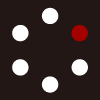
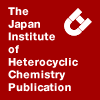
HETEROCYCLES
An International Journal for Reviews and Communications in Heterocyclic ChemistryWeb Edition ISSN: 1881-0942
Published online by The Japan Institute of Heterocyclic Chemistry
e-Journal
Full Text HTML
Received, 6th July, 2012, Accepted, 29th August, 2012, Published online, 11th September, 2012.
DOI: 10.3987/COM-12-12540
■ Competitive Condensation and Tandem Cyclization Reactions of 2-Cyano-3-ferrocenylacrylonitrile with Amidines in an Aqueous Medium
Elena I. Klimova,* Marcos Flores-Alamo, José M. Méndez Stivalet, and Tatiana Klimova
Faculty of Chemistry, National Autonomous University of Mexico, Cd. Universitaria, Coyoacan, C.P. 04510, Mexico
Abstract
The synthesis of a series of ferrocenyl-substituted 2-aminopyridine- and 6-amino-4,5-dihydropyridine-3,5-dicarbonitriles, 4-aminopyrimidine- and 3,4-dihydropyrimidine-5-carbonitriles by reactions of 2-cyano-3-ferrocenylacrylonitrile with amidines in aqueous medium is described. New fused 6-amino-2-ethoxy-4-ferrocenyl-5-ferrocenylmethyl-4,5-dihydropyridine-3,5-dicarbonitrile was prepared in a tricomponent cyclodimerization from 2-cyano-3-ferrocenylacrylonitrile in presence of ethanol. The structures of the obtained compounds were established by IR, 1H and 13C NMR spectroscopy, mass-spectrometry and X-ray diffraction analysis.Ferrocenyl-substituted organic molecules have been immensely exploited for their potential applications in diverse fields, such as organic synthesis,1 medicinal chemistry,2,3 supramolecular chemistry,4 chemo- and biosensors,5 materials science,6 etc. The stability and non toxicity of ferrocenyl group in aqueous and aerobic media has made it an ideal candidate for use in drug designing. Heterocyclic derivatives of ferrocene are the most interesting from the point of view of the biological activity.6-9 Many ferrocenyl-substituted nitrogen heterocycles, such as pyrazole, triazole, pyridazine, pyrimidine, quinuclidine, pyridine, etc., were reported to pertain to biologically active compounds.9-14 Their activity depends on the nature of functional groups and of the heterocycle in the molecules. This stimulates the research on new heterocyclic compounds of the ferrocene series with potential practically useful properties. Therefore, the interest in the synthesis of novel ferrocenyl-containing heterocycles is quite justified. The past years have seen a boost in the synthesis of functionalized ferrocenyl-pyrimidines and pyridines.15,16 However, the approaches to the synthesis of these compounds and their properties remain largely unexplored so far. Among methods used for the synthesis of such kind of compounds, the most common are reactions of ferrocenyl-α,β-enones (1) (Schemes 1 and 2a) or acetylferrocene (3) (Scheme 2b) with amidine derivatives (for synthesis of ferrocenylpyrimidines 2)15-17 or with malononitrile, ethyl cyanoacetate, or with arylcarboxaldehydes in the presence NH4OAc (for synthesis of ferrocenylpyridines 4a,b).18,19
Since the synthetic approach used for obtaining heteryl-ferrocenes plays an important role and is crucial for their possible future application, the aim of our work was to develop a new convenient method of the synthesis of aminocyano(ferrocenyl)pyrimidine and pyridine derivatives by reactions of 2-cyano-3-ferrocenylacrylonitrile with amidines. To the best of our knowledge, the use of 2-cyanoacrylonitniles for the preparation of pyrimidine and pyridine derivatives has not hitherto been documented.
The starting 2-cyano-3-ferrocenylacrylonitrile (7) was prepared in 76% yield by condensation of ferrocenecarbaldehyde (5) with malononitrile (6) (Scheme 3). The physical and 1H NMR spectroscopic characteristics of compound 7 were well in line with the literature data.20
The reaction of 2-cyano-3-ferrocenylacrylonitrile (7) with acetamidine hydrochloride (8a) and benzamidine hydrochloride (8b) was performed in aqueous EtOH in presence of Na2CO3 at ca. 80-85 °C. It was found that this reaction affords ferrocenecarboxaldehyde(5) (∼9-10%), 2-amino-6-ethoxy-4-ferrocenylpyridine-3,5-dicarbonitrile (9) (∼23-27%), 4-amino-2-methyl(or phenyl)-6-ferrocenyl-pyrimidine- 5-carbonitriles (10a) and (10b) (∼9-10%), 6-amino-2-ethoxy-4-ferrocenyl-5-ferrocenylmethyl-4,5-dihydropyridine-3,5-dicarbonitrile (11a) (∼12-15%), 6-amino-4-ferrocenyl-4-ferrocenyl-2-methyl(or phenyl)-3,4-dihydropyrimidine-5-carbonitriles (12a) and (12b) (∼32-33%) (Scheme 4).
