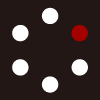
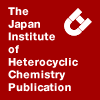
HETEROCYCLES
An International Journal for Reviews and Communications in Heterocyclic ChemistryWeb Edition ISSN: 1881-0942
Published online by The Japan Institute of Heterocyclic Chemistry
e-Journal
Full Text HTML
Received, 26th June, 2012, Accepted, 8th August, 2012, Published online, 21st August, 2012.
DOI: 10.3987/COM-12-12533
■ Suzuki-Miyaura Coupling Reactions of 3,5-Dichloro-1,2,4-thiadiazole
Abdelbasset A. Farahat and David W. Boykin*
Department of Chemistry, Georgia State University, P.O. Box 4098, Atlanta, Georgia 30302-4098, U.S.A.
Abstract
3,5-Dichloro-1, 2, 4-thiadiazole was allowed to react with different aryl boronic acids under different Suzuki-Miyaura coupling conditions: at room temperature 5-aryl-3-chloro-1, 2, 4-thiadiazoles were obtained and at toluene reflux temperature the products were 3,5-diaryl-1, 2, 4-thiadiazoles. Sequential coupling reactions lead to 3,5-diaryl-1, 2, 4-thiadiazoles with non-identical aryl groups. The structure of 3-methoxy-5-(4-methoxyphenyl) -1, 2, 4-thiadiazole was established from X-ray crystallographic data.INTRODUCTION
The divergent and key roles played by substituted 1,2,4-thiadiazoles in a wide variety of biological applications illustrates the importance of this heterocyclic system and are highlighted in two recent reviews.1,2 The role played by the 1,2,4-thiadiazole unit includes that of reactive warhead in irreversible enzyme inhibitors,3 a scaffold role in more conventional enzyme inhibition4 and binding to human muscarinic receptors.5 More recently, 1,2,4-thiadiazoles have been optimized as inhibitors of inducible nitric oxide synthase and have shown efficacy against acute inflammation and chronic neuropathic pain,6 have shown affinity for insect muscarinc receptor(s) and hold promise as insecticides7 and have been demonstrated to have chemoprotective potential as a result of inhibition of aromatase and NF-κB.8 The synthetic methodology employed to make these various systems is limited.
RESULTS AND DISCUSSION
A majority of these biologically important molecules have different substitutents at the 3 and 5 positions. Such molecules are most often made by convergent synthesis and the thiadiazole unit is generated by either intra- or intermolecular cyclization reactions.1,9 1,2,4-Thiadiazoles with identical substitutents at positions 3 and 5 are frequently prepared by one of a plethora of methods of oxidative dimerization of thioamides.1,8b,10 Most approaches to make either the 1,2,4-thiadiazoles with dissimilar or identical substitutents are not convenient for the rapid generation of analogues which is highly desirable for medicinal chemistry studies. A very attractive strategy employed by Wehn and coworkers starts with intact 1,2,4-thiadiazoles, presents significant versatility and provides access to both types of substituted 1,2,4-thiadiazoles.11 This approach involved as the key step optimization of Suzuki-Miyaura methodology for the reaction of protected 5-amino-3-bromo-1,2,4-thiadiazoles to ultimately obtain 5-amino-3-aryl-1,2,4-thiadiazoles. In the same study, the optimized conditions, using bis(di-tert-butyl(4-dimethylaminophenyl)phosphine)dichloropalladium(II) in dioxane at 80 oC, obtained for coupling the protected 5-amino-3-bromo-1,2,4-thiadiazole was applied to the Suzuki-Miyaura coupling of arylboronic acids with 3-bromo-5-chloro-1,2,4-thiadiazole as a means to ultimately obtain the regioisomeric 5-aryl-3-amino-1,2,4-thiadiazoles. This investigation demonstrated that the regioselectivity of Suzuki-Miyaura coupling is at the 5-chloro group as opposed to the 3-bromo group which is counter to the normal reactivity of halogens.11 The observed regioselectivity is consistent with a frontier molecular orbital analysis first described by Houk and coworkers12 and employed by Wehn.11
As part of a drug discovery effort we had need of a convenient approach for preparation of 3,5-di-(cyanoaryl)-1,2,4-thiadiazoles for both classes of compounds; those with identical aryl groups and those with non-identical ones. Consequently, we decided to explore the Suzuki-Miyaura coupling reactions of 3,5-dichloro-1,2,4-thiadiazole (1). First, we investigated the synthesis of thiadiazoles with identical substituents at positions 3 and 5 by using 2.2 equivalents of aryl boronic acids. Subsequently, we explored sequential coupling reactions using 1.1 equivalents of aryl boronic acids in each step. This report describes the results of these investigations.
