), 7.67-7.78 (m, 4H), 7.84-7.88 (m, 1H), 8.11 (d, J = 8.4 Hz, 1H), 8.25 (d, J = 7.8 Hz, 1H), 8.62 (d, J = 8.2 Hz, 1H), 8.71 (d, J = 8.4 Hz, 1H); 13C NMR (100 MHz, CDCl3) δ 121.9, 122.2, 123.7, 125.2, 126.9, 127.1, 128.4, 128.7, 128.8, 128.9, 129.7, 130.3, 130.6, 133.4, 139.8, 143.8, 161.3; HRMS m/z (M+) Calcd for C19H13N: 255.1048. Found 255.1045.
9-Methyl-6-(4-methylphenyl)phenanthridine (3b):6d mp 70-72 oC; 1H NMR (400 MHz, CDCl3) δ 2.47 (s, 3H), 2.64 (s, 3H), 7.36 (d, J = 7.3 Hz, 2H), 7.42 (d, J = 8.7 Hz, 1H), 7.62-7.66 (m, 3H), 7.69-7.74 (m, 1H), 8.01 (d, J = 8.7 Hz, 1H), 8.21 (d, J = 8.2 Hz, 1H), 8.46 (s, 1H), 8.58 (d, J = 8.3 Hz, 1H); 13C NMR (100 MHz, CDCl3) δ 21.4, 22.2, 121.8, 121.9, 123.45, 123.54, 126.5, 128.6, 128.7, 128.9, 129.0, 129.7, 130.3, 133.6, 137.1, 138.5, 140.8, 144.1, 161.1; HRMS m/z (M+) Calcd for C21H17N: 283.1361. Found 283.1358.
9-(tert-Butyl)-6-[4-(tert-butyl)phenyl]phenanthridine (3c): mp 178-179 oC; 1H NMR (400 MHz, CDCl3) δ 1.41 (s, 9H), 1.50 (s, 9H), 7.56-7.58 (m, 2H), 7.65-7.75 (m, 5H), 8.12 (d, J = 8.7 Hz, 1H), 8.23 (d, J = 8.7 Hz, 1H), 8.64-8.68 (m, 2H); 13C NMR (100 MHz, CDCl3) δ 31.3, 31.4, 34.7, 35.5, 117.7, 121.8, 123.4, 123.9, 125.35, 125.38, 126.5, 128.5, 128.8, 129.4, 130.3, 133.2, 137.0, 144.1, 151.6, 153.7, 161.0; HRMS m/z (M+) Calcd for C27H29N: 367.2300. Found 367.2302.
2,3-Dimethyl-6-phenylphenanthridine (3e):6d mp 92-94 oC; 1H NMR (400 MHz, CDCl3) δ 2.50 (s, 3H), 2.55 (s, 3H), 7.48-7.57 (m, 4H), 7.71-7.73 (m, 2H), 7.79-7.83 (m, 1H), 8.01 (s, 1H), 8.07 (d, J = 8.2 Hz, 1H), 8.34 (s, 1H), 8.65 (d, J = 8.2 Hz, 1H); 13C NMR (100 MHz, CDCl3) δ 20.1, 20.4, 121.7, 121.96, 121.98, 125.0, 126.5, 128.4, 128.5, 128.8, 129.8, 130.23, 130.24, 133.2, 136.4, 138.4, 140.0, 142.6, 160.3; HRMS m/z (M+) Calcd for C21H17N: 283.1361. Found 283.1359.
2-Bromo-N-(diphenylmethylene)aniline (4):11 mp 97-99 oC; 1H NMR (400 MHz, CDCl3) δ 6.53 (d, J = 7.8 Hz, 1H), 6.76-6.80 (m, 1H), 7.00-7.04 (m, 1H), 7.19-7.27 (m, 5H), 7.40-7.51 (m, 4H), 7.82 (d, J = 7.3 Hz, 2H); 13C NMR (100 MHz, CDCl3) δ 115.2, 121.2, 124.2, 127.4, 127.9, 128.2, 128.7, 128.8, 129.6, 131.1, 132.4, 136.0, 138.8, 150.2, 169.4; HRMS m/z (M+) Calcd for C19H14BrN: 335.0310. Found 335.0308.