Further we found that the reaction of 2-cyano-3-ferrocenylacrylonitrile (7) with 1,1-dimethylguanidinium sulfate (8c) under analogous conditions affords, in contrast to condensations of 7 with 8a and 8b, only four compounds: 5, 9, 10c, 11a (Scheme 4), and the product 12c was not formed in detectable amount. Compounds 5, 9, 10a-c, 11a, and 12a,b were isolated by column chromatography on alumina and their structures were established based on the data from IR and NMR sprctroscopy, mass spectrometry, and elemental analysis (see experimental section). The spatial structures of compounds 9, 11a, and 12b were determined by X-ray diffraction analysis of their single crystals.21 Crystals of 9 and 12b were obtained by crystallization from CHCl3, and crystals of 11a were obtained by crystallization from CH2Cl2 - MeOH (1:1). The general views of molecules 9, 11a, and 12b are shown in Figures 1-3, respectively. The data from X-ray diffraction analysis confirmed the aromatic pyridine and dihydropyridine structures for compounds 9 and 11a, respectively, and the dihydropyrimidine structure for compound 12b. The central fragment of the molecule 9 is a flat six-membered ring with one nitrogen atom. Data from the X-rayanalysis show that the N(1)-C(15) bond in the compound 9 is somewhat shorter [d = 1.320(4) Å] than the standard value of 1.338 Å.22
The C(15)-C(14), C(11)-C(12) and C(12)-C(13) bond lengths are somewhat longer [d = 1.413(4) Å, d = 1.413(5) Å and d = 1.414(5) Å, respectively] than the standard value of 1.339 Å.23 The lengths of the C-Fe and C-C bonds in the ferrocenyl substituents, as well as the geometric parameters of the ferrocene sandwiches are close to standard values.24 The central nonplanar fragment of the molecule 11a is a six-membered ring with one nitrogen atom in the half-chair conformation. The N(1)-C(25), N(1)-C(26), C(23)-C(24) and C(24)-C(25) bond lengths are equal to d = 1.377(3) Å, d = 1.315(3) Å, d = 1.516(3) Å, and d = 1.362(3) Å, respectively. The ferrocenyl and ferrocenylmethyl substituents at C-4 and C-5 have trans-orientation.
The central nonplanar fragment of the molecule 12b is a six-membered ring with two nitrogen atoms in the half-chair conformation. Data from the X-ray analysis show that the N(1)-C(17), N(2)-C(17) and N(3)-C(20) bonds in the dihydropyrimidine are somewhat shorter [d = 1.335(5) Å, d = 1.318(4) Å and d = 1.361 Å, respectively] than the corresponding standard values of d = 1.387 Å, d = 1.355 Å and d = 1.394 Å.23
Although the detailed mechanism of the reaction shown in Scheme 4 requires to be fully clarified, the formation of ferrocenylcarboxaldehyde 5, 2-amino-6-ethoxy-4-ferrocenylpyridine-3,5-dicarbonitrile 9, 4-amino-6-ferrocenylpyrimidine-5-carbonitriles 10a-c and 12a,b can be explained by possible reaction sequence presented in Schemes 5a-c. Compound 5 may be formed via sequential addition of the amidines 8a-c at the double bond FcCH=C of the inicial compound 7 (the Michael addition) resulting in intermediate products 13a-c, further fragmentation of which can lead to aldehyde 5 and malononitrile 6 (Scheme 5a). This type of fragmentation of Cα−Cβ bonds in the ferrocenyl-β-dicarbonilic compouds was well documented in previous publications.35 The intramolecular cyclization of (13a-c) gives dihydropyrimidines 12a,b and aromatic pyrimidines 10a-c (Scheme 5b). The addition between malononitrile 6 and 2-cyano-3-ferrocenylacrylonitrile 7 affords intermediate (14), which upon intramolecular cyclization in the presence of EtOH gives rise to (15) and 9 (Scheme 5c). This type of cyclization represents, in reality, a tricomponent reaction.
