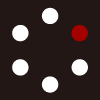
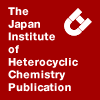
HETEROCYCLES
An International Journal for Reviews and Communications in Heterocyclic ChemistryWeb Edition ISSN: 1881-0942
Published online by The Japan Institute of Heterocyclic Chemistry
e-Journal
Full Text HTML
Received, 22nd June, 2012, Accepted, 16th August, 2012, Published online, 23rd August, 2012.
DOI: 10.3987/REV-12-747
■ Imidazoquinolines as Diverse and Interesting Building Blocks: Review of Synthetic Methodologies
Zeinab Mahmoud and Mohsen Daneshtalab*
School of Pharmacy, Memorial University of Newfoundland, St. John’s, NL, A1B 3V6, Canada
Abstract
Imidazoquinolines are interesting heterocyclic systems that depending on the position of fusion and the type of substituents display diverse biological and pharmaceutical properties. From the chemistry point of view, imidazoquinolines are heterocylic scaffolds resulting from the fusion between the 5-membered ring imidazole and the benzopyridine ring, quinoline. At least eight different combinations can be identified for these significant scaffolds. From the retrosynthetic point of view, imidazoquinolines can be prepared using one of two general approaches: imidazole-ring formation or pyridine-ring one. According to the type of imidazoquinoline and the position of the substituents either one of these two plans can be adopted. Pertinent to the specific plan to be used, different starting materials can be implanted as well. The most general starting materials are the diaminoquinolines and the aminobenzimidazoles. The main objective of this review article is to shed light on the reported synthetic pathways for each imidazoquinoline system.INTRODUCTION
Imidazoquinolines are classified as diverse and interesting heterocyclic systems that represent benzene-fused deazapurine scaffolds (Figure 1).1 These fused heterobicyclic scaffolds have shown significant biological and pharmaceutical properties depending on the position of the fusion and the nature of the substituents. A survey through the literature has revealed that different imidazoquinolines have been claimed to display contraceptive,2 antihypertensive,3 antiallergic,4 antiasthmatic,5 and antiplatelets aggregation6 activities. They have also been reported to act as immunomodifying,7 chelating,8 antibacterial,9 anticancer10 agents and non-xanthine adenosine antagonists.11
Consequently, imidazoquinolines can be classified into two main subgroups:
1) Angular imidazoquinolines (Figure 2)
2) Linear imidazoquinolines (Figure 3)
Hitherto, no comprehensive survey has been published to outline the different synthetic methods for the preparation of imidazoquinolines as fused heterocyclic systems. In this review article, we would like to comprehensively outline different methodologies for the syntheses of these structurally diverse scaffolds.
1. SYNTHETIC APPROACHES
1.1. ANGULAR IMIDAZOQUINOLINES
1.1.1. IMIDAZO[1,2-a]QUINOLINES
In accordance with the retrosynthetic possible pathways, the 2-aminoquinoline (1) is considered as the most common precursor for the preparation of imidazo[1,2-a]quinolines (Figure 4).12-16 Consequently, its reaction with α–halocarbonyl compounds such as ethyl 3-bromopyruvate yielded the desired nucleus in 63-96% yields (Scheme 1).12,13 The mechanism of the reaction can be summarized as the quinolinium quaternary salt 3 is first formed through the reaction of 2-aminoquinoline (1) with the pyruvate ester 2. Heating 3 in ethanol followed by basification with sodium carbonate furnishes the way to the desired derivative 4.12,13
The reaction of 1,1,1-trifluoro-4-(phenyl/methylsulfonyl)but-3-ene-2,2-diols, 5a,b, as 1,2-bielectrophilic reagents, with 2-aminoquinoline (1) is reported to afford the stable 2,3-dihydroimidazo[1,2-a]quinolin-2-ol derivatives 6a,b in 80-96% yields, respectively. (Scheme2). This straightforward one-step cyclization reaction possesses the advantages of being simple, environmentally safe, and having reasonable reaction times.14
The use of a Ugi three-component coupling (3cc) reaction strategy in combination with microwave-assisted heating has been reported by Ireland et al. for the preparation of 9a,b (Scheme 3).15 This multi-component synthetic procedure afforded 9a,b via the reaction of 1 with benzylisocyanide (7) and the appropriate aldehydes 8. The target compounds, 1-(benzylamino)-2-arylimidazo[1,2-a]quinolines 9a,b were formed through an iminium species followed by a [4+1] cycloaddition with the isocyanide. It is worth mentioning that the use of scandium triflate catalyst increased the conversion reaction rate to a notable extent.15
In the course of investigating the effect of 2-(arylmethylamino)quinolines 10a-f, as ligands for the Tec SH3 domain, Inglis and his co-workers16 reported the formation of the 3-fluorophenylimidazo[1,2-a]quinoline derivative 11. This unexpected product, 11, was separated in 10% yield under the reductive alkylation conditions of the amino group (Scheme 4).16
The same compound was further verified via an independent synthetic pathway. In this case, 2-aminoquinoline (1) was reacted with an aldehyde to afford the imine intermediate 12, which upon treatment with sodium cyanide in methanol at ca. 50 oC afforded 11 in 33% yield, along with a small percentage (4%) of 11a as a result of condensation of 11 with another molecule of aldehyde (Scheme 5).16
Although 2-aminoquinoline (1) is considered the most common precursor for the imidazo[1,2-a]quinolines, nevertheless, some other less-common approaches were also attempted. The polysubstituted imidazo[1,2-a]quinolines 16ai-cviii were successfully prepared in a facile manner using both microwave-assisted heating and 3cc domino reaction protocol.17 The above series of compounds were synthesized using the one-pot multicomponent strategy between the enaminones 13, malononitrile 14, and the appropriate aldehydes 15. The yields range between 81-89% with a significant reduction in the reaction time (Scheme 6).