Seeking to obtain 3,5-di(aryl)-1,2,4-thiadiazoles with identical 3,5-substitutents we employed conventional Suzuki-Miyaura coupling using a tetrakis(triphenylphosphine) palladium (0), K2CO3 catalyst system with toluene/water/methanol as the solvent heated at reflux.13 As a qualitative gage of the reactivity of 1 under these conditions in our exploratory study we used the typically lower yielding 4-methoxyphenylboronic acid as the coupling partner (Scheme 1). Under these conditions we isolated, using silica gel column chromatography, the desired 3,5-bis(4-methoxyphenyl)-1,2,4-thiadiazole (2a) in a 55% yield. In addition, two other thiadiazoles were isolated in low yields (ca. 5% each). One was shown to be 3-chloro-5-(4-methoxyphenyl)-1,2,4-thiadiazole (3) consistent with the expected regioselectivity for introduction of an aryl group.11 The second low yield thiadiazole was shown to be 5-methoxy-3-(4-methoxyphenyl)-1,2,4-thiadiazole (4) which is counter to the expected regioselectivity for introduction of an aryl group. In addition, a 5% yield of 4,4’-dimethoxybiphenyl the product of homocoupling of the boronic acid was isolated. The yield of 2a was satisfactory for our purpose of making 3,5-diaryl-1,2,4-thiadiazoles with identical 3,5-substitutents. However, the formation of 4 under these conditions provides a cautionary note for our desire to use a sequential approach to make 3,5-aryl-1,2,4-thiadiazoles with non-identical 3,5-substitutents which will be addressed in a later section of this manuscript.
After obtaining an acceptable yield of 2a as described above we proceeded to prepare a series of identically substituted 3,5-di(cyanoaryl)-1,2,4-thiadiazoles (2b-2h) (Scheme 2). The isolated yields of the bis-nitriles are generally around 60% and the compounds were characterized by NMR spectroscopy, mass spectroscopy and elemental analysis. During the preparation of the bis-nitriles 2b-2h we were unable to isolate compounds corresponding to 4. Therefore, we hypothesized that perhaps electron donating groups on the arylboronic acids were required to obtain analogues of 4. Consequently, we performed coupling reactions between 1 and phenyl-, 4-methylphenyl- and 4-dimethylaminophenyl-boronic acid under the conditions previously described. In the case of the reaction involving phenylboronic acid to yield 2i we were unable to detect an analogue of 4. However, in the case of the reactions of both 4-methylphenyl- and 4-dimethylaminophenyl-boronic acid to form 2j and 2k, respectively, we were able to isolate, in low yields chromatographic fractions (ca. 5%) which gave proton NMR data which were consistent with being a mixture of the 3-chloro-5-aryl-1,2,4-thiadiazole and 5-methoxy-3-aryl-1,2,4-thiadiazole. Unfortunately, we were unable to separate either of these mixtures. We hypothesize, in the three cases in which we have evidence of formation of 5-methoxy-3-aryl-1,2,4-thiadiazole, that at reflux in toluene/methanol/water a small amount of 5-chloro-3-aryl-1,2,4-thiadiazole (the product not predicted by frontier molecular orbital analysis) is formed which then reacts rapidly with methanol to yield the 5-(methoxy)-3-(aryl) 1,2,4-thiadiazole.
Prior to attempting to make thiadiazole bis-nitriles which had non-identical groups at positions 3 and 5 it was essential to confirm the structure 4 since its regioisomer, 3-methoxy-5-(4-methoxyphenyl)-1,2,4-thiadiazole, was the more likely product based on theoretical grounds.11,12 We decided to attempt to obtain a X-ray crystal structure of 4 to provide unambiguous structural evidence. Unfortunately, we were unable to obtain suitable crystals of 4, therefore we prepared its isomer, 3-methoxy-5-(4-methoxyphenyl)- 1,2,4-thiadiazole (5). In view of the formation of 4 at elevated temperatures (Scheme 1), we decided to evaluate the Suzuki-Miyaura coupling reaction at room temperature as a possible means to enhance the regioselectivity at position-5. We were pleased to find that after stirring at room temperature for 24 h with 1.1 equivalent boronic acid, the reaction proceeded to give a 75% yield of 3-chloro-5-(4-methoxyphenyl)-1,2,4-thiadiazole (3) (Scheme 3).