Tentative mechanism for the formation of the diferrocenyl(dihydro)pyridine-3,5-dicarbonitriles 11a,b is represented in Scheme 6.
To prove the mechanism described above, the cyclodimerization of 2-cyano-3-ferrocenylacrylonitrile 1 was carried out under identical conditions in isopropyl alcohol in presence of water and Na2CO3. The product of the cyclodimerization, 6-amino-4-ferrocenyl-5-ferrocenylmethyl-2-isopropoxy-4,5-dihydropyridine-3,5-dicarbonitrile 4b, was obtained with ∼ 27% yield. Thus, this cyclodimerization of compound 1 represents a novel type of the three-component anomalous reaction of [4+2]-cycloadition, absolutely different from the reaction of Diels-Alder.
Thus, in the present work, a convenient method of obtaining of 6-alkoxy-2-amino-4-ferrocenylpyridine-3,5-dicarbonitriles, 2-alkoxy-6-amino-4-ferrocenyl-5-ferrocenylmethyl-4,5-dihydropyridine-3,5-dicarbonitriles, 6-amino-4-ferrocenyl-2-(alkyl or aryl)-3,4-dihydropyrimidine-5-carbonitriles and 4-amino-6-ferrocenyl-2-(alkyl or aryl)pyrimidine-5-carbonitriles based on accessible 2-cyano-3-ferrocenylacrylonitrile 7 was developed. This method can be widely used in the synthesis of various ferrocene derivatives. The reactions described in this study will be of interest to synthetic, theoretical and practical organic chemists looking for obtaining functionalized ferrocenylheterocycles.
EXPERIMENTAL
Column chromatography was carried out on alumina (Brockmann activity III). The 1H and 13C NMR spectra were recorded on a Unity Inova Varian spectrometer (300 and 75 MHz) for solutions in CDCl3, with Me4Si as the internal standard. The IR spectra were measured on a Spectrofotometer FT-IR (Spectrum RXI Perkin Elmer instruments) using KBr pellets. The mass spectra were obtained on a Varian MAT CH-6 instrument (EI MS, 70 eV). Elementar Analysensysteme LECO CHNS-900 was used for elemental analyses. The unit cell parameters and the X-ray diffraction intensities were recorded on a Gemini (detector AtlasCCD, Cryojet N2) diffractometer. The structures of compounds 9, 11a and 12b were solved by the direct method (SHELXS-9725).
Tipical procedure of the reactions of 2-cyano-3-ferrocenylacrylonitrile (7) with acetamidine (8a), benzamidine (8b) and 1,1-dimethylguanidine (8c). A mixture of compound 7 (2.26 g, 10 mmol), acetamidine hydrochloride 8a (1.4 g, 15 mmol), benzamidine hydrochloride 8b (2.34 g, 15 mmol) or 1,1-dimethylguanidinium sulfate 8c (2.05 g, 15 mmol), EtOH (100 mL), H2O (10 mL) and 2.0 g Na2CO3 was stirred for 8 h at 80 οC. The solvents were removed in vacuo and the residue was dissolved in CH2Cl2 (50 mL). The solution was mixed with Al2O3 (activity III) (20 g) and the solvent was evaporated in air. This sorbent was applied onto a column with Al2O3 (the height of alumina is ca. 20 cm) and the reaction products were eluted from the column first with petroleum ether, then with a 1:2 CH2Cl2 - petroleum ether and 1:10 CH2Cl2 - MeOH solvent system to give compounds 5 (0.09g, 9% from 8a; 0.11g, 11% from 8b); 0.1g, 10% from 8c), 9, 10a-c, 11a and 12a,b.
2-Amino-6-ethoxy-4-ferrocenylpyridine-3,5-dicarbonitrile (9): yield 0.5g (27.5%, from 8a), 0.4g (22%, from 8b) and 0.42g (23%, from 8c), red crystals, mp 168-169 °C. IR 499, 817, 1006, 1109, 1483, 1555, 1611, 2202, 2218, 2978, 3105, 3226, 3369cm-1; 1H NMR δ 1.43 (t, J = 7.08 Hz, 3H), 4.43 (q, J = 7.08 Hz, 2H), 4.28 (s, 5H), 4.58 (m, 2H), 5.21 (m, 2H), 5.58 (bs, 2H); 13C NMR δ 14.43, 59.10, 64.12, 70.99, 69.62, 69.85, 70.89, 71.10, 84.99, 116.32, 117.68, 160.12, 161.54, 164.88, 167.18, 170.09; MS: m/z 372 [M]+. Anal. Calcd for C19H16FeN4O: C, 61.32; H, 4.33; N, 15.05. Found: C, 61.39; H, 4.42; N, 15.07.