The formation of the above compounds can be explained according to the following plausible mechanism (Scheme 7).17 The first step is the condensation of malononitrile 14 with aldehyde 15 to yield the intermediate 2-arylidenemalononitrile I. The Michael addition of I to the enaminone 13 affords the intermediate II. This intermediate II undergoes further intramolecular cyclization and dehydration to afford 16ai-cviii.17
Some of the same polysubstituted imidazo[1,2-a]quinoline series 16ai-cviii were recently prepared by a novel approach. The novelty of this protocol is in the use of the nanocrystalline CuO as an efficient catalyst for the following one-pot three-component synthesis (Scheme 8). The advantages of this method can be summarized in being environmentally friendly, run in aqueous medium, and cost efficient. The catalyst is easily recovered and maintains its catalytic activity for several runs.18
A minor route for the synthesis of imidazo[1,2-a]quinolines is the use of N-alkylisatoic anhydrides 17a-c.19 It is well-known that these anhydrides react with active methylene compounds such as 18a-e, to yield the target imidazoquinoline derivatives.19 The reaction proceeds in either acidic (acetic acid - sodium acetate) or basic (DMF/dioxane – TEA) conditions to yield 19a-j and 20a-e (Scheme 9).20 In this approach,20 the synthesis of the above-mentioned imidazo[1,2-a]quinolines is based on the so-called “domino reaction”.21 The utilization of a domino reaction protocol allowed efficiently preparing complex molecules from simple precursors in an economic and ecologically sensitive manner.21 This cascade tandem reaction can simply be defined as a consecutive series of intramolecular organic reactions proceeding via highly reactive intermediates A and B, in which the subsequent transformation takes place at the functionalities obtained in the former transformation.
Recently, a general, simple and effective method was reported for the preparation of the imidazo[1,2-a]quinolines. Compounds 23a-c were prepared in a five-step one-pot protocol method starting from 21a-c, respectively.22 This method can be outlined as having two main parts; an activation step, and a five-step cascade annulation reaction. The activation step is achieved via the reaction of the quinoline derivatives with 1,2-dibromoethane to enhance the electrophilicity of the pyridine ring. The resulting 2-bromoethylquinolinium bromide derivatives 22a-c undergo a five-step reaction to yield compounds 23a-c in 65-100% yields (Scheme 10).22
1.1.2. IMIDAZO[1,5-a]QUINOLINES
The chemistry of imidazo[1,5-a]quinoline 24 is not extensively studied. Through a few reported synthetic procedures, the quinoline-2-carbonitrile (25) was considered as the most common precursor.
Kim et al.23 reported the preparation of substituted imidazo[1,5-a]quinolines. In this process, Reissert compound, such as 1-acyl-1,2-dihydroquinoline-2-carbonitrile 25a,24 has been developed for the preparation of allyl-substituted imidazo[1,5-a]quinolines 26 and 27 via the indium-mediated Barbier type allylation and dehydrative cyclization protocol (Scheme 11).23
The quinolinylglycinate esters have also been introduced as a novel synthetic building blocks for the preparation of imidazo[1,5-a]quinolines.25 In this regard, the methyl (quinolin-2-yl)glycinate (28) was heated at reflux with dimethylformamide dimethyl acetal (DMFDMA) or dimethylacetamide dimethyl acetal (DMADMA) mixtures to afford this fused system or its 1-methyl analog 29a,b, respectively, as shown in Scheme 12.
In the course of preparing novel fused tetracyclic imidazoquinoline derivatives, the benz[f]imidazo[1,5-a]quinolines 32 and the benz[h]imidazo[1,5-a]quinolines 33 were prepared from the corresponding 1-aminomethylbenzo-fused quinolines 30 and 31, respectively, using a similar synthetic route (Scheme 13).26
With the aim of establishing a low-cost, in situ, analytical method for monitoring the levels of contamination of groundwater with chloroform, compounds 21 and 36 were prepared. In this related approach, the 2-(aminomethyl)quinoline 34 and its 4-methoxy derivatives 35 were reacted with chloroform, adopting a basic phase-transfer catalysis technique, to yield the highly fluorescent products 21 and 36, respectively (Scheme14).27
Sasaki and his co-workers reported the preparation of imidazo[1,5-a]quinolines 38 and 39 in a one-step reaction, adopting the Vilsmeier reaction conditions (Scheme 15).28 In this context, the Vilsmeier reagent acts as a one-carbon unit donor.
It is worth mentioning that 21a was reported to form 24 in 25% yield via the reaction with dilithiotosylmethyl isocyanide (40) in a single step reaction29 (Scheme 16).
1.1.3. 1H-IMIDAZO[4,5-c]QUINOLINES
Derivatives of 1H-imidazo[4,5-c]quinolines 41 have proven to possess diverse biological activities. Subsequently, the syntheses of various substituted-1H-imidazo[4,5-c]quinolines have been reported since the 1950’s.30 A perusal of the literature has revealed that anthranilic acid (43) is the most common precursor for the synthesis of imidazo[4,5-c]quinolines. Other less common approaches have also been reported. Such precursors, besides anthranilic acid, have been introduced with the aim of either exploring different methodologies31 or introducing different fuctionalilities at different positions on the nucleus.32 Consequently, the following general scheme for the synthesis of compound 49, starting from anthranilic acid, is suggested as depicted in Scheme 17.