We note that after performing the reaction at room temperature with 2.2 equivalent boronic acid, we obtained mostly the mono analogue and not more than 5% yield of the bis-aryl analogue. The two isomeric compounds 4 and 5 were not identical as demonstrated by their melting points (including a depressed mixed melting point), 1H NMR, and 13C NMR data. Fortunately, we were able to obtain crystals of 5 which were suitable for X-ray analysis and the results from that study are found in Figure 1 and in Table 1. Figure 1 shows an ORTEP representation of the crystal structure for 5, which by elimination confirms the structure of 3. Table 1 contains selected bond lengths and angles for 5.
In order to make the non-identical 3,5-di(cyanoaryl)-1,2,4-thiadiazoles sequential coupling reactions with 1.1 equivalent of arylboronic acid in each step were explored. In the first step we performed the reaction at room temperature as described for Scheme 3 and for the second step we used the reflux temperature reaction conditions described in Schemes 1 and 2 to make the two different isomeric bis-nitriles 7a and 7b in good yields (Scheme 4).
The synthesis, in reasonable yields, of 3,5-diaryl-1,2,4-thiadiazoles with identical aryl groups using Suzuki-Miyaura coupling, employing the widely used catalyst tetrakis(triphenylphosphine)palladium (0), at reflux has been described. Under these conditions compound 3 was isolated in low yields, which is counter to the regiochemistry predicted by frontier molecular orbital analysis.11,12 The structure of 3 was verified by determination of the X-ray structure of its isomer 5. Synthesis of 3,5-di(aryl)-1,2,4-thiadiazoles with non-identical aryl groups has been achieved in good yields, using tetrakis(triphenylphosphine)palladium (0), by a sequential process conducted at room temperature in the first step and by performing the second step by heating at reflux. The methodology described here provides ready access to both types of 3,5-di(aryl)-1,2,4-thiadiazoles which should be useful for future medicinal chemistry studies.
EXPERIMENTAL
All commercial reagents were used without purification. Melting points were determined on a Mel-Temp 3.0 melting point apparatus, and are uncorrected. TLC analysis was carried out on silica gel 60 F254 precoated aluminum sheets using UV light for detection. 1H and 13C NMR spectra were recorded on a Bruker 400 MHz spectrometer using the indicated solvents. Mass spectra were obtained from the Georgia State University Mass Spectrometry Laboratory, Atlanta, GA. Elemental analysis were performed by Atlantic Microlab Inc., Norcross, GA. X-ray crystallography was performed at the X-ray Crystallography Center, Department of Chemistry, Emory University, Atlanta, GA.
General procedure for the synthesis of 3,5-bis(aryl)-1,2,4-thiadiazole (2a-k)
Deaerated 2 M aqueous solution of K2CO3 (10 mL) and arylboronic acids (11 mmol) in deaerated MeOH (10 mL) were added to a stirred solution of 3,5-dichloro-1,2,4-thiadiazole 1 (5 mmol), and tetrakis(triphenylphosphane)palladium (0) (0.5 mmol) in deaerated toluene (30 mL) under a nitrogen atmosphere. The reaction mixture was heated at reflux for 24 h. The solvent was evaporated under reduced pressure, the solid was partitioned between ethyl acetate (200 mL) and 2 M aqueous Na2CO3 (25 mL) containing concentrated ammonia (5 mL), then washed with water, passed through celite to remove the catalyst, dried (sodium sulfate) and evaporated. The product was purified by column chromatography on silica gel, using hexanes/EtOAc (90/10-50/50 v/v).
3,5-Bis(4-methoxyphenyl)-1,2,4-thiadiazole (2a)
White solid 0.82 g, (55%), mp 138-140 °C (lit.,10a mp 137-138 °C); 1H NMR (DMSO-d6): δ = 3.85 (s, 3 H), 3.88 (s, 3 H), 7.10-7.16 (m, 4 H), 8.07 (d, J = 8 Hz, 2 H), 8.25 (d, J = 8 Hz, 2 H); 13C NMR (DMSO-d6): δ = 54.8, 55.1, 113.8, 114.4, 122.0, 124.6, 128.7, 129.0, 160.6, 161.9, 171.9, 186.7; ESI-MS: m/z calculated for C16H14N2O2S: 298.35, found: 299.00 [M+ + 1].