4-Amino-6-ferrocenyl-2-methylpyrimidine-5-carbonitrile (10a): yield 0.32 g (10%), red crystals, 182-183 °C. IR 485, 822, 1000, 1100, 1494, 1568, 1595, 1655, 2167, 2930, 3135, 3323, 3377 cm-1; 1H NMR δ 2.53 (s, 3H), 4.18 (s, 5H), 4.56 (m, 2H), 5.32 (m, 2H), 5.52 bs, 1H); 13C NMR δ 26.00, 69.95, 69.21, 71.06, 79.06, 117.09, 164.28, 168.25, 169.21, 171.45; MS: m/z 318 [M]+. Anal. Calcd. for C16H14FeN4: C, 60.40; H, 4.44; N, 17.60. Found: C, 60.31; H, 4.53; N, 17.58.
4-Amino-6-ferrocenyl-2-phenylpyrimidine-5-carbonitrile (10b): yield 0.34g (9%), red crystals, mp 197-198 °C. IR 483, 821, 1001, 1106, 1461, 1485, 1531, 1615, 2208, 3093, 3223, 3331 cm-1; 1H NMR δ 4.18 (s, 5H), 4.58 (m, 2H), 5.44 (m, 2H), 5.63 (bs, 2H), 7.32-7.72 (m, 5H); 13C NMR δ 70.51, 70.04, 71.92, 79.22, 117.50, 128.55, 128.96, 131.65, 137.01, 137.54, 164.42, 164.70,170.83; MS: m/z 380 [M]+. Anal. Calcd for C21H16FeN4: C, 66.33; H, 4.24; N, 14.73. Found: C, 66.42; H, 4.17; N, 14.65.
4-Amino-2-dimethylamino-6-ferrocenylpyrimidine-5-carbonitrile (10c): yield 1.46 g (42%), red crystals, mp 156-157 °C. IR 483, 818, 1000, 1104, 1557, 1612, 1633, 2160, 2927, 3094, 3322, 3467 cm-1; 1H NMR δ 3.21 (bs, 6H), 4.17 (s, 5H), 4.46 (m, 2H), 5.22 (m, 2H), 5.25 (m, 2H); 13C NMR δ 36.95, 70.29, 69.65, 71.02, 80.31, 119.44, 164.81, 170.06, 178.67, 189.72; MS: m/z 347 [M]+. Anal. Calcd For C17H17FeN5: C, 58.81; H, 4.93; N, 20.16. Found: C, 58.75; H, 4.81; N, 20.23.
6-Amino-2-ethoxy-4-ferrocenyl-5-ferrocenylmethyl-4,5-dihydropyridine-3,5-dicarbonitrile (11a): yield 0.19g (14%, from 8a), 0.16g (12%, from 8b), 0.2 g (15%, from 8c), yellow crystals, mp dec. ca. 273 °C. IR 482, 1001, 1106, 1553, 1595, 1638, 1665, 2194, 2245, 3098, 3325 cm-1; 1H NMR δ 1.40 (t, J = 6.9 Hz, 3H), 2.92 (d, J = 14.1 Hz, 1H), 3.11 (d, J = 14.1 Hz, 1H), 3.43 (s, 1H), 4.33 (q, J = 6.9 Hz, 2H), 4.15 (s, 5H), 4.28 (s, 5H), 3.87 (m, 1H), 4.09 (m, 1H), 4.13 (m, 1H), 4.17 (m, 2H), 4.24 (m, 2H), 4.43 (m, 1H), 5.56 (bs, 2H); 13C NMR δ 15.53, 37.01, 63.55, 41.42, 69.09, 69.60, 66.98, 68.24, 68.60, 68.91, 68.95, 69.17, 69.87, 70.29, 79.33, 83.64, 119.22, 120.51, 51.30, 160.54, 164.30, 165.68, 165.98; MS: m/z 572 [M]+. Anal. Calcd for C30H28Fe2N4O: C, 62.96; H, 4.93; N, 9.79. Found: C, 62.97; H, 4.62; N, 9.55.