Imidazo[1,2-f]phenanthridine (6):12 mp 115-124 oC; 1H NMR (400 MHz, CDCl3) δ 7.49 (td, J = 8.2 and 1.4 Hz, 1H), 7.58-7.66 (m, 4H), 7.84 (dd, J = 8.2 and 0.9 Hz, 1H), 7.97 (d, J = 1.4 Hz, 1H), 8.33-8.37 (m, 1H), 8.43 (dd, J = 8.3 and 1.4 Hz, 1H), 8.63-8.97 (m, 1H); 13C NMR (100 MHz, CDCl3) δ 112.0, 115.8, 121.7, 122.3, 123.7, 124.11, 124.12, 125.1, 127.4, 128.55, 128.60, 128.8, 131.5, 131.7, 142.5; HRMS m/z (M+) Calcd for C15H10N2: 218.0844. Found 218.0842.
Benzo[4,5]imidazo[1,2-f]phenanthridine (8):13 mp 145-147 oC; 1H NMR (400 MHz, CDCl3) δ 7.44-7.54 (m, 3H), 7.64-7.73 (m, 3H), 8.03-8.06 (m, 1H), 8.33 (t, J = 8.3 Hz, 2H), 8.43 (dd, J = 8.0 and 1.2 Hz, 1H), 8.52 (d, J = 7.8 Hz, 1H), 8.85 (dd, J = 7.8 and 1.8 Hz, 1H); 13C NMR (100 MHz, CDCl3) δ 113.9, 116.0, 120.3, 121.7, 122.3, 122.9, 123.4, 124.1, 124.2, 124.4, 126.0, 128.6, 129.1, 129.5, 130.4, 131.9, 134.4, 144.5, 147.5; HRMS m/z (M+) Calcd for C19H12N2: 268.1000. Found 268.1003.
Indolo[1,2-f]phenanthridine (10):14 mp 146-148 oC; 1H NMR (400 MHz, CDCl3) δ 7.28 (s, 1H), 7.33-7.42 (m, 3H), 7.48-7.53 (m, 2H), 7.57-7.61 (m, 1H), 7.85 (d, J = 8.0 Hz, 1H), 8.13-8.17 (m, 1H), 8.22-8.25 (m, 1H), 8.34 (d, J = 7.8 Hz, 1H), 8.40 (d, J = 8.2 Hz, 1H), 8.56 (d, J = 8.7 Hz, 1H); 13C NMR (100 MHz, CDCl3) δ 96.1, 114.2, 116.3, 121.0, 121.7, 121.95, 122.01, 122.3, 123.0, 123.9, 124.1, 126.1, 126.8, 127.8, 128.1, 128.7, 130.2, 133.8, 135.2, 135.9; HRMS m/z (M+) Calcd for C20H13N: 267.1048. Found 267.1044.
ACKNOWLEDGEMENTS
This work was partly supported by Grants-in-Aid from MEXT and JSPS, Japan and Kansai Research Foundation for technology promotion (KRF).
References
1. Selected examples: (a) V. Abet, A. Nuñez, F. Mendicuti, C. Burgos, and J. Alvarez-Builla, J. Org. Chem., 2008, 73, 8800; CrossRef (b) E. Ahmed, A. L. Briseno, Y. Xia, and S. A. Jenekhe, J. Am. Chem. Soc., 2008, 130, 1118; CrossRef (c) A. D. C. Parenty, Y.-F. Song, C. J. Richmond, and L. Cronin, Org. Lett., 2007, 9, 2253; CrossRef (d) E. Gondek, I. V. Kityk, A. Danel, A. Wisla, and J. Sanetra, Synth. Met., 2006, 156, 1348; CrossRef (e) I. B. Bersuker, S. Bahceci, J. E. Boggs, and R. S. Pearlman, J. Comput.-Aided Mol. Des., 1999, 13, 419; CrossRef (f) H. Tomoda, T. Hirano, S. Saito, T. Mutai, and K. Araki, Bull. Chem. Soc. Jpn., 1999, 72, 1327; CrossRef (g) J. R. Barrio, P. D. Sattsangi, B. A. Gruber, L. G. Dammann, and N. J. Leonard, J. Am. Chem. Soc., 1976, 98, 7408. CrossRef
2. Selected examples: (a) Y. H. Lee, N. G. Park, Y. Ha, and Y. S. Kim, Jpn. J. Appl. Phys., 2006, 45, 563; CrossRef (b) Y.-J. Su, H.-L. Huang, C.-L. Li, C.-H. Chien, Y.-T. Tao, P.-T. Chou, S. Datta, and R.-S. Liu, Adv. Mater., 2003, 15, 884. CrossRef
3. J. Peng, T. Chen, C. Chen, and B. Li, J. Org. Chem., 2011, 76, 9507. CrossRef
4. S. W. Youn and J. H. Bihn, Tetrahedron Lett., 2009, 50, 4598. CrossRef