3,5-Bis(4-cyanophenyl)-1,2,4-thiadiazole (2b)
White solid 0.88 g, (61%), mp > 255 °C dec. (lit.,8a mp > 260 °C dec.); 1H NMR (DMSO-d6): δ = 8.01 (d, J = 8.4 Hz, 2 H), 8.07 (d, J = 8.4 Hz, 2 H), 8.32 (d, J = 8.4 Hz, 2 H), 8.49 (d, J = 8.4 Hz, 2 H); 13C NMR (DMSO-d6): δ = 112.3, 114.0, 117.6, 118.0, 127.3, 128.2, 131.0, 132.4, 133.5, 135.4, 170.8, 186.4; ESI-MS: m/z calculated for C16H8N4S: 288.32, found: 289.05 (M+ + 1).
3,5-Bis(3-cyanophenyl)-1,2,4-thiadiazole (2c)
White solid 0.85 g, (59%), mp 181-182 °C (lit.,8a mp 176-177 °C); 1H NMR (DMSO-d6): δ = 7.83 (d, J = 8.4 Hz, 2 H), 8.04 (br s, 1 H), 8.14 (d, J = 8.4 Hz, 1 H), 8.48 (s, 1 H), 8.60 (s, 1 H), 8.71 (m, 2 H); 13C NMR (DMSO-d6): δ = 112.0, 113.5, 117.6, 117.9, 127.0, 128.0, 131.2, 132.6, 133.0, 135.0, 170.8, 186.4; ESI-MS: m/z calculated for C16H8N4S: 288.32, found: 289.05 (M+ + 1).
3,5-Bis(2-cyanopyrid-5-yl)-1,2,4-thiadiazole (2d)
White solid 0.87 g, (59%), mp > 260 °C dec.; 1H NMR (DMSO-d6): δ = 7.56 (m, 3 H), 7.64 (d, J = 7.6 Hz, 1 H), 7.66 (d, J = 8.0 Hz, 1 H), 7.68 (d, J = 7.6 Hz, 1 H), 7.80 (s, 1 H); ESI-MS: m/z calculated for C14H6N6S: 290.30, found: 291.10 (M+ + 1). Anal. Calcd for C14H6N6S: C, 57.92; H, 2.08; N, 28.95. Found: C, 57.89; H, 2.17; N, 28.66.
3,5-Bis(4-cyano-2-methylphenyl)-1,2,4-thiadiazole (2e)
White solid 0.91 g, (58%), mp 201-202 °C; 1H NMR (DMSO-d6): δ = 2.73 (s, 3H), 2.82 (s, 3H), 7.80 (br s, 1 H), 7.87 (br s, 2 H), 7.96 (s, 1 H), 8.20 (d, J = 8.0 Hz, 1 H), 8.28 (d, J = 8.0 Hz, 1 H); 13C NMR (DMSO-d6): δ = 26.0, 27.9, 112, 114.1, 117.5, 118.9, 128.3, 129.7, 130.1, 131.0, 133.3, 134.5, 134.6, 135.2, 137.9, 139.0, 17.01, 184.9; ESI-MS: m/z calculated for C18H12N4S: 316.37, found: 317.41 (M+ + 1). Anal. Calcd for C18H12N4S: C, 68.33; H, 3.82; N, 17.71. Found: C, 68.25; H, 3.66; N, 17.42.
3,5-Bis(4-cyano-3-methylphenyl)-1,2,4-thiadiazole (2f)
White solid 0.94 g, (59%), mp 213-215 °C; 1H NMR (DMSO-d6): δ = 2.69 (s, 3H), 2.75 (s, 3H), 7.80 (br s, 1 H), 7.91 (m, 4 H), 8.28 (d, J = 8.4 Hz, 1 H); 13C NMR (DMSO-d6): δ = 28.1, 29.0, 112.3, 113.4, 117.5, 117.7, 129.2, 129.7, 130.1, 131.0, 133.0, 134.5, 134.7, 135.2, 137.9, 138.5, 171.0, 184.9; ESI-MS: m/z calculated for C18H12N4S: 316.37, found: 317.40 (M+ + 1). Anal. Calcd for C18H12N4S: C, 68.33; H, 3.82; N, 17.71. Found: C, 68.21; H, 3.71; N, 17.33.
3,5-Bis(4-cyano-2-fluorophenyl)-1,2,4-thiadiazole (2g)
White solid 1.0 g, (63%), mp 198-200 °C; 1H NMR (DMSO-d6): δ = 7.56 (br s, 2 H), 7.62 (d, J = 7.2 Hz, 1 H), 7.66 (d, J = 7.2 Hz, 1 H), 7.68 (br s, 2 H); ESI-MS: m/z calculated for C16H6 F2N4S: 324.30, found: 325.10 (M+ + 1). Anal. Calcd for C16H6 F2N4S: C, 59.26; H, 1.86; N, 17.28. Found: C, 59.43; H, 1.88; N, 17.01.