6-Amino-4-ferrocenyl-2-methyl-3,4-dihydropyrimidine-5-carbonitrile (12a): yield 1.02 g (32%), yellow crystals, mp 203-204 °C. IR 485, 822, 1000, 1100, 1494, 1568, 1595, 1655, 2167, 2930, 3323, 3377 cm-1; 1H NMR δ 1.75 (s, 3H), 3.92 (m, 1H), 3.97 (m, 3H), 4.04 (s, 5H), 4.71 (d, J = 3.0 Hz, 1H), 5.55 (bs, 1H), 5.64 (bs, 2H); 13C NMR δ 22.01, 52.87, 69.12, 65.74, 65.84, 67.51, 67.99, 95.29, 122.99, 148,94, 159.50, 160.14; MS: m/z 320 [M]+. Anal. Calcd for C16H16FeN4: C, 60.02; H, 5.04; N, 17.50. Found: C, 59.91; H, 4.89; N 17.51.
6-Amino-4-ferrocenyl-2-phenyl-3,4-dihydropyrimidine-5-carbonitrile (12b): yield 1.26 g (33%), yellow crystals, mp 218-219 °C. IR 499, 812, 998, 1105, 1573, 1596, 1635, 2168, 3073, 3135, 3255 cm-1; 1H NMR δ 4.13 (m, 3H), 4.19 (m, 1H), 4.21 (s, 5H), 4.97 (d, J = 2.8 Hz, 1H), 5.74 (bs, 1H), 6.01 (bs, 2H), 7.49-7.55 (m, 3H), 7.87 (d, J = 6.0 Hz, 2H); 13C NMR δ 54.14, 69.17, 65.73, 65.95, 67.62, 68.24, 95.25, 122.82, 127.88, 128.87, 131.99, 133.93, 157.57, 159.9, 162.41; MS: m/z 382 [M]+. Anal. Calcd. for C21H18FeN4: C, 66.00; H, 4.74; N, 14.65. Found: C, 65.97; H, 4.58; N, 14.78.
Tipical procedure of the cyclodimerization of 2-cyano-3-ferrocenylacrylonitrile 7 in the presence 2-PrOH, H2O and Na2CO3. A mixture of compound 7 (1.13 g,5 mmol), 2-PrOH (60 mL), H2O (10 mL) and 1.0 g Na2CO3 was stirred for 12 h at 80 οC. The reaction mixture was worked up as described above, subsequent chromatography on Al2O3 gave 6-amino-4-ferrocenyl-5-ferrocenylmethyl-2-isoprpoxy-4,5- dihydropyridine-3,5-dicarbonitrile 11b, yield 0.4g (27%), yellow crystals, mp ca. 302 °C (decomp). IR 483, 821, 1001, 1106, 1294, 1316, 1355, 1423, 1585, 1629, 2191, 2230, 3095, 3241, 3335 cm-1; 1H NMR δ 1.39 (d, J = 6.0 Hz, 6H), 2.90 (d, J = 13.8 Hz, 1H), 3.09 (d, J = 13.8 Hz, 1H), 3.81 (s, 1H), 4.13 (s, 5H), 4.28 (s, 5H), 4.08 (m, 1H), 4.16 (m, 2H), 4.23 (m, 2H), 4.28 (m, 2H), 4.42 (m, 1H), 5.07 (m, J = 6.0 Hz, 1H), 5.54 (bs, 2H); 13C NMR δ 21.94, 36.96, 41.40, 66.96, 69.04, 69.56, 68.20, 68.54, 68.90, 68.94, 69.09, 69.84, 70.38, 71.52, 79.25, 83.68, 119.16, 120.49, 51.28, 160.43, 161.54, 165.37, 166.82; MS: m/z 586 [M]+. Anal. Calcd for C31H30Fe2N4O: C, 63.51; H, 5.16; N, 9.55. Found: C, 63.67; H, 5.03; N 9.41.
ACKNOWLEDGEMENTS
This work was supported by CONACyT (Mexico, grant 100970) and DGAPA-UNAM (Mexico, grant IN-211112).
References
1. G. Salem and C. L. Raston, ´The Use of Organometallic Compounds in Organic Synthesis´, Vol. 4, ed. by F. R. Hartley, Wiley: Chicheester, 1987, 159.