The nitromethane reacts with a strong base, e.g. NaOH, to form methazonic acid or 2-nitroacetaldehyde oxime in situ.33 This oxime when reacted with anthranilic acid affords the 2-(2-nitroethylideneamino)benzoic acid (44).33,34 It is worth mentioning that this reaction is conducted completely under ice cooling to avoid the potential hazards of heating nitromethane.34 This is considered as an essential modification from the originally reported procedure where the reaction was to be completed by heating up to 55 °C.3 The cyclization of compound 44 using acetic anhydride / sodium acetate mixture leads to the formation of 3-nitro-4-hydroxyquinoline (45).33 Compound 45 is converted to the corresponding halo derivative 46 upon heating at reflux with POCl3,35-39 or with SOCl2.32a The nucleophilic substitution reaction of this compound with ammonia35-37 or other secondary amines32a,38,39 affords compounds 47 and 48, respectively. The reduction of the nitro group was reported via different techniques; catalytic hydrogenation with 5% Pd/C,35,36,38,40 Pt/C,32a nickel(II) chloride hexahydrate (NiCl2.6H2O) and sodium borohydride (NaBH4)32b or Raney nickel38. Having the diaminoquinoline derivatives 42a-b in hand enables the imidazole ring cyclization in the proceeding step to obtain the desired products 49ai-biv. According to the type of the ring formed, a diverse variety of reagents can be used, e.g. aliphatic carboxylic acids,35 aromatic carboxylic acids/HMPA,36 different carboxylic acids/PPA37 or HATU,39,41 different ortho esters,32a-b,37,38 or the respective aldehydes/sodium pyrosulphate (Na2S2O7)32c to afford the imidazole ring either with or without substitution on the 2-position (ZX = N=CH, N=CR’). The 2-oxoimidazole ring (ZX = NHC=O) can be formed through reaction with CDI34 or diphosgene.38 Also, the 2-mercaptoimidazole derivatives (ZX = N=CSH) can be prepared via the reaction with CS2.34,42
1.1.4. 3H-IMIDAZO[4,5-f]QUINOLINES
Studying the possible retrosynthetic pathways for the 3H-imidazo[4,5-f]quinoline 50, in relation to the reported synthetic approaches, revealed two major pathways (Figure 7). Those pathways are classified according to the type of ring to be formed;
i) Pathway a: Imidazole-ring formation
ii) Pathway b: Pyridine-ring formation
1.1.4.1. Pathway a: Imidazole-ring formation
The precursor for this pathway is 5,6-diaminoquinoline (51), which can be obtained through the reduction of the nitro group of compound 52 via different approaches.43-48 As a general protocol, compound 51 can be reacted with different reagents, e.g. carboxylic acids,49-51 aldehydes43 or different cyclizing agents, e.g. cyanogen bromide,44,45 or carbon disulfide,45 under varying conditions to afford the 3H-imidazo[4,5-f]quinoline ring system 50 and other related derivatives 50a (Scheme 18).
It is worth mentioning that compound 50 was reported to be synthesized directly from 52 in a two-in-one, one-pot protocol using iron powder and formic acid (Scheme 19).46
In 1980, 2-amino-3-methylimidazo[4,5-f]quinoline (IQ), its 2-amino-3,4-dimethylimidazo[4,5-f]quinoline analogue (Me-IQ) (Figure 8) and other structurally-related compounds were isolated as carcinogenic pyrolysate products from protienaceous foods.52 Pertinent to such findings, several studies have been carried out.
In order to verify the final structure and establish a detailed biological profile for such group of compounds, many synthetic trials have been reported.44,45,53-56 In this context, Lee et al. were able to synthesize azaaromatic mutagenic ring systems in a simple high-yielding procedure.54 Starting with 6-aminoquinoline (55a) or its 7-methyl analogue 55b, the N-methylated derivatives 56a,b were prepared. In this context, formylation of compounds 55a,b followed by reduction with LiAlH4 afforded the target 6-(methylamino)quinoline derivatives 56a,b. The selective mononitration of 56a,b at position 5 was achieved using conc. H2SO4 – conc. HNO3 mixture. The diaminoquinoline derivatives 58a,b, produced from the reduction of the nitro group, were reacted with cyanogen bromide to yield IQ and Me-IQ in 35% and 30% yields, respectively (Scheme 20).
Furthermore, in 1994, Ronne and his co-workers synthesized IQ, Me-IQ and many structurally-related compounds 60a-d with the aim of establishing a detailed structure-activity relationships study for such health hazardous products.45 The small-scale synthesis of the compounds were achieved via two pathways starting from the same precursor, 57a. The first approach was to react the in situ-formed diaminoquinoline derivative directly with cyanogen bromide to afford IQ. The reaction of IQ with tert-butyl hydroperoxide afforded its methylated derivatives, Me-IQ, and 60a-d in 27–47% yields, respectively (Scheme 21, Method A). The second approach was introduced to avoid the use of the highly toxic, hazardous reagent cyanogen bromide according to the method of Ziv et al.,56 but with certain modifications. This multi-step synthetic pathway was to prepare the 2-mercaptoimidazo[4,5-f]quinoline 59 as a center for amination through varying routes (Scheme 21, Methods B, C, & D).45
In continuation of the detailed studies on the mutagenicity and carcinogenicity of IQ and Me-IQ, the 2-amino-3,6-dihydro-3-methyl-7H-imidazo[4,5-f]quinolin-7-one (HOIQ) was identified as a metabolite of IQ. In contrast to IQ, HOIQ is an active, potent, direct-acting mutagenic metabolite produced by the action of human intestinal flora on IQ. The preparation of HOIQ is outlined in Scheme 22.44
1.1.4.2. Pathway b: Pyridine-ring formation
The cornerstone precursor for this pathway is the 5-aminobenzimidazole (53). The general pathway for the quinoline ring formation can start with the catalytic reduction of 5-nitrobenzimidaole 54 to afford the corresponding amino derivative 53. The pyridine-ring cyclization is achieved through the adaptation of Skraup’s/Doebner–von Miller57 or Conrad – Limpach57 methods for quinoline synthesis. The condensation of the resulting amino group with different unsaturated carbonyl derivatives, e.g. acrolein,58,59 crotonaldehyde,47,48,60 methyl propenyl ketone,48 or different β-ketoesters48, 61, 62 followed by cyclization, yields the imidazo[4,5-f]quinolines 50 or the substituted analogues 64-66, respectively (Scheme 23). It is worth mentioning that if the 4(7)-position of the benzimidazole ring -where cyclization occurs - is occupied (i.e. R ≠ H), the linear imidazo[4,5-g]quinolines will be formed instead.47
As a minor synthetic pathway, the imidazo[4,5-f]quinoline 69 was also reported to be prepared through a ring-transformation strategy.63 The imidazo[4,5-f]quinoline 69 was formed through the course of studying the stability of 2,2,4-trialkyl-2,3-dihydro-1H-[1,4]diazepino[2,3-f]quinolines (67) after treatment with acidic or at elevated-temperature conditions.63 A plausible mechanism for this reaction is outlined in Scheme 24. Under thermal conditions, the ring transformation takes place through a 1,3-hydrogen shift followed by an alkyne group and a bulky alkane elimination to afford compound 69. On the other hand, under acidic conditions, the diazepine 67 is first protonated and then undergoes a stepwise loss of alkyne, proton and finally a bulky alkane. Such ring contraction accompanied by a bulky group loss has been previously reported.64
Another minor pathway is to begin with 5-hydrazinoquinoline (70) as a starting material. In this approach, the heterocyclic cyanamide 71a or its acetyl derivative 71b undergo a smooth rearrangement under thermal conditions to afford the 2-aminoimidazo[4,5-f]quinolines 72a,b (Scheme 25).65 This reaction is suggested to happen via a [3.3] sigmatropic rearrangement of either compound 71a or 71b to form the intermediate 71c, followed by an intramolecular cyclization, aromatization, and a hydrogen shift.