3,5-Bis(4-cyano-3-fluorophenyl)-1,2,4-thiadiazole (2h)
White solid 0.90 g, (55%), mp 179-180 °C; 1H NMR (DMSO-d6): δ = 7.51 (m, 3 H), 7.62 (d, J = 7.2 Hz, 1 H), 7.65 (br s, 2 H); ESI-MS: m/z calculated for C16H6 F2N4S: 324.30, found: 325.10 (M+ + 1). Anal. Calcd for C16H6 F2N4S: C, 59.26; H, 1.86; N, 17.28. Found: C, 59.37; H, 1.84; N, 16.97.
3,5-Bis(phenyl)-1,2,4-thiadiazole (2i)
White solid 0.63 g, (52%), mp 99-100 °C (lit.,10a mp 91 °C); 1H NMR (DMSO-d6): δ = 7.60-7.66 (m, 6 H), 8.12 (d, J = 7.2 Hz, 2 H), 8.31 (d, J = 7.2 Hz, 2 H); 13C NMR (DMSO-d6): δ = 112.0, 117.0, 118.6, 128.4, 131.1, 132.3, 150.3, 152.1, 172.4, 186.5; ESI-MS: m/z calculated for C14H10N2S: 238.30, found: 239.11 [M+ + 1].
3,5-Bis(4-methylphenyl)-1,2,4-thiadiazole (2j)
White solid 0.76 g, (57%), mp 123-124 °C (reported mp 124-125 °C)10a; 1H NMR (DMSO-d6): δ = 2.39 (s, 3 H), 2.41 (s, 3 H), 7.31 (d, J = 8.0 Hz, 2 H), 7.42 (d, J = 8.0 Hz, 2 H), 8.00 (d, J = 8.0 Hz, 2 H), 8.14 (d, J = 8.0 Hz, 2 H); 13C NMR (DMSO-d6): δ = 21.3, 21.5, 111.2, 116.7, 119.9, 125.6, 128.2, 128.6, 151.0, 152.2, 172.4, 186.5; ESI-MS: m/z calculated for C16H14N2S: 266.36, found: 267.09 [M+ + 1].
3,5-Bis(4-(N,N-dimethylamino)phenyl)-1,2,4-thiadiazole (2k)
White solid 0.80 g, (50%), mp 233-235 °C (lit.,14 mp 222-224 °C); 1H NMR (DMSO-d6): δ = 3.15 (s, 6 H), 3.27 (s, 6 H), 6.78-6.82 (m, 4 H), 7.86 (d, J = 8.4 Hz, 2 H), 8.10 (d, J = 8.4 Hz, 2 H); 13C NMR (DMSO-d6): δ = 32.0, 32.5, 111.1, 118.0, 118.3, 125.9, 128.2, 128.7, 150.3, 152.1, 172.4, 186.5; ESI-MS: m/z calculated for C18H20N4S: 324.44, found: 325.13 [M+ + 1].
3-Chloro-5-(4-methoxyphenyl)-1,2,4-thiadiazole (3)
White solid 0.057 g, (5%), mp 90-92 °C; 1H NMR (DMSO-d6): δ = 3.87 (s, 3 H), 7.13 (d, J = 8.4 Hz, 2 H), 7.98 (d, J = 8.4 Hz, 2 H); 13C NMR (DMSO-d6): δ = 55.2, 114.5, 120.1, 128.7, 155.4, 162.6, 189.2; ESI-MS: m/z calculated for C9H7ClN2OS: 226.68, found: 227.03[M+ + 1]. Anal. Calcd for C9H7ClN2OS: C, 47.69; H, 3.11; N, 12.36. Found: C, 47.65; H, 3.12; N, 12.31.
5-Methoxy-3-(4-methoxyphenyl)-1,2,4-thiadiazole (4)
White solid 0.055 g, (5%), mp 73-74 °C; 1H NMR (DMSO-d6): δ = 3.82 (s, 3 H), 4.23 (s, 3 H), 7.06 (d, J = 8 Hz, 2 H), 8.07 (d, J = 8 Hz, 2 H); 13C NMR (DMSO-d6): δ = 54.8, 60.4, 113.3, 124.7, 128.3, 160.5, 166.1, 190.8; ESI-MS: m/z calculated for C10H10N2O2S: 222.26, found: 223.00 [M+ + 1]. Anal. Calcd. For C10H10N2O2S: C, 54.04; H, 4.53; N, 12.60. Found: C, 54.14; H, 4.53; N, 12.58.