2. P. Kopf-Maier and H. Kopf, Chem. Rev., 1987, 87, 1137. CrossRef
3. (a) N. Metzler-Nolte, Angew. Chem. Int. Ed., 2001, 40, 1040; CrossRef U. Schatzschneider and N. Metzler-Nolte, Angew. Chem. Int. Ed., 2006, 45, 1504; CrossRef (b) D. R. van Staveren and N. Metzler-Nolte, Chem. Rev., 2004, 104, 5931; CrossRef (c) G. Gasser, I. Ott and N. Metzler-Nolte, J. Med. Chem., 2011, 54, 3. CrossRef
4. A. E. Kaifer and J. de Mendoza Ch, Comprehensive Supramolecular Chemistry, Vol. 1, (Elsevier, Oxford), 1996, 701.
5. T. Yao and G. A. Rechnitz, Biosensors, 1987, 3, 307. CrossRef
6. P. Stepnicka, Ferrocenes: ligands, materials and biomolecules, Wiley, Chichester, 2008.
7. C. G. Hartinger and P. J. Dyeon, Chem. Soc. Rev., 2009, 38, 391. CrossRef
8. H. Parveen, F. Hayat, A. Salahuddin, and A. Azam, Eur. J. Med. Chem., 2010, 45, 3497. CrossRef
9. E. Hillard, A. Vessières, L. Thouin, G. Jaouen, and C. Amatore, Angew. Chem. Int. Ed., 2006, 45, 285; CrossRef M.-G. Schvekhgeimer, Russ. Chem. Rev., 1996, 65, 43.
10. M. S. K. Youssef, Rev. Roum. Chim., 1981, 26, 1005.
11. E. Klimova, T. Klimova, T. Ramírez Apan, A. Nieto Camacho, R. Moreno Esparza, C. Damian Zea, and M. Martínez García, Heterocycles, 2004, 63, 1045. CrossRef
12. E. A. Vázquez López, E. Klimova, T. Klimova, C. Alvarez Toledano, L. Ruíz Ramírez, R. A. Toscano, and M. Martínez García, Synthesis, 2004, 2471. CrossRef
13. E. I. Klimova, E. A. Vázquez López, T. Klimova, C. Alvarez Toledano, R. A. Toscano, and M. Martínez García, J. Heterocycl. Chem., 2005, 42, 265. CrossRef
14. S. Toma, M. Putala, and M. Salisava, Collect. Czech. Chem. Commun., 1987, 52, 395. CrossRef
15. V. N. Postnov, A. V. Goncharov, I. Hocke, and D. P. Krut’ko, J. Organomet. Chem., 1993, 456, 235. CrossRef
16. G. G. Abashev, A. D Antuf’eva, A. Yu. Bushueva, P. G. Kudryavtsev, I. V. Osorgina, R. V. Syutkin, and E. V. Shklyaeva, Russ. Appl. Chem., 2010, 83, 1330; CrossRef A. I. Moskalenko, A. V. Boeva, and V. I. Boev, Russ. J. Gen. Chem., 2011, 81, 521. CrossRef
17. C. Fehér, Á. Kuik, L. Márk, L. Kollár, and R. Skoda-Földes, J. Organomet. Chem., 2009, 694, 4036. CrossRef
18. G. S. Rashinkar, S. B. Pore, K. B. Mote, and R. S. Salunkhe, Indian J. Chem., 2009, 48B, 606.
19. W.-J. Zhou, S.-J. Ji, and Z.-L. Shen, J. Organomet. Chem., 2006, 691, 1356. CrossRef
20. E. Stankovic, P. Elecko, and S. Toma, Chem. Pap., 1996, 50, 68.
21. Crystallographic data for the structure of compounds 9 (CCDC-872988), 11b (CCDC-873043) and 12b (CCDC–873044) reported in this paper have been deposited with the Cambridge Crystallographic Data Centre, 12, Union Road, Cambridge DB2 1EZ, UK; Fax: (internat.) +44-1223/336-033; E-mail: deposit@ccdc.cam.ac.uk. These data can be obtained free of charge via www.ccdc.com uk/data_request/cif.
22. D. J. Brown, in: The Pyrimidines, John Wiley& Sons: New York, 1994.
23. F. H. Allen, O. Kennard, D. G. Watson, L. Brammer, A. Guy Orpen, and R. Taylor, J. Chem. Soc., Perkin Trans. 2, 1987, S1. CrossRef
24. V. N. Postnov, E. I. Klimova, A. N. Pushin, and N. N. Meleshonkova, Metalloorg. Khim. Chem., 1992, 5, 564.
25. G. M. Sheldrick, SHELXS-97, Program for the Refinement of Crystal Structures; University of Göttingen: Göttingen, Germany, 1994.