1.1.5. 3H-IMIDAZO[4,5-h]QUINOLINES
In general, 3H-imidazo[4,5-h]quinoline (73) can be prepared through two successive ring-closure procedures; the closure of the quinoline ring, followed by the imidazole ring. Consequently, the quinoline ring was reported to be synthesized via Skraup’s reaction protocol from either 3-chloroaniline (75)59 or the 3-nitro analogue 7666,67 to afford 75a and 76a, respectively. Accordingly, different procedures for introduction of the 7,8-diamino functionalities to the quinoline nucleus were investigated, to afford 74. The nitration of 75a followed by aminolysis afforded compound 75b.59 This aminonitroquinoline derivative 75b was reduced using catalytic hydrogenation to yield compound 74.59 On the other hand, the 7-nitroquinoline 76a was reacted with hydroxylamine to introduce the 8-amino functionality and afford the 8-amino-7-nitroquinoline analogue 76b.67,68 Alternatively, the protection of 76aa66 with toluenesulfonyl chloride followed by nitration afforded 76ab.66 The deprotection of 76ab proceeded by reduction of the nitro group yielded compound 74 in an improved procedure66 (Scheme 26).
This 7,8-diaminoquinoline derivative 74 is considered as the building block for the imidazole ring-closure step. The reaction of 74 either with nitriles in the presence of polyphosphoric acid at 250 °C59,69,70 or formic acid / HCl yielded the desired ring system 73a-g or 73, respectively (Scheme 27).
1.1.6. 4H-and 6H-IMIDAZO[4,5,1-ij]QUINOLINES
Theoretically, from a retrosynthetic point of view, 8-aminoquinoline nuclei 79a,b should be the most common precursors for the synthesis of this class of compounds, as shown in Figure 10. On the contrary, the reaction of 8-amino-1,2-dihydroquinoline (79a) with formic or acetic acid was reported to afford only the formylated and the acetylated derivatives 79aa and 79ab, respectively (Scheme 28).71
An alternative approach for the preparation of compounds possessing the 4H-imidazo[4,5,1-ij] quinoline skeleton 77 was reported through the reaction of 8-triphenylphosphoiminoquinoline 81 with aryl isocyanates and aryl aldehydes to yield the series 82a,b and 83a-c, respectively (Scheme 29).72
Besson et al. have reported a novel approach for the preparation of the 2-cyano-4H-imidazo[4,5,1-ij]quinoline-4-thiones 85a-d through a pericyclic reaction. The quinolinic nitrogen atom participates in this reaction with the formation of the imidazole ring and the insertion of a sulphur atom in 4-position of the delineated ring. Those thiones (85a-d) are easily oxidized to their corresponding 4-one derivatives 86a-d upon stirring with cupric nitrate in acetic anhydride at room temperature (Scheme 30).73
A plausible mechanism for this thermo-rearrangement reaction is explained by the contribution of the quinoline nitrogen as a nearby nucleophile to divert the reaction to the imidazoquinolinium species I. This species could form a tetracyclic intermediate II to deliver the sulfur atom to the 2-position on the quinoline ring. Elimination of a hydrogen chloride and sulfur from II leads to the formation of the desired ring system 86b (Scheme 31).73
Furthermore, the synthesis of 1,2-dihydro-4H-imidazo[4,5,1-ij]quinoline-2,4-dione (89a) and 4H-imidazo[4,5,1-ij]quinolin-2-one (89b) have been reported to proceed either via the thermolysis of the ethyl N-(2-oxo-1,2-dihydroquinolin-8-yl)carbamate derivative 8774 or the reaction of the phenyl carbamate 88 with sodium borohydride respectively.75 It is worth mentioning that the thermolysis of 87 is associated with the elimination of an ethanol molecule. In case of the phenyl carbamate 88, the ring cyclization is facilitated through the addition of the hydride to the 2-position of the quinoline ring with the elimination of a phenoxide ion (Scheme 32).