3-Methoxy-5-(4-methoxyphenyl)-1,2,4-thiadiazole (5)
K2CO3 (8 mmol) was added to a stirred solution of 3-chloro-5-(4-methoxyphenyl)-1,2,4-thiadiazole 3 (4 mmol) in MeOH (25 ml). The reaction mixture was heated at reflux for 24 h. The solvent was evaporated under reduced pressure, the residual solid was purified by column chromatography on silica gel, using hexanes/EtOAc (90/10, v/v). White solid 0.83 g, (95%), mp 89-90 °C; 1H NMR (DMSO-d6): δ = 3.85 (s, 3 H), 4.04 (s, 3 H), 7.12 (d, J = 8.0 Hz, 2 H), 7.92 (d, J = 8.0 Hz, 2 H); 13C NMR (DMSO-d6): δ = 55.1, 56.6, 114.4, 121.8, 128.1, 162.1, 171.3, 187.1; ESI-MS: m/z calculated for C10H10N2O2S: 222.26, found: 223.00 [M+ + 1]. Anal. Calcd for C10H10N2O2S: C, 54.04; H, 4.53; N, 12.60. Found: C, 54.18; H, 4.53; N, 12.48.
General procedure for the synthesis of 3-chloro-5-aryl-1,2,4-thiadiazoles (3 and 6a,b)
Deaerated 2 M aqueous solution of K2CO3 (5 mL) and arylboronic acids (5.5 mmol) in 5 mL deaerated MeOH were added to a stirred solution of 3,5-dichloro-1,2,4-thiadiazole (5 mmol), and tetrakis(triphenylphosphane)palladium(0) (0.25 mmol) in deaerated toluene (20 mL) under a nitrogen atmosphere. The reaction mixture was stirred at room temperature for 24 h. The solvent was evaporated under reduced pressure, the solid was partitioned between EtOAc (100 mL) and 2 M aqueous Na2CO3 (15 mL) containing concentrated ammonia (5 mL), then washed with water, passed through celite to remove the catalyst, dried (sodium sulfate) and evaporated. The product was purified by column chromatography on silica gel, using hexanes/ EtOAc (95/5-85/15, v/v).
3-Chloro-5-(4-methoxyphenyl)-1,2,4-thiadiazole (3)
White solid 0.85 g, (75%); mp and other data as described in the previous section.
3-Chloro-5-(4-cyanophenyl)-1,2,4-thiadiazole (6a)
White solid 0.86 g, (77%), mp 110-111 °C; 1H NMR (DMSO-d6): δ = 7.43 (br s, 2 H), 7.57 (br s, 2 H); 13C NMR (DMSO-d6): δ = 112.6, 118.6, 130.3, 131.1, 135.5, 162.3, 187.8; ESI-MS: m/z calculated for C9H4ClN3S: 221.67, found: 222.00 [M+ + 1]. Anal. Calcd for C9H4ClN3S: C, 48.77; H, 1.82; N, 18.96. Found: C, 48.69; H, 1.81; N, 18.99.
3-Chloro-5-(3-cyanophenyl)-1,2,4-thiadiazole (6b)
White solid 0.83 g, (76%), mp 115-117 °C; 1H NMR (DMSO-d6): δ = 7.80-7.83 (m, 1H), 8.15 (d, J = 8.0 Hz, 1 H), 8.37 (d, J = 8.0 Hz, 1 H), 8.55 (br s, 1H); 13C NMR (DMSO-d6): δ = 112.2, 117.9, 130.8, 132.0, 135.9, 163.2, 187; ESI-MS: m/z calculated for C9H4ClN3S: 221.67, found: 222.00 [M+ + 1]. Anal. Calcd For C9H4ClN3S: C, 48.77; H, 1.82; N, 18.96. Found: C, 49.12; H, 1.85; N, 18.67.
General procedure for the synthesis of 3,5-Bis(3- or 4-cyanophenyl)-1,2,4-thiadiazole (7a, b)
Deaerated 2 M aqueous solution of K2CO3 (5 mL) and arylboronic acids (5.5 mmol) in deaerated MeOH (5 mL) were added to a stirred solution of 6a or 6b (5 mmol), and tetrakis(triphenylphosphane)palladium(0) (0.25 mmol) in deaerated toluene (20 mL) under a nitrogen atmosphere. The reaction mixture was refluxed for 24 h. The solvent was evaporated under reduced pressure, the solid was partitioned between EtOAc (100 mL) and 2 M aqueous Na2CO3 (15 mL) containing concentrated ammonia (5 mL), to remove palladium, then washed with water, passed through celite to remove the catalyst, dried (sodium sulfate) and evaporated. The product was purified by column chromatography on silica gel, using hexanes/ EtOAc (50/50 v/v).
