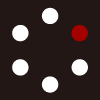
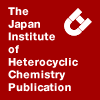
HETEROCYCLES
An International Journal for Reviews and Communications in Heterocyclic ChemistryWeb Edition ISSN: 1881-0942
Published online by The Japan Institute of Heterocyclic Chemistry
e-Journal
Full Text HTML
Received, 26th July, 2012, Accepted, 29th August, 2012, Published online, 6th September, 2012.
DOI: 10.3987/COM-12-12556
■ RUTHENIUM PORPHYRIN CATALYZED FRIEDEL–CRAFTS TYPE REACTION OF ARENES WITH IMINES
Takuma Terada, Takuya Kurahashi,* and Seijiro Matsubara*
Department of Material Chemistry, Graduate School of Engineering, Kyoto University, Kyoudai-katsura, Nishikyo, Kyoto 615-8510, Japan
Abstract
Cationic ruthenium porphyrin complex was found to be an efficient catalyst for the Friedel–Crafts type reaction of arenes with imines. The use of a structurally rigid tetradentate porphyrin as the equatorial ligand and weakly coordinating axial ligand is the key to bring out the catalytic reactivity of ruthenium for the reaction.In the past few decades, many transition metal complexes have emerged as powerful catalyst for the Friedel-Crafts type reaction. The transformation is considered to be triggered by activation of the electrophiles through coordination to electrophilic metal complex to provide key intermediates such as alkyl cations and acyl cations.1,2 Thus, taking into account the Lewis acidity of the metal complexes, we supposed that higher-valent complexes that do not oxidize the substrate are favorable catalysts for the Friedel-Crafts type reaction. In this context, we presumed that a cationic high-valent metalloporphyrin would be an ideal catalyst because the structurally rigid tetradentate porphyrin ligand with a large π-conjugated planar aromatic structure can help in maintaining the high oxidation state of the metal throughout the catalytic process.3 Herein, we report that the cationic ruthenium porphyrin complex catalyzes the Friedel-Crafts type reaction of arenes with imines.4
Our investigation began with attempted Friedel-Crafts type reaction of trimethoxybenzene (1a) with aldimine (2a) in the presence of 1 mol % of cationic ruthenium porphyrin catalyst [Ru(TBPP)](SbF6)2 in MeCN at ambient temperature for 1 h. This resulted in formation of 3aa in 97% yield (Table 1, entry 1). Of note, non-ruthenium cationic metalloporphyrin complexes such as [Mn(TPP)]SbF6, [Fe(TPP)]SbF6, and [Co(TPP)]SbF6 did not show any catalytic activity (entries 2 and 4). We also examined the effect of various counteranions such as BF4– and Cl–, which would act as axial ligands, on the cationic nature of ruthenium (entries 5–6).5 The desired product 3aa was not obtained when using other ruthenium catalysts such as [Ru(TBPP)]Cl2 and [Ru(TBPP)](BF4)2. Among various reaction solvents examined, toluene gave the best yield of the product (entries 7–9). It was found that the use of mono cationic ruthenium complex [RuCl(TPPB)](SbF6) as a catalyst resulted in formation of 3aa in slightly lower yield than that of [Ru(TPPB)](SbF6)2 (entry 10).
With the optimized conditions in hand, we next investigated the scope of the reaction using various imines and the results are summarized in Table 2. Imines 2 possessing electron-withdrawing and –donating substituents on the phenyl group also reacted with 1a to afford the correspondingly substituted 3 in excellent yields. It is worth to note that acetoxy, cyano, and nitro substituents were well tolerated under the present reaction conditions and the corresponding adducts were isolated in excellent yields. Naphthyl aldimines reacted with 1a in the presence of the ruthenium catalyst to furnish the correspondingly substituted products 3ag and 3ah respectively. Notably, aliphatic aldimine 2i also reacted with 1a to afford 3ai in 96% isolated yield. Imine 2 bearing a nosyl (Ns: 2-nitrobenzenesulfonyl) substituent, which is more easily removable than the tosyl group, reacted with 1a to yield 3aj in 67% yield. Furthermore, the reaction of imine possessing Boc and Cbz protection group with 1a provided 3ak and 3al in 90% and 71% isolated yields, respectively.