Preparation of 6-oxo-6H-imidazo[4,5,1-ij]quinoline-4-carboxylic acid methyl ester (97) and 5-carboxylic acid ethyl esters 98a,b were reported to proceed via the corresponding 8-amino-4-oxo-1,4-dihydroquinoline-2- and -2,3-(di)carboxylates 95 and 96a,b, respectively. Stepwise total syntheses of compounds 97 and 98a,b are illustrated in Schemes 33 and 34, respectively. Namely, reacting the 2-nitroaniline (90a) with dimethyl acetylenedicarboxylate (91) (as illustrated in Scheme 33) or 90a and its 4-methyl derivative 90b with diethyl ethoxymethyenemalonate 92 (as illustrated in Scheme 34) afforded the 2-substituted quinolin-4-one 93 and the 3-substiuted quinolin-4-ones 94a,b, respectively. Reduction of the 8-nitro group on the quinoline ring followed by the reaction with triethyl orthoformate afforded compounds 97 and 98a,b, respectively.76
2.1. LINEAR IMIDAZOQUINOLINES
2.1.1. 1H-IMIDAZO[4,5-b]QUINOLINES
Investigating the retrosynthetic routes for this imidazoquinoline (99) lead us to two main possibilities; namely, a central pyridine-ring closure of compound 100 (Pathway a, Figure 11) or imidazole-ring formation from compound 10167 (Pathway b, Figure 11). In reality, Pathway a is the most common, well-accessed protocol while none of the reported procedures refers to making use of Pathway b as a synthetic approach. Other minor pathways, e.g. ring contraction,77 and Beckmann rearrangement,78 have also been reported. Accordingly, the synthesis of imidazo[4,5-b]quinoline-2-one scaffold 106, as an example for this imidazoquinolines, is described in the following general scheme. The synthetic plan starts with the introduction of an imidazole ring to the substituted 2-nitrobenzyl halides 102 or substituted 2-nitrobenzaldehydes 103 by implementing different methods, e.g. A / B or C / D, respectively. The reduction of the nitro group of the hydantoin derivatives 104 is reported to afford 105. Finally, the dehydrative cyclization and aromatization of 105 yields the imidazoquinolines 106 (Scheme 35).79-81
In a similar manner, anthranilic acid (43) or its substituted derivatives 107a,b were used for the preparation of the imidazo[4,5-b]quinoline-2,4-diones 109a-c. The reaction of diphenylthiohydantoin 108 with 43 and 107a,b in refluxing methanol yielded the imidazo[4,5-b]quinolin-2,9-dione series 109a-c in 70, 75 and 67% yields, respectively (Scheme 36).82
Other derivatives, e.g. 1-aminoimidazo[4,5-b]quinolin-2-one (110)77 and 9-hydroxy derivative 11378 were reported to be prepared via short synthetic pathways. Compound 110 was isolated as an unexpected ring contraction product of 1,2,4-triazine moiety 111 under slightly acidic conditions. The structure was confirmed through an independent preparation from the open-ring precursor 112 (Scheme 37).77
In Scheme 38, the 9-hydroxyimidazo[4,5-b]quinoline derivative 113 was prepared via a Beckmann rearrangement mechanism. This microwave-assisted one-pot reaction of hydroxylamine hydrochloride with 3-acetyl-2-(propylamino)-1,4-dihydroquinolin-4-one (114) yielded 113 in 75% yield, along with other minor products.78
2.1.2. 3H-IMIDAZO[4,5-g]QUINOLINES
Pertinent to the already discussed synthetic strategies, the imidazo[4,5-g]quinolines can be prepared using either from 6,7-diaminoquinoline (116) or the 4-substiuted-5-aminobenzimidazole 117, as starting materials, as shown in Figure 12. In the second case, blocking of position 4 (R ≠ H) is necessary to prevent the formation of the angular imidazo[4,5-f]quinolines.
Scheme 39 illustrates the two approaches for the preparation of the parent imidazo[4,5-g]quinoline 115.83 The utilization of other typical quinoline synthetic approaches, e.g. Gould–Jacobs, can lead to the formation of 5,8-dihydroimidazo[4,5-g]quinolin-8-one 123 (Scheme 40).84
An effective procedure using a Diels–Alder methodology has been developed for the synthesis of 4,9-dihydroimidazo[4,5-g]quinolin-4,9-dione derivatives 126a,b and 127a,b (Scheme 41). The regiochemistry of the cycloadditions of azadiene 124 with quinones 125a,b agrees with the calculations of the semi–empirical method PM3.85
CONCLUSION
The imidazoquinolines are fully synthetic scaffolds with diverse biopharmaceutical applications that are mainly attributed to the variable possible positions of fusion between the imidazole and quinoline rings. Interestingly, their members possess activities ranging from being potent carcinogens to being effective anticancer chemotherapeutic agents. Accordingly, a growing area of research is directly related to the development of new synthetic methodologies and strategies for these significant scaffolds.
In general, this fused heterocyclic system can be prepared via two major synthetic plans: pyridine-ring formation followed by the imidazole one or vice versa. According to the type of imidazoquinolines and the nature of substituents, either one of these two synthetic strategies can be adopted. Nevertheless, this field is still in need of future work to improve the methodologies and to implement new approaches to achieve clean, cost-effective, efficient, large-scaled products, as well as to explore and discover novel biological and pharmaceutical applications.
References
1. V. Milata, ‘Advances in Heterocyclic chemistry: Tricyclic Azoloquinolines,’ ed. by A. R. Katritzky, Academic Press, San Diego, California, 2000, pp. 189-268.
2. A. Omodei-Sale, E. Toia, G. Galliani, and L. J. Lerner, 1976, Ger. Offen. 2551868 (Chem. Abstr., 1976, CAN 85: 192731).
3. H. Inaba, K. Nishijima, K. Kato, I. Yamamoto, E. Mochida, and K. Ohtomo, 1991, Eur. Pat. Appl. 405442 (Chem. Abstr., 1991, CAN 115: 29328).
4. I. R. Ager, A. C. Barnes, G. W. Danswan, P. W. Hairsine, D. P. Kay, P. D. Kennewell, S. S. Matharu, P. Miller, P. Robson, D. A. Rowlands, W. R. Tully, and R. Westwood, J. Med. Chem., 1988, 31, 1098. CrossRef
5. R. J. Williams, Expert Opin. Ther. Targets, 2000, 4, 313. CrossRef
6. a) N. A. Meanwell, P. Hewawasam, J. A. Thomas, J. J. K. Wright, J. W. Russell, M. Gamberdella, H. J. Goldenberg, S. M. Seiler, and G. B. Zavoico, J. Med. Chem., 1993, 36, 3251; CrossRef b) N. A. Meanwell, B. C. Pearce, H. R. Roth, E. C. R. Smith, D. L. Wedding, J. J. K. Wright, J. O. Buchanan, U. M. Baryla, M. Gamberdella, E. Gillespie, D. C. Hayes, S. M. Seiler, H. C. Stanton, G. B. Zavoico, and J. S. Fleming, J. Med. Chem., 1992, 35, 2672; CrossRef c) J. S. Fleming, J. O. Buchanan, S. M. Seiler, and N. A. Meanwell, Thromb. Res., 1991, 63, 145; CrossRef d) N. A. Meanwell, H. R. Roth, E. C. R. Smith, D. L. Wedding, J. J. K. Wright, J. S. Fleming, and E. Gillespie, J. Med. Chem., 1991, 34, 2906. CrossRef
7. E. Mandrea, 2006, U.S. Pat. 0183767 (Chem. Abstr., 2006, CAN 145: 241694).
8. H. Hennig, J. Tauchnitz, G. Koehler, and W. Schindler, J. Prakt. Chem., 1976, 317, 853 (Chem. Abstr., 1976, CAN 84: 61157).