3-(3-Cyanophenyl)-5-(4-cyanophenyl)-1,2,4-thiadiazole (7a)
White solid 1.05 g, (71%), mp 231-233 °C; 1H NMR (DMSO-d6): δ = 7.77 (br s, 1 H), 8.12 (m, 3 H), 8.47 (br s, 2 H), 8.53 (s, 1 H), 8.65 (s, 1 H); 13C NMR (DMSO-d6): δ = 112.6, 114.0, 117.6, 117.9, 127.8, 128.1, 129.7, 131, 132.7, 133.0, 135.0, 138.0, 170.8, 186.4; ESI-MS: m/z calculated for C16H8N4S: 288.32, found: 289.05 (M+ + 1). Anal. Calcd for C16H8N4S: C, 66.65; H, 2.80; N, 19.43. Found: C, 66.37; H, 2.79; N, 19.14.
5-(3-Cyanophenyl)-3-(4-cyanophenyl)-1,2,4-thiadiazole (7b)
White solid 1.0 g, (69%), mp >250 °C dec.; 1H NMR (DMSO-d6): δ = 7.83-7.84 (m, 4 H), 8.01-8.06 (m, 4 H); 13C NMR (DMSO-d6): δ = 113.0, 113.5, 117.9, 118.0, 127.0, 129,129.9, 131.0, 133.0, 133.1, 134.2, 134.6, 170.1, 186.0; ESI-MS: m/z calculated for C16H8N4S: 288.32, found: 289.05 (M+ + 1). Anal. Calcd for C16H8N4S: C, 66.65; H, 2.80; N, 19.43. Found: C, 66.26; H, 2.71; N, 19.38.
CRYSTAL STRUCTURE DATA
A suitable single crystal of 5 (from acetone) was selected from the sample and mounted onto a nylon fibre with paratone oil and placed under a cold stream at 110 K. Single crystal X-ray data were collected on a Bruker APEX2 diffractometer with 1.6 kW graphite monochromated Mo radiation. The detector to crystal distance was 5.1 cm. Exposure times of 20s per frame and scan widths of 0.5º were used throughout the data collection. The data collection was performed using a combination of 5 sets of ω scans with different φ values yielding data in theta range 1.67 to 29.57° with an average completeness of 98.4%. The frames were integrated with SAINT v7.68a (Bruker, 2009).15 A combination of multi-scan absorption and a numerical correction was carried out using the program SADABS V2008-1 (Bruker, 2008).16 The structure was solved and refined with Olex217 and SHELX (Sheldrick, 2008).18 In the final cycles of refinement all non-hydrogen atoms were refined anisotropically. The crystal structure is chiral while the molecule has approximate Cm symmetry. The absolute configuration could be determined unequivocally from the diffraction.
Crystallographic data for compound 5 have been deposited with accession number CCDC879278 and can be obtained free of charge from Cambridge Crystallographic Data Centre, 12 Union Road, Cambridge CB2 1EZ, UK: +44(1223)336033; E-mail: deposit@ccdc.cam.ac.uk; Web site: www.ccdc.cam.ac.uk/const/retrieving.html
ACKNOWLEDGEMENTS
This work was supported by an award from the Bill and Melinda Gates Foundation and by NIH grant AI064200. We thank Dr. John Bacsa, Emory University X-ray Crystallography Center, for obtaining the crystal structure.