5. (a) L. Zhang, G. Y. Ang, and S. Chiba, Org. Lett., 2010, 12, 3682; CrossRef (b) M. Lysén, J. L. Kristensen, P. Vedsø, and M. Begtrup, Org. Lett., 2002, 4, 257. CrossRef
6. The annulation via routes a & c using complicated substrates has been reported: (a) M. Blanchot, D. A. Candito, F. Larnaud, and M. Lautens, Org. Lett., 2011, 13, 1486; CrossRef (b) G. Maestri, M.-H. Larraufie, E. Derat, C. Ollivier, L. Fensterbank, E. Lacôte, and M. Malacria, Org. Lett., 2010, 12, 5692; CrossRef (c) D. A. Candito and M. Lautens, Angew. Chem. Int. Ed., 2009, 48, 6713; CrossRef (d) T. Gerfaud, L. Neuville, and J. Zhu, Angew. Chem. Int. Ed., 2009, 48, 572. CrossRef
7. (a) K. Morimoto, M. Itoh, K. Hirano, T. Satoh, Y. Shibata, K. Tanaka, and M. Miura, Angew. Chem. Int. Ed., 2012, 51, 5359; CrossRef (b) K. Morimoto, K. Hirano, T. Satoh, and M. Miura, J. Org. Chem., 2011, 76, 9548; CrossRef (c) K. Morimoto, K. Hirano, T. Satoh, and M. Miura, Chem. Lett., 2011, 40, 600; CrossRef (d) N. Umeda, K. Hirano, T. Satoh, N. Shibata, H. Sato, and M. Miura, J. Org. Chem., 2011, 76, 13; CrossRef (e) S. Mochida, N. Umeda, K. Hirano, T. Satoh, and M. Miura, Chem. Lett., 2010, 39, 744; CrossRef (f) K. Morimoto, K. Hirano, T. Satoh, and M. Miura, Org. Lett., 2010, 12, 2068; CrossRef (g) S. Mochida, M. Shimizu, K. Hirano, T. Satoh, and M. Miura, Chem. Asian J., 2010, 5, 847; CrossRef (h) M. Yamashita, K. Hirano, T. Satoh, and M. Miura, J. Org. Chem., 2009, 74, 7481; CrossRef (i) T. Fukutani, N. Umeda, K. Hirano, T. Satoh, and M. Miura, Chem. Commun., 2009, 5141; CrossRef (j) S. Mochida, K. Hirano, T. Satoh, and M. Miura, J. Org. Chem., 2009, 74, 6295; CrossRef (k) M. Shimizu, K. Hirano, T. Satoh, and M. Miura, J. Org. Chem., 2009, 74, 3478; CrossRef (l) N. Umeda, H. Tsurugi, T. Satoh, and M. Miura, Angew. Chem. Int. Ed., 2008, 47, 4019; CrossRef (m) M. Shimizu, H. Tsurugi, T. Satoh, and M. Miura, Chem. Asian J., 2008, 3, 881; CrossRef (n) K. Ueura, T. Satoh, and M. Miura, J. Org. Chem., 2007, 72, 5362; CrossRef (o) K. Ueura, T. Satoh, and M. Miura, Org. Lett., 2007, 9, 1407. CrossRef
8. Actually, 1d was sparingly soluble in DMAc at room temperature.
9. J. P. Wolfe, J. Ahman, J. P. Sadighi, R. A. Singer, and S. L. Buchwald, Tetrahedron Lett., 1997, 38, 6367. CrossRef
10. P. L. Pickard and T. L. Tolbert, Org. Synth., 1964, 44, 51.
11. J. P. Wolfe, R. A. Singer, B.-H. Yang, and S. L. Buchwald, J. Am. Chem. Soc., 1999, 121, 9550. CrossRef
12. A. D. C. Parenty, K. M. Guthrie, Y.-F. Song, L. V. Smith, E. Burkholder, and L. Cronin, Chem. Commun., 2006, 1194. CrossRef
13. P. G. Houghton, D. F. Pipe, and C. W. Rees, J. Chem. Soc., Perkin Trans. 1, 1985, 1471. CrossRef
14. C.-S. Xie, Y.-H. Zhang, Z.-D. Huang, and P.-X. Xu, J. Org. Chem., 2007, 72, 5431. CrossRef