9. M. Fujita, H. Egawa, M. Kataoka, T. Miyamoto, J. Nakano, and J.-I. Matsumoto, Chem. Pharm. Bull., 1995, 43, 2123. CrossRef
10. a) F. Lacarrubba, M. C. Potenza, S. Gurgone, and G. Micali, J. Dermatological Treat., 2011, 22, 353; CrossRef b) A. Carta, I. Briguglio, S. Piras, P. Corona, G. Boatto, M. Nieddu, P. Giunchedi, M. E. Marongiu, G. Giliberti, F. Iuliano, S. Blois, C. Ibba, B. Busonera, and P. La Colla, Bioorg. Med. Chem., 2011, 19, 7070. CrossRef
11. P. J. M. Van Galen, P. Nissen, I. Van Wijngaarden, A. P. Ijzerman, and W. Soudijn, J. Med. Chem., 1991, 34, 1202. CrossRef
12. I. R. Ager, A. C. Barnes, G. W. Danswan, P. W. Hairsine, D. P. Kay, P. D. Kenewell, S. S. Matharu, P. Miller, P. Robonson, D. A. Rowlands, W. R. Tully, and R. Westwood, J. Med. Chem., 1988, 31, 1098. CrossRef
13. S. Clements-Jewery, G. Danswan, C. R. Gardner, S. S. Martharu, R. Murdoch, W. R. Tully, and R. Westwood, J. Med. Chem., 1988, 31, 1220. CrossRef
14. A. L. Krasovsky, V. G. Nenajdenko, and E. S. Balenkova, Synthesis, 2002, 10, 1379. CrossRef
15. S. M. Ireland, H. Tye, and M. Whittaker, Tetrahedron Lett., 2003, 44, 4369. CrossRef
16. S. R. Inglis, R. K. Jones, G. W. Booker, and S. M. Pyke, Bioorg. Med. Chem. Lett., 2006, 16, 387. CrossRef
17. S. Tu, C. Li, G. Li, L. Cao, Q. Shao, D. Zhou, B. Jiang, J. Zhou, and M. Xia, J. Comb. Chem., 2007, 9, 1144. CrossRef
18. S. J. Ahmadi, M. Hosseinpour, and S. Sadjadi, Synth. Commun., 2011, 42, 426. CrossRef
19. a) G. M. Coppola, Synthesis, 1980, 505; CrossRef b) G. M. Coppola, J. Heterocycl. Chem., 1985, 22, 1087; CrossRef c) G. M. Coppola, J. Heterocycl. Chem., 1986, 23, 1717; CrossRef d) G. M. G. M. Coppola, J. Heterocycl. Chem., 1992, 29, 1873; CrossRef e) G. M. Coppola, Synthesis, 1983, 939; CrossRef f) G. M. Coppola, Synthesis, 1988, 81. CrossRef
20. R. T. Iminov, A. V. Tverdokhlebov, A. A. Tolmachev, Y. M. Volovenko, A. N. Kostuk, A. N. Chernega, and E. B. Rusanov, Synthesis, 2008, 1535. CrossRef
21. a) L. F. Tietze and N. Rackelmann, Pure Appl. Chem., 2004, 76, 1967; CrossRef b) A. Padwa, Chem. Comm., 1998, 1417.
22. A. D. C. Parenty, Y.-F. Song, C. J. Richmond, and L. Cronin, Org. Lett., 2007, 9, 2253. CrossRef
23. S. H. Kim, Y. M. Kim, B. R. Park, and J. N. Kim, Bull. Korean Chem. Soc., 2010, 31, 3031. CrossRef
24. a) M. Takamura, K. Funabashi, M. Kanai, and M. Shibasaki, J. Am. Chem. Soc., 2000, 122, 6327; CrossRef b) M. Takamura, K. Funabashi, M. Kanai, and M. Shibasaki, J. Am. Chem. Soc., 2001, 123, 6801; CrossRef c) J. S. Yadav, B. V. S. Redey, M. Srinivas, and K. Sathaiah, Tetrahedron Lett., 2005, 46, 3489. CrossRef
25. a) P. Kolar, A. Petrie, and M. Tisler, J. Heterocycl. Chem., 1991, 28, 1715; CrossRef b) P. Kolar and M. Tisler, Z. Naturforsch., 1991, 46, 1110.