During the course of our study, we found that the reaction of imine 2a with less stericaly hindered arene such as 1b in the presence of [Ru(TBPP)](SbF6)2 catalyst furnish 4ba in 59% yield via double arylation and elimination of tosyl amide (Table 3). Further examination of reaction condition revealed that the use of [RuCl(TBPP)](SbF6) in place of [Ru(TBPP)](SbF6)2 gave better result and 4ba was obtained in 69% yield. Imines 2 possessing electron-withdrawing and –donating substituents on the phenyl group also reacted with 1b to afford the correspondingly substituted triarylmethanes 4 in excellent yields. Naphthyl aldimines 2g and 2h reacted with 1b to furnish 4bg and 4bh in 47% and 49% isolated yields, respectively. Aliphatic aldimine 2i also reacted with 1b to afford 4bi in 44% yield. It was also found that the [RuCl(TBPP)](SbF6) catalyzed reaction of 2-methylfuran (1c) in place of 1b with 2a also afforded 5ca in 77% isolated yield via elimination of tosyl amide (Scheme 1). However, the reaction of less electron-rich arenes such as toluene and anisole did not afford any products.
n conclusion, we demonstrated the catalytic efficiency of the ruthenium-porphyrin complexes [Ru(TBPP)](SbF6)2 and [RuCl(TBPP)](SbF6) for the Friedel–Crafts type reaction of imines with arenes. The high activation ability of the catalyst that competes with the high chemoselectivity could be attributed to the cationic ruthenium, which in turn was due to the rigid tetradentate planar porphyrin ligand and the weakly coordinating axial SbF6 ligand. Efforts toward elucidation of the unique reactivity of the cationic ruthenium-porphyrin complex are underway.
ACKNOWLEDGEMENTS
This work was supported by Grants-in-Aid from the Ministry of Education, Culture, Sports, Science, and Technology, Japan. T. K. acknowledges Asahi Glass Foundation, Tokuyama Science Foundation, Kurata Memorial Hitachi Science and Technology Foundation.
References
1. C. Friedel and J.-M. Crafts, R. Hebd. Seances Acad. Sci., 1877, 84, 1392; M. Bandini, A. Melloni, and A. Umani-Ronchi, Angew. Chem. Int. Ed., 2004, 43, 550. CrossRef
2. A. Ishii, V. A. Soloshonok, and K. Mikami, J. Org. Chem., 2000, 65, 1597; CrossRef N. Gathergood, W. Zhuang, and K. A. Jørgensen, J. Am. Chem. Soc., 2000, 122, 12517; CrossRef W. Zhuang, N. Gathergood, R. G. Hazell, and K. A. Jørgensen, J. Org. Chem., 2001, 66, 1009; CrossRef M. P. A. Lyle, N. D. Draper, and P. D. Wilson, Org. Lett., 2005, 7, 901; CrossRef P. Thirupathi and S. S. Kim, J. Org. Chem., 2010, 75, 5240. CrossRef
3. For some examples of the use of metalloporphyrins in non-oxidative bond formation, see: K. Suda, K. Baba, S.-I. Nakajima, and T. Takanami, Chem. Commun., 2002, 2570; CrossRef K. Suda, T. Kikkawa, S.-I. Nakajima, and T. Takanami, J. Am. Chem. Soc., 2004, 126, 9554; CrossRef J. Chen and C.-M. Che, Angew. Chem. Int. Ed., 2004, 43, 4950; CrossRef J. A. R. Schmidt, E. B. Lobkovsky, and G. W. Coates, J. Am. Chem. Soc., 2005, 127, 11426. CrossRef
4. R. C. Young, J. K. Nagle, T. J. Meyer, and D. G. Whitten, J. Am. Chem. Soc., 1978, 100, 4773; CrossRef W.-H. Leung, T. S. M. Hun, H.-w. Hou, and K.-Y. Wu, J. Chem. Soc., Dalton Trans., 1997, 237. CrossRef
5. W. Beck and K. Sünkel, Chem. Rev., 1988, 88, 1405. CrossRef