References
1. A. Castro, T. Castano, A. Encinas, W. Porcal, and C. Gil, Bioorg. Med. Chem., 2006, 14, 1644. CrossRef
2. T. F. Tam, R. Leung-Toung, W. Li, M. Spino, and K. Karimain, Mini-Reviews Med. Chem., 2005, 5, 367.
3. (a) R. Leung-Toung, J. Wodzinska, W. Li, J. Lowrie, R. Kukreja, D. Desilets, K. Karimian, and T. F. Tam, Bioorg. Med. Chem., 2003, 11, 5529; CrossRef (b) C. Marrano, P. de Macedo, P. Gagnon, D. Lapierre, C. Gravel, and J. W. Keillor, Bioorg. Med. Chem., 2001, 9, 3231. CrossRef
4. (a) A. Castro, A. Encinas, C. Gil, S. Brase, W. Porcal, C. Perez, F. J. Moreno, and A. Martinez, Bioorg. Med. Chem., 2008, 16, 495; CrossRef (b) T. Kondo, T. Nekado, I. Sugimoto, K. Ochi, S. Takai, A. Kinoshits, A. Hatayama, S. Yamamoto, K. Kishikawa, H. Nakai, and M. Toda, Bioorg. Med. Chem., 2008, 16, 1613. CrossRef
5. F. R. Tejada, P. I. Nagy, M. Xu, C. Wu, T. Katz, J. Dorsey, M. Rieman, E. Lawlor, M. Warrier, and W. S. Messer, Jr., J. Med. Chem., 2006, 49, 7518. CrossRef
6. T. C. Gahman, M. K. Herbert, H. Lang, A. Thayer, K. T. Symons, P. M. Nguyen, M. E. Massari, S. Dozier, Y. Zhang, M. Sablad, T. S. Rao, S. A. Noble, A. K. Shiau, and C. A. Hassig, Bioorg. Med. Chem. Lett., 2011, 21, 6888. CrossRef
7. J. Suzuki, D. Okamura, T. Gushikawa, K. Hirai, and T. Ando, J. Pestic. Sci., 2011, 36, 392. CrossRef
8. (a) A. S. Mayhoub, L. Marler, T. P. Kondratyuk, E.-J. Park, J. M. Pezzuto, and M. Cushman, Bioorg. Med. Chem., 2012, 20, 510; CrossRef (b) A. S. Mayhoub, L. Marler, T. P. Kondratyuk, E.-J. Park, J. M. Pezzuto, and M. Cushman, Bioorg. Med. Chem., 2012, 20, 2427. CrossRef
9. M. Reuman, Z. Hu, G-H. Kuo, X. Li, R. K. Russell, L. Shen, S. Youells, and Y. Zhang, Org. Proc. Res. Dev., 2007, 11, 1010. CrossRef
10. (a) P. C. Patil, D. S. Bhalerao, P. S. Dangate, and K. G. Akamanchi, Tetrahedron Lett., 2009, 50, 5820; CrossRef (b) A. A. Shah, Z. A. Khan, N, Choudhary, C. Loholter, S. Schafer, G. P. L. Marie, U. Farooq, B. Witulski, and T. Wirth, Org. Lett., 2009, 11, 3578. CrossRef
11. P. M. Wehn, P. E. Harrington, and J. E. Eksterowicz, Org. Lett., 2009, 11, 5666. CrossRef
12. (a) Y. Garcia, F. Schoenebeck, C. Y. Legault, C. A. Merlic, and K. N. Houk, J. Am. Chem. Soc., 2009, 131, 6632; CrossRef (b) C. Y. Legault, Y. Garcia, C. A. Merlic, and K. N. Houk, J. Am. Chem. Soc., 2007, 129, 12664. CrossRef
13. (a) A. A. Farahat, A. Kumar, M. Say, A. M. Barghash, F. E. Goda, H. M. Eisa, T. Wenzler, R. Brun, Y. Liu, L. Mickelson, W. D. Wilson, and D. W. Boykin, Bioorg. Med. Chem., 2010, 18, 557; CrossRef (b) D. Branowska, A. A. Farahat, A. Kumar, T. Wenzler, R. Brun, Y. Liu, W. D. Wilson, and D. W. Boykin, Bioorg. Med. Chem., 2010, 18, 3551; CrossRef (c) A. A. Farahat, E. Paliakov, A. Kumar, M. Say, A. M. Barghash, F. E. Goda, H. M. Eisa, T. Wenzler, R. Brun, Y. Liu, W. D. Wilson, and D. W. Boykin, Bioorg. Med. Chem., 2011, 19, 2156. CrossRef
14. H. Z. Boeini, J. Iran. Chem. Soc., 2009, 6, 547.
15. Bruker SAINT Version 7.68a; Bruker AXS Inc.: Madison (WI, USA), 2009.
16. Bruker SADABS Version 2008-1; Bruker AXS Inc.: Madison (WI, USA), 2008.
17. O. V. Dolomanov, L. J. Bourhis, R. J. Gildea, J. A. K. Howard, and H. Puschmann, J. Appl. Cryst., 2008, 42, 339. CrossRef
18. G. M. Sheldrick, Acta Cryst., 2008, A64, 112. CrossRef