26. H. Zimmer, Chimia, 1972, 26, 131.
27. K. C. Langry, J. Org. Chem., 1991, 56, 2400. CrossRef
28. K. Sasaki, A. Tsurumori, and T. Hirota, J. Chem. Soc., Perkin Trans. 1, 1998, 3851. CrossRef
29. S. P. J. M. Van Nispen, C. Mensink, and A. M. Van Leusen, Tetrahedron Lett., 1980, 21, 3723. CrossRef
30. G. B. Bachman, G. E. Bennett, and R. S. Barker, J. Org. Chem., 1950, 15, 1278. CrossRef
31. H. Yoshioka, Y. Matsuya, T. Choshi, E. Sugino, and S. Hibino, Chem. Pharm. Bull., 1996, 44, 709. CrossRef
32. a) J. F. Gerster, K. J. Lindstrom, R. L. Miller, M. A. Tomai, W. Birmachu, S. N. Bomersine, S. J. Gibson, L. M. Imbertson, J. R. Jacobson, R. T. Knafla, P. V. Maye, N. Nikolaides, F. Y. Oneyemi, G. J. Parkhurst, S. E. Pecore, M. J. Reiter, L. S. Scribner, T. L. Testerman, N. J. Thompson, T. L. Wagner, C. E. Weeks, J.-D. Andre, D. Lagain, Y. Bastard, and M. Lupu, J. Med. Chem., 2005, 48, 3481; CrossRef b) T. Izumi, J. Sakagughi, M. Takeshita, H. Tawara, K.-I. Kato, H. Dose, T. Tsujino, Y. Watanabe, and H. Kato, Bioorg. Med. Chem., 2003, 11, 2541; CrossRef c) T. Shiro, H. Takahashi, K. Kakiguchi, Y. Inoue, K. Masuda, H. Nagata, and M. Tobe, Bioorg. Med. Chem. Lett., 2012, 22, 285; CrossRef d) N. M. Shukla, C. A. Mutz, R. Ukani, H. J. Warshakoon, D. S. Moore, and S. A. David, Bioorg. Med. Chem. Lett., 2010, 20, 6384; CrossRef e) N. M. Shukla, T. C. Lewis, T. P. Day, C. A. Mutz, R. Ukani, C. D. Hamilton, R. Balakrishna, and S. A. David, Bioorg. Med. Chem. Lett., 2011, 21, 3232; CrossRef f) F. Suzuki, T. Kuroda, H. Hayashi, Y. Nakasato, H. Manabe, K. Ohmori, and S. Kitamura, Chem. Pharm. Bull., 1992, 40, 3245; CrossRef g) Y. Ikuma and H. Nakahira, Tetrahedron, 2011, 67, 9509. CrossRef
33. G. B. Bachman, D. E. Welton, G. L. Jenkins, and J. E. Christian, J. Am. Chem. Soc., 1947, 69, 365. CrossRef
34. Z. Mahmoud (2012). 1H-Imidazo[4,5-c]quinoline Derivatives as Potential Bioactive Molecules, Synthesis and Biological Evaluation. (PhD thesis). Memorial University of Newfoundland, St. John’s Campus, St. John’s, NL, Canada.
35. A. P. Ijzerman, P. J. M. Van Galen, H. W. Th. Van VJijmen, W. Soudjin, P. Nissen, and I. Van Wijngaarden, Nucleotide Nucleosides, 1991, 10, 1039.
36. S. Takada, T. Sasatani, N. Chomei, M. Adachi, T. Fujishita, M. Eigyo, S. Murata, K. Kawasaki, and A. Matsushita, J. Med. Chem., 1996, 39, 2844. CrossRef
37. A. Göblyös, Z.-G. Gao, J. Brussee, R. Connestari, S. N. Santiago, K. Ye, A. P. IJzerman, and K. A. Jacobson, J. Med. Chem., 2006, 49, 3354. CrossRef
38. F. Stauffer, S.-M. Maira, P. Furet, and C. Garcia-Echeverria, Bioorg. Med. Chem. Lett., 2008, 18, 1027. CrossRef
39. N. M. Shukla, M. R. Kimbrell, S. S. Malladi, and S. A. David, Bioorg. Med. Chem. Lett., 2009, 19, 2211. CrossRef
40. Y. Kim, S. de Castro, Z.-G. Gao, A. P. Ijzerman, and K. A. Jacobson, J. Med. Chem., 2009, 52, 2098. CrossRef
41. N. M. Shukla, S. S. Malladi, C. A. Mutz, R. Balakrishna, and S. A. David, J. Med. Chem., 2010, 53, 4450. CrossRef
42. E. Capkauskaite, L. Baranauskiene, D. Golovenko, E. Manakova, S. Grazulis, S. Tumkevicius, and D. Matulis, Bioorg. Med. Chem., 2010, 18, 7357. CrossRef
43. a) A. P. R. Reddy and V. Veeranagaiah, Indian J. Chem., 1985, 24B, 372; b) A. A. Aleksandrov, A. S. Dedeneva, E. V. Vlasova, and M. M. El’chaninov, Russian J. Org. Chem., 2011, 47, 120. CrossRef
44. M. Bashir and D. G. I. Kingston, Heterocycles, 1987, 26, 2877. CrossRef
45. E. Ronne, S. Grivas, and K. Olsson, Acta Chem. Scand., 1994, 48, 823. CrossRef
46. E. J. Hanan, B. K. Chan, A. A. Estrada, D. G. Shore, and J. P. Lyssikatos, Synlett, 2010, 18, 2759. CrossRef
47. S. Ishiwata and Y. Shiokawa, Chem. Pharm. Bull., 1969, 17, 1153. CrossRef
48. S. Ishiwata and Y. Shiokawa, Chem. Pharm. Bull., 1969, 17, 2455. CrossRef
49. A. M. Simonov, B. I. Khristich, and V. G. Poludnenko, Khim. Geterotsikl., 1967, 5, 927 (Chem. Abstr., 1968, CAN 68: 114499).
50. A. P. R. Reddy and V. Veeranagaiah, Indian J. Chem., 1984, 23B, 673.
51. B. Rajitha, M. K. Roa, and P. N. Reddy, Indian J. Chem., 2004, 43B, 417.
52. a) T. Kosuge, K. Tsuji, K. Wakabayashi, T. Okamoto, K. Shudo, Y. Iitaka, A. Itai, T. Sugimura, T. Kawachi, M. Nagao, T. Yahagi, and Y. Seino, Chem. Pharm. Bull., 1978, 26, 611; CrossRef b) T. Yamamto, K. Tsuji, T. Okamoto, K. Shudo, K. Takeda, Y. Iitaka, K. Yamauchi, Y. Seino, T. Yahagi, M. Nagao, and T. Sugimura, Proc. Japan Acad., 1978, 54B, 248; CrossRef c) K. Wakabayashi, K. Tsuji, T. Kosuge, K. Takeda, K. Yamauchi, K. Shudo, Y. Iitaka, T. Okamoto, T. Yahagi, M. Nagao, and T. Sugimura, Proc. Japan Acad., 1978, 54B, 569; CrossRef d) H. Kasai, Z. Yamaizumi, S. Nishimura, K. Wakabayashi, M. Nagao, T. Sugimura, N. E. Spingarn, J. H. Weisburger, S. Yokoyama, and T. Miyazawa, J. Chem. Soc., Perkin Trans. 1, 1981, 2290; CrossRef e) W. A. Hargraves and M. W. Pariza, Cancer Res., 1983, 43, 1467.
53. H. Kasai and S. Nishimura, Bull. Chem. Soc. Jpn., 1982, 55, 2233. CrossRef
54. C.-S. Lee, Y. Hashimoto, K. Shudo, and T. Okamoto, Chem. Pharm. Bull., 1982, 30, 1857. CrossRef
55. A. L. Waterhouse and H. Rapoport, J. Labelled Compd. Rad., 1985, 22, 201. CrossRef
56. J. Ziv, S. Knapp, and J. D. Rosen, Synth. Commun., 1988, 18, 973. CrossRef
57. J.-J. Li and E. J. Corey, (eds). ‘Name Reactions in Heterocyclic Chemistry’, (Chapter 9), New Jersey, John Willey and Sons, Inc, 2005.
58. E. Lebenstedt and W. Schunack, Arch. Pharm., 1974, 307, 894. CrossRef
59. L. G. Moores, R. K. Smalley, and H. Suschitzky, J. Fluorine Chem., 1982, 20, 573. CrossRef
60. A. El kihel, M. Ahbala, M. El Ouar, and N. Knouzil, Phys. Chem. News, 2005, 24, 112.
61. C. F. Spencer, H. R. Snyder, Jr., and R. J. Alaimo, J. Heterocycl. Chem., 1975, 12, 1319. CrossRef
62. A. El kihel, M. El Ouar, M. Ahbala, A. Mouzdahir, T. Harjane, and N. Knouzil, Arabian J. Chem., 2010, 3, 9.
63. A. P. Reddy and V. Veeranagaiah, Indian J. Chem., 1989, 28B, 272.
64. D. Lloyd, R. H. Mcdougall, and D. R. Marshall, J. Chem. Soc., 1965, 3785. CrossRef
65. M. T. V. L. Carvalho, A. M. Lobo, P. S. Branco, and S. Prabhakar, Tetrahedron Lett., 1997, 38, 3115. CrossRef
66. F. Linsker and R. Evans, J. Am. Chem. Soc., 1946, 68, 149. CrossRef
67. K. S. Sharma, S. Kumari, and R. P. Singh, Synthesis, 1981, 316. CrossRef
68. M. Colonna and F. Montanari, Gazz. Chim. Ital., 1951, 81, 744 (Chem. Abstr., 1952, CAN 46: 42308).
69. F. H. Case, J. Heterocycl. Chem., 1967, 4, 157. CrossRef
70. F. H. Case and L. Kennon, J. Heterocycl. Chem., 1967, 4, 483. CrossRef
71. A. Richardson Jr. and E. D. Amstutz, J. Org. Chem., 1960, 25, 1138. CrossRef
72. K. Nagamatsu, E. Akiyoshi, H. Ito, H. Fujii, A. Kakehi, and N. Abe, Heterocycles, 2006, 69, 167. CrossRef
73. T. Besson, C. W. Rees, D. G. Roe, and V. Tiery, J. Chem. Soc., Perkin Trans. 1, 2000, 555. CrossRef
74. M. Hamana and S. Kumadaki, Chem. Pharm. Bull., 1974, 22, 1506. CrossRef
75. W. Saari, W. Halczenko, M. B. Freedman, and B. H. Arison, J. Heterocycl. Chem., 1982, 19, 837. CrossRef
76. N. P. Peet, L. E. Baugh, S. Sunder, and J. E. Lewis, J. Med. Chem., 1985, 28, 298. CrossRef
77. J. Slouka, V. Bekarek, K. Napela, and A. Lycka, Coll. Czech. Chem. Commun., 1984, 49, 2628. CrossRef
78. B. H. Hwang, E. B. Choi, H. K. Lee, H. C. Yang, B. Y. Chung, and C. S. Pak, Synthesis, 2008, 22, 3569. CrossRef
79. N. A. Meanwell, H. R. Roth, E. C. R. Smith, D. L. Wedding, J. J. K. Wright, J. S. Fleming, and E. Gillespie, J. Med. Chem., 1991, 34, 2906. CrossRef
80. N. A. Meanwell, R. D. Dennis, H. R. Roth, M. J. Rosenfeld, E. C. R. Smith, J. J. K. Wright, J. O. Buchanan, C. L. Brassard, M. Gamderdella, E. Gillespie, S. M. Seiler, G. B. Zavoico, and J. S. Fleming, J. Med. Chem., 1992, 35, 2688. CrossRef
81. Z. Zhu, B. Lippa, and L. B. Townsend, J. Org. Chem., 1999, 64, 4159. CrossRef
82. A. M. Sh. El-Sharief, A. M. Hussein, M. S. A. El-Gaby, A. A. Atalla, and A. A. Ahmed, Phosphorus Sulfur, 2001, 170, 47. CrossRef
83. E. Lebenstedt and W. Schunack, Arch. Pharm., 1975, 308, 977. CrossRef
84. A. Carta, G. Boatto, G. Paglietti, G. Poni, M. G. Sctzu, and P. Caredda, Heterocycles, 2003, 60, 833. CrossRef
85. F. Alvarez, A. Taleb, J. Gentili, P. Nebois, R. Terreux, M. Domard, A. Thozet, D. Merle, H. Fillion, and N. Walchshofer, Eur. J. Org. Chem., 2005, 1903.
86. R. M. Black, 1977, GB 1488412 (Chem. Abstr., 1977, CAN 88: